Key points
- •
Rigid fixation for maxillofacial fractures has been in use for over the last 40 years.
- •
The concept of rigid fixation uses hardware in the form of bone plates and screws to absorb part or all of the functional load of the fracture site preventing any motion across the fracture.
- •
Many different systems and materials have been introduced over the years and have evolved to the current systems we use today.
- •
The use of titanium plates and screws in maxillofacial fractures is now considered the standard of care in the repair of maxillofacial fractures.
Introduction
Rigid fixation for maxillofacial fractures has been in use for over the last 40 years. The concept of rigid fixation uses hardware in the form of bone plates and screws to absorb part or all of the functional load of the fracture site preventing any motion to the fracture. Rigid fixation first originated in the orthopedic literature in 1932 by Key and then in 1949 by Luhr whom described compression of bony fragments as an adjunct to fracture healing. Subsequently in 1958, Bagby and Janes published an article on the use of bone plates to achieve immobilization of the fracture and active compression. The early articles on rigid fixation for maxillofacial fractures had mixed results, as the appliances used were designed for extremities fractures. It was not until 1973 when Michelet described the use of miniaturized screws and plates in the reduction and immobilization of fractures in detail with the experience of 300 cases. Since then, many different systems and materials have been introduced and have evolved to the current systems we have today. The use of these miniaturized plates and screws in maxillofacial fractures is now considered the standard of care in the repair of maxillofacial fractures ( Fig. 1 ).

Pathways of maxillofacial skeletal fracture healing
Fracture healing involves a complex set of events to restore the bone to its preinjured form. The mechanical stability of a fracture site determines the type of healing. Bone healing, like soft tissue healing, can occur via a secondary or primary route. Secondary bone healing occurs when there is increased mechanical strain resulting in the ability of the fractured ends to move during the healing process or when precise anatomic reduction is not achieved. Movement of the fracture segments result in a large amount of external and internal callus, which is the body’s way to immobilize the fracture. Secondary bone healing consists of 3 main stages: inflammation, repair, and remodeling. During the inflammatory phase, a hematoma is formed and provides a source of hematopoietic cells capable of secreting growth factors. Fibroblast and mesenchymal cells then migrate to the fracture site allowing granulation tissue to form around the fractured ends after which osteoblasts and fibroblasts start to proliferate. In the repair phase, a primary callus will start to form normally within 2 weeks, and when the bone ends do not meet, a bridging callus forms. The size of the callus is inverse to the extent of immobilization and the distance between the fractured ends. The remodeling phase begins in the middle of the repair phase continuing long after clinical union, allowing the remodeling woven bone produced in the repair phase to become lamellar bone.
Primary bone healing occurs when there is minimal strain and good anatomic reduction such as when rigid fixation is performed. Primary bone healing is predicated on the direct restoration of lamellar bone. The callus formed when good anatomic reduction is achieved is minimal compared with secondary bone healing. Medullary bone portion when placed into apposition will allow osteogenic elements and capillary blood supply to traverse the fracture line and result in longitudinal bone healing with remodeling in about 3 to 4 weeks. Repair of the cortical bone occurs by growth of capillaries and osteogenic elements across the fracture lines by cortical tunnels, also known as contact healing. Cortical bone completely heals after fracture in approximately 16 weeks.
Compression and tension forces
Frederic Pauwels described the early concepts of load transfer within bone. He observed that a curved tubular structure under axial load always has a compression side as well as a tension side. In fracture healing, there is general agreement that compression have been shown to promote rapid healing and a greater resistance to separation when a fracture is placed under compression. Compression does not necessarily promote osteogenesis, but it provides the intimate apposition and mechanical stability allowing the fractured bone to heal primarily.
At any time, to be in equilibrium, the bone will be under both compressive and tensile forces, depending on the mechanics of the articulation of the bone and the attached muscular attachments. Rigid fixation should address both the tension and compression areas of a fracture. When the tension areas of a bone are addressed, it eliminates overcompressive forces, allowing equal distribution of forces over the fracture areas, which allows the bone to heal optimally. When one looks at mandible fractures, compressive and tensile forces are different depending on location. In fractures distal to the canine region, the alveolar region is predominantly under tension when the inferior border is under compression. But when looking in the area anterior to the canine region, the forces are mixed with the alveolar and inferior border of the mandible having almost equal tensile and compressive forces during function ( Fig. 2 ).

Load-bearing versus load-sharing concepts in rigid fixation
Currently when rigid fixation is being considered for fractures, there are 2 basic types of fracture fixation techniques: load-bearing osteosynthesis and load-sharing osteosynthesis. In load-bearing osteosynthesis, the plate bears all the forces of function at the fracture site; this is accomplished with a locking reconstruction plate ( Figs. 3 and 4 ). In load-sharing osteosynthesis, stability at the fracture site is through frictional resistance between the fractured bone ends and the hardware used to hold the ends together. In the ideal load-sharing scenario, the bone bears most of the functional loads. Load-sharing osteosynthesis includes lag screw fixation, compression plating, or miniplate fixation. In the lag screw osteosynthesis technique, the screw threads engage the cortical bone across the fracture line from the site of screw insertion. The site of insertion of the screw is overdrilled, and when the screw is tightened, the fractured segments are placed under compression. Insertion of at least 2 screws is recommended to eliminate the possibility of rotational movements ( Fig. 5 ).

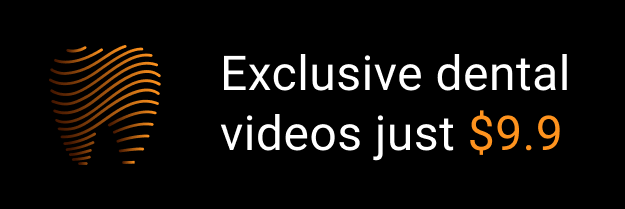