Connective tissue
Publisher Summary
Living organisms are not merely random collections of cells; each organism grows according to a detailed pattern that determines both the structure and the functions of its many constituent parts, and ensures that they are all integrated with one another both anatomically and biochemically. One of the basic requirements for systematic arrangement within tissues is a mechanical one—a means of maintaining the cells in appropriate relationship to each other despite the various forces that act upon them, including gravity, externally applied physical stress, and the internal movements of the organism itself, which result from growth and exertion. Two contrasting systems for organizing their mechanical structures are employed by multicellular organisms. These systems differ in whether the mechanical elements that determine the rigidity of the structure are closely associated with cells or frankly extracellular. This chapter describes the characteristics and functions of the connective tissue. They fulfill many functions but their primary function is a mechanical one, connective tissue elements being responsible for maintaining cells, tissues, and organs in proper relationship to one another. They also provide the animal with support, usually by means of a rigid skeleton, to which the softer tissues are attached, and with a system for the transmission of mechanical force, so that the contractile power of the muscles can be harnessed to the skeletal framework and used to move the animal as a coordinated whole.
Two contrasting systems for organizing their mechanical structures are employed by multicellular organisms. These systems differ in whether the mechanical elements that determine the rigidity of the structure are closely associated with cells or frankly extracellular. A good example of a mechanical system of the former type occurs in higher plants where the cells secrete a strong thick layer around themselves, the cell wall, which resists the internal pressure exerted against it by the cell itself. As a result, the individual cells are rigid like inflated motor tyres and thus form the basis of a rigid multicellular structure. The main building material of plant cell walls is cellulose, a homopolymer composed of bundles of chains each of which contains about 5000 glucose units. Another kind of cell-based mechanical organization occurs in the keratinous epithelia of higher animals (Chapter 26).
Occurrence and characteristics of connective tissues
Connective tissues fulfil many functions but their primary function is a mechanical one, connective tissue elements being responsible for maintaining cells, tissues and organs in proper relationship to one another. They also provide the animal firstly with support, usually by means of a rigid skeleton, to which the softer tissues are attached and, secondly, with a system for the transmission of mechanical force, so that the contractile power of the muscles can be harnessed to the skeletal framework and used to move the animal as a coordinated whole. Connective tissues are essentially mesodermal in origin being derived from the primitive mesenchyme, a layer of the early embryo. They all contain the fibrous protein collagen, as their most important and characteristic constituent. Typical forms are tendon, the corium layer of the skin, loose connective tissue, cartilage and the basement membranes of various tissues. In a mineralized form connective tissue is present in calcified cartilage, bone, dentine and cementum, which are described in Chapter 29. Connective tissues thus include many of the tissues of specific dental interest such as the oral subepithelium (corresponding to the corium of skin), the periodontal membrane or periodontal ligament, the alveolar bone of the tooth socket, cementum, dentine and the dental pulp.
The main components of connective tissues are shown in Table 27.1. The fibroblasts are responsible for the synthesis of the remaining non-living extracellular components, and, when actively laying down collagen fibres in soft connective tissues, are typically amoeboid cells which protrude spiky processes. They may become differentiated as in bone, where the osteocytes become embedded throughout the mineralized tissue, and in dentine, where odontoblasts remain near the boundary between the mineralized dentine and the pulp with their processes passing through the thickness of the dentine. The connective tissue cells first lay down the ground substance as a gel containing various kinds of macromolecule. This contains a relatively large volume of water and provides a suitable environment for the subsequent deposition of the fibrous proteins. These are secreted by the fibroblasts as macromolecules which are further assembled extracellularly, and eventually give rise to large insoluble fibrous aggregates, which, as the connective tissue matures, fill most of the extracellular space and give the tissue great mechanical strength.
Table 27.1
Components of connective tissues
Cells | Fibroblasts in more or less differentiated forms, e.g. chondroblasts, odontoblasts, osteocytes, osteoblasts, etc. |
Fat cells, macrophages, plasma cells, mast cells and leucocytes | |
Interfibrillar matrix or ground substances (molecules, often high polymers) | Proteoglycans (glycosaminoglycans) |
Glycoproteins | |
Phospholipids | |
Water | |
Protein fibres | Collagen |
Reticulin | |
Elastin |
The ground substance
Proteoglycans
With the exception of keratan sulphate, which contains galactose residues in place of uronic acid, the composition of connective tissue glycosaminoglycans shows variations on the theme described in the previous paragraph (Table 27.2). Hyaluronic acid is only weakly acidic, whereas the chondroitin sulphates have strongly acidic sulphate groups.
Table 27.2
Features of the composition of glycosaminoglycans from connective tissues
Polysaccharide | Hexosamine | Uronic acid (or hexose) |
Hyaluronic acid | N-Acetyl-D-glucosamine (1:3 linkage, SO4 absent) | D-Glucuronic acid (1:4 linkage) |
Chondroitin 4-sulphate | N-Acetyl-D-galactosamine (1:3 linkage, 4-SO4) | D-Glucuronic acid (1:4 linkage) |
Dermatan sulphate | N-Acetyl-D-galactosamine (1:3 linkage, 4-SO4) | L-Iduronic acid (1:4 linkage) |
Chondroitin 6-sulphate | N-Acetyl-D-galactosamine (1:3 linkage, 6-SO4) | D-Glucuronic acid (1:4 linkage) |
Chondroitin | N-Acetyl-D-galactosamine (1:3 linkage, SO4 absent) | D-Glucuronic acid (1:4 linkage) |
Heparan sulphate | N-Acetyl-D-glucosamine (partially sulphated) | D-Glucuronic acid |
Keratan sulphate | N-Acetyl-D-glucosamine (1:4 linkage, 6-SO4) | D-Galactose (1:3 linkage) |
Glycosaminoglycans are nearly always associated with a smaller amount of protein. At one time this was thought to be a loose electrostatic association, but recently it has been shown that the polysaccharide is usually covalently linked through an alkali-labile O-glycosidic bond to a serine group on the protein. This gives a very large molecule, with a single protein chain, the core protein, to which many long carbohydrate chains are covalently attached (Figure 27.1).
Glycoproteins
Glycoproteins are conjugated proteins having one or more short irregular heterosaccharide side chains bound covalently to the polypeptide chain. In some respects therefore they resemble proteoglycans of the chondroitin sulphate type but differ from them in the shortness of their polysaccharide chains (2–20 units long) which do not contain a regularly repeating disaccharide unit. The carbohydrate content is usually lower than in proteoglycans. Glycoproteins vary enormously in the number, composition and size of their carbohydrate side chains. Like glycosaminoglycans, they possess acetylhexosamines but uronic acids are not present in glycoproteins. Mannose and galactose and the methyl pentose, fucose (6-deoxy-L-galactose), occur commonly, but the most characteristic constituent is N-acetylneuraminic acid (sialic acid) which often terminates the free ends of the carbohydrate chains. Sialic acid is both a sugar and an amino acid and its strongly acid carboxyl group (pK 2·6) gives it acidic properties throughout a wide range of pH, which it confers on the glycoprotein as a whole. Glycoproteins which contain sialic acids are known as sialoproteins. The polar nature of sialic acid makes this group of proteins strongly hydrophilic, stretching out the side chains in an aqueous environment. Glycoproteins are present in saliva and are responsible for its surface active and ‘spinnbarkeit’ properties. They probably occur in the ground substance of many connective tissues but in rather small proportions. The best characterized connective tissue glycoprotein is bone sialoprotein (page 439).
The glycoproteins, as mentioned in Chapter 5, are a most interesting and varied group of proteins and are of special importance in oral biology as constituents of both saliva and the capsules of many bacteria. The rigid wall of these bacteria is composed of the glycopeptide murein, which is elaborately cross-linked so that it forms a single bag-shaped molecule. This is responsible for the shape and mechanical strength of the cell wall (page 205) and its importance is illustrated by the lethal effects of penicillin, which interferes with its synthesis (page 480). Its carbohydrate component is susceptible to the antibacterial enzyme lysozyme.
Functions of the ground substance
The higher water content of young tissues provides a clue to one of the most important properties of proteoglycans, which enables them to form the intial extracellular milieu for fibre deposition. Their molecules being polyanionic spread out in net-like form, each strand being associated with a comparatively large quantity of water. This is hydrogen bonded to the hydrophilic hydroxyl groups and loosely held by ‘solvation’ of the fixed ionized groups on the proteoglycan chains (see Chapter 3). Because they have very open structures the proteoglycans will hold an enormous weight of water per unit weight of organic material and, although soft tissues contain up to 70% of water, no fluid seeps out when they are punctured.
The small molecules of nutrients and waste products can diffuse freely, through the water held by proteoglycans, but diffusion of macromolecules, such as tropocollagen (page 44), is restricted to those regions that are fairly remote from the individual strands of this hydrated net. Such molecules are therefore channelled through the free water, either within the large holes in the network or between the larger sheets of netting. Presumably the restriction to free diffusion resulting from the size and spatial distribution of these holes affects the distribution and orientation of newly laid down collagen fibres.
At present it is not possible to account for the specific behaviour of each of the various proteoglycans occurring in tissues. The kinds and proportions of different mucosubstances vary greatly between tissues and even change in the same tissue as it ages. Thus cartilage is rich in chondroitin 4-sulphate and dermatan sulphate, whereas chondroitin 6-sulphate and keratan sulphate are dominant in the cornea. Skin contains hyaluronic acid, dermatan sulphate and chondroitin 6-sulphate, which predominate in turn as the embryonic tissue grows older. As rib cartilage ages, the chondroitin sulphate is replaced by keratan sulphate. The proportion of fibrous protein to proteoglycan also varies in different tissues and, as already mentioned, increases with age. Since collagen is the principal fibrous protein and characteristically contains a high proportion of hydroxyproline (page 35), the hydroxyproline/hexosamine ratio is a convenient index of the extent to which the ground substance has been impregnated with fibres. Values for this index vary considerably, e.g. 2·8 for cartilage, 12·2 for skin and 30 for tendon.
Some disorders of proteoglycan metabolism, e.g. Hurler’s syndrome, have been shown to be enzyme-deficiency diseases in which a lysosomal enzyme is missing (page 203).
The protein fibres
Three main kinds of fibrous protein are found in connective tissues, collagen, reticulin and elastin. Collagen is the most abundant protein of mammals and accounts for 25–30% of their total protein content. Reticulin, although a histologically defined entity, is built up from collagen units, together with carbohydrate and possibly lipid, as described in Chapter 26
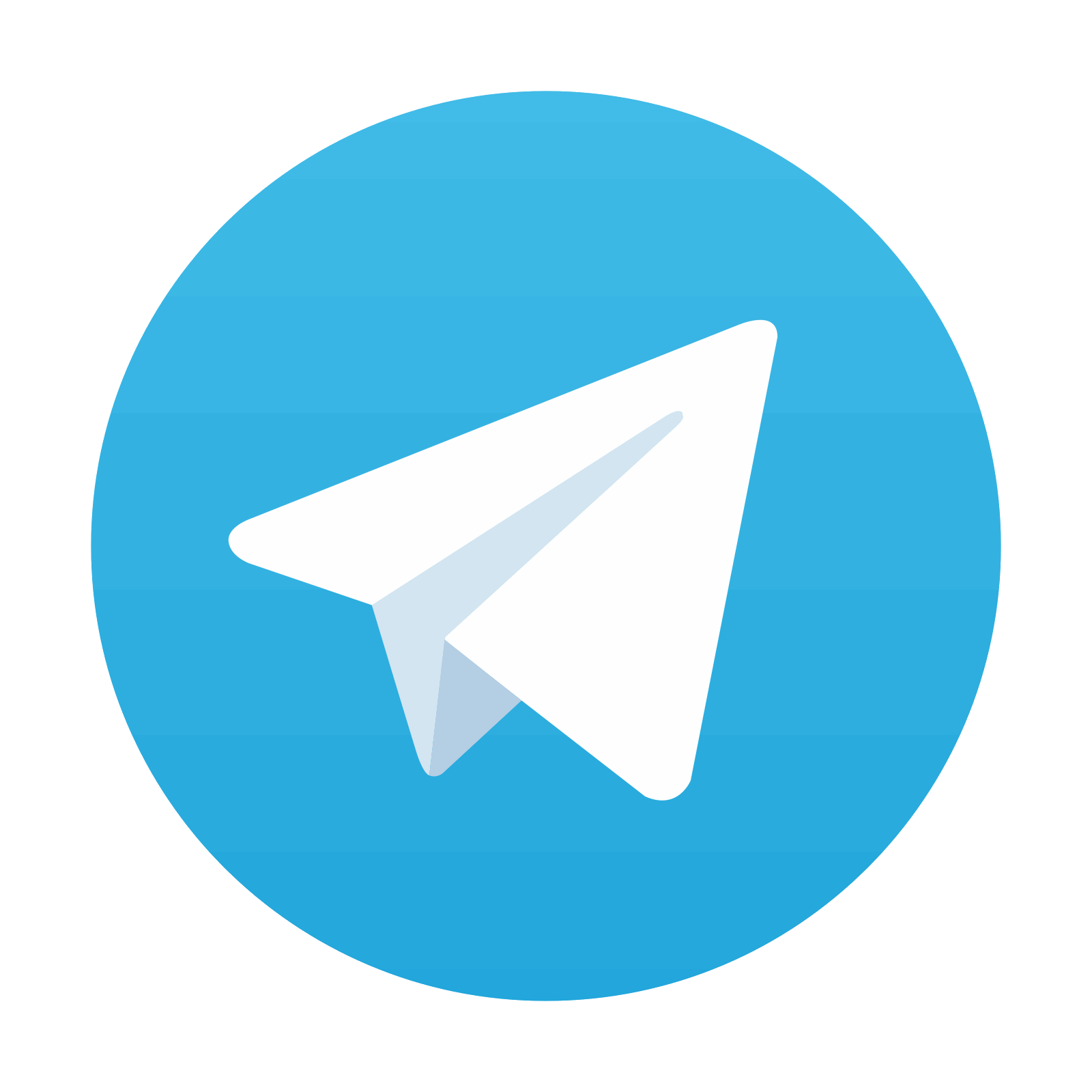
Stay updated, free dental videos. Join our Telegram channel

VIDEdental - Online dental courses
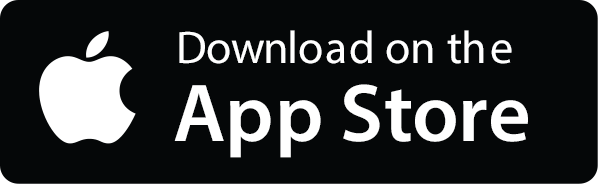
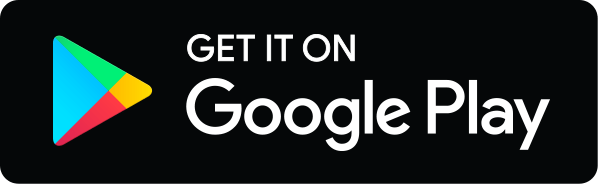