9
Computed Tomography Imaging Devices and Techniques
Marco A. Versiani and Gianluca Gambarini
Summary
Since the early part of the 21st century, technological advancements in three-dimensional computed tomographic imaging have given rise to more accurate methods for clinical and laboratory approaches in the field of endodontics. Cone beam computed tomography (CBCT) and high-resolution micro-computed tomography (micro-CT) have gained increasing significance in dental research and clinical applications, particularly because they are nondestructive and reproducible techniques. Use of CBCT and micro-CT in experimental and clinical endodontics is now widespread and is also becoming integrated into dental education. The purpose of this chapter is to discuss the technical aspects and potential applications of these technologies in clinical and education endodontic training.
9.1 Digital Dentistry and Impact on Clinical Training and Education
CBCT is one of the most significant advances in endodontics since 2010. When used appropriately, it can greatly enhance the diagnosis and treatment planning of a variety of cases [1, 2]. However, it is important that each case is evaluated individually to ensure that the potential risk of additional radiation is justified with the benefits provided from its indication. In the latest position statement of the European Society of Endodontology (ESE) on the use of CBCT in endodontics [3], a wide range of scenarios in which it can be used to improve performance or facilitate the endodontic treatment are listed; in addition, the potential employment of CBCT-based endodontic software was also mentioned.
9.1.1 3D Endodontic Rendering
3D rendering is a computer graphic process that converts 3D models into 2D images on a computer (Figure 9.1). Three-dimensional rendering can be used to visualise in an easier way tooth anatomy and aspects of endodontic treatment, the lesions, and the surrounding tissue. It is helpful for both dentists and patients and consequently is a great tool for communication with patients (Figure 9.2) in order to visualise preoperative, postoperative, and follow-up scans and to explain the clinical conditions, healing, and so forth. The rendering can be visualised on the computer screen, immediately and without adding costs, or can be 3D printed, which is a more complex, time consuming, and costly procedure. To visualise the various tissues and structures on computer images, a special process is needed: image segmentation, which is the process of partitioning a digital image into multiple segments (sets of pixels, also known as image objects). The goal of segmentation is to simplify and/or change the representation of an image into something that is more meaningful and easier to analyse [2, 4]. Image segmentation is typically used to locate objects and boundaries (e.g. lines, curves) in images. More precisely, image segmentation is the process of assigning a label to every pixel in an image such that pixels with the same label share certain characteristics. The simplest method of image segmentation is called thresholding. This method is based on a clip-level (or a threshold value) to turn a grey-scale image into a binary image. The key to this method is to select the threshold value (or values, when multiple levels are selected). Several popular methods are used in industry including the maximum entropy method, balanced histogram thresholding, Otsu’s method (maximum variance), and k-means clustering. Recently, other methods have been developed for thresholding computed tomographic (CT) images.

Figure 9.1 3D rendering creates 3D images or video by combining images from multiple plans.

Figure 9.2 CBCT images from multiple molar case plans and 3D rendering (pink).
9.1.2 3D Endodontic Software
Radiographic examination is essential in the diagnosis and management of pulp and periapical conditions and has been performed for many decades with two-dimensional (2D) periapical radiographic images. A single radiograph has limited diagnostic ability due to anatomical noise from superimposed structures, such as the maxillary sinus, zygomatic arch, and inferior alveolar nerve canal. Apical periodontitis is also difficult to diagnose in periapical radiographic images when the lesions are confined to cancellous bone, especially when covered by a thick cortical plate. Moreover, root canal anatomy and canal trajectories are also difficult to determine in a pre-operative 2D periapical radiographic image: the three-dimensional anatomy is compressed into a two-dimensional image, which will result in superimposition of the roots and canals and prevent full appreciation of the real anatomy [5]. An intraoperative radiographic view is therefore recommended for better visualisation of root canals and working length determination using hand instruments. A CBCT scan, on the contrary, could efficiently provide the information needed before using hand or automated instrumentation systems. It also allows a better assessment of complex canal morphology.
CBCT is the preferred imaging modality for complex situations demanding a more detailed localisation and description of tooth anatomy because of its capacity to render 3D information compared to conventional dental radiographs [6]. Even though the use of CBCT amongst endodontists has become more popular recently, the potential of 3D imaging in clinical endodontic practice is hampered by limitations such as the low resolution of many CBCT machines, the absence of small field-of-view (FOV) (less radiation) parameters, and the lack of analytical tools provided by computer software. Its clinical benefit is also influenced by the experience of the clinician during the interpretation and analysis of CBCT scans.
Recently, a new software developed for analysing endodontic parameters on CBCT images, the 3D EndoTM (Dentsply Sirona, Wals bei Salzburg, Austria), allows a more interactive and user-friendly interface for the identification and visualisation of root canals (Figure 9.3). In the past, the number and location of the canal orifices have been a major clinical problem in endodontics, which may cause treatment failure [7, 8], but the use of the dental operating microscope (and also CBCT) significantly reduced the risk of missed canals [9]. However, an operating microscope can only allow visualisation of the straight parts of the canal, and it is not helpful in understanding curvatures. CBCT can depict curvatures in three dimensions, but it requires skill and expertise from the clinicians to correctly visualise the entire curve. Faraj and Boutsioukis [5] reported that computer-based quantitative methods for the measurement of curvatures by endodontists were more reliable than visual estimation. Without dedicated software, it would be difficult even for an expert clinician to reassemble the various multiplanar CBCT images and precisely rebuild a mental image of canal curvatures.

Figure 9.3 CBCT images of a maxillary molar imported into the 3D Endo™ software.
3D EndoTM software has an intuitive interface in which the clinician can follow steps for the identification and measurement of canals, as well as virtual planning of the access cavity, apical limit, and instrument selection for canal shaping procedures (Figures 9.4–9.10). It has been reported that this software enhances the 3D visualisation of the canal complexities such as curvatures and confluences [1]. An innovative feature of this software is the semiautomated detection of the root canal trajectory; after identifying the orifice and apical foramen (Figure 9.6), an automated line connecting them is generated, which can be adjusted to follow the canal trajectory in three dimensions (Figure 9.7). Using this trajectory as a reference, a virtual endodontic instrument can be used to estimate the working length, using either an automatic suggestion or manual adjustment by the clinician (Figure 9.8). This semiautomated detection of the canal trajectory has the potential to minimise subjective errors related to the operator’s skills. Clinicians can visualise the straight-line access of the instrument towards the canal terminus and how wide the access cavity must be designed from the occlusal plane; they can also observe the effect of a straighter access on the cavity design (Figure 9.9). Additionally, this software can identify the canal curvature, measure root canal length, and also use a colour code for easier visualisation of anatomical details such as confluences and multiple curvatures in three dimensions (Figure 9.10).

Figure 9.4 3D Endo™ software selecting the tooth to analyse.

Figure 9.5 3D Endo™ software cropping the tooth to be analysed in order to reduce superimposition of other structures.

Figure 9.6 3D Endo™ software selecting the orifice and canal terminus for each canal (using different colours for each canal).

Figure 9.7 3D Endo™ software selecting the tooth to analyse.

Figure 9.8 3D Endo™ software automatic design trajectories, which can be manually adjusted.

Figure 9.9 3D Endo™ software digital insertion of a K-file to visualise canal trajectory.

Figure 9.10 Images provided automatically by the software for each case in different views.
The early detection of confluences, where canals split and join, is also clinically relevant because they often result in abrupt multiple hidden curvatures that can easily overstress instruments and cause intraoperative failure. The same problems can occur when multiple hidden curvatures are present (Figures 9.11–9.12). To overcome problems related to confluences, it was suggested that the less complex canal should be prepared to the canal terminus and the more complex only to the point where the canals merge [10]. Thus, besides precisely identifying the distance of confluence from the foramen, clinicians should also detect which canal is less complex. Unfortunately, such a precise understanding of root canal anatomy is nearly impossible using traditional radiography, and might be challenging even using CBCT images [11]. Moreover, there are no clinical recommendations on how the complexities of canal curvatures should be assessed in three dimensions.

Figure 9.11 Differences between clinical (buccolingual) and hidden (mesiodistal) view in a mesial root of a mandibular first molar as shown by software.

Figure 9.12 Differences between clinical (buccolingual) and hidden (mesiodistal) view in a mesial root of a maxillary first molar as shown by software.
Using such 3D software packages, the pathway of the root canal is rendered in three dimensions and the operator can make adjustments to position the line centred in the canal according to any view. This segmented line can then be visualised by changing the transparency of the virtual model of the tooth. To determine canal length (CL), the operator must choose one view. Usually, the canal length can be determined by the arithmetic mean between the buccolingual and mesiodistal lengths, which is likely to increase the accuracy because it also considers the mesiodistal aspect of the canal anatomy. However, even using both views, this approach is measuring the canal in two-dimensional projections of the CBCT scan. For canal length measurements in 3D EndoTM, the operator can adjust both the apical distance and the position of the coronal reference. In a recent study, 3D EndoTM was found to be reliable and similar to more complex conventional CBCT software in terms of precision, and it can be a useful tool if a scan is already available [4]. Nevertheless, the use of an electronic apex locator is still required to confirm and adjust the apical limit because the clinical working length is subjected to small changes during root canal preparation or changes to the access cavity.
9.1.3 Dynamic Navigation Systems (Software and Devices)
Dynamic navigation systems (DNS) were introduced to facilitate and improve the insertion accuracy of dental implants [12–14]. They integrate dental surgical instruments, medical imaging, and optical positioning devices with preoperative planning software for dental implants [13]. Through a clinically applicable interface that provides real-time display (Figure 9.13), these systems guide users to prepare the targeted position according to the output of the preoperative planning software [15]. Implantologists described this technique as ‘just like a GPS guides drivers,’ and they list several advantages: better accuracy in placing the implants, enhanced safety and aesthetics, less invasive, reduced discomfort of the patients, and improved recovery time [16].

Figure 9.13 Dynamic navigation system (DNS) in the clinic.
The great majority of currently published studies on DNS are related to implant placement [17, 18]. However, more recently, researchers proposed the use of this new technology in endodontics. Buchanan was the first author to publish a case report concerning the use of a Dynamic Guidance System, the X-GuideTM (X-Nav Technologies, LLC, Lansdale, PA, USA) [19]. He attributed the use of this technique to Dr Charles Maupin, an endodontist from Texas, USA. The X-Guide works by referencing two scanning drums, one secured to the patient’s jaw before CT imaging and the second secured to a latch-grip handpiece attachment. Two overhead cameras then trace these reference points allowing the computer processor to generate an avatar of the tooth and the bur in real time allowing the clinician to look at the computer screen while drilling into the tooth (Figure 9.14). Buchanan [19] reported three cases and concluded the technique to be very effective in locating canals and creating less invasive access preparations.

Figure 9.14 Principles of DNS Plan, Trace and Place.
In 2019, Chong and colleagues published an in vitro study concerning the advantage of DNS for locating canals in extracted teeth [20]. They reported that this technology was helpful mainly for the location of canals that otherwise could not be detected and negotiated with traditional techniques. In February of 2019, Burgess published a case report showing how DNS helped him in locating and treating a root canal through an access cavity cut into the palatal aspect of an existing prosthetic crown [21]. He reported that DNS allowed the preparation pathway to be monitored in three dimensions and provided guidance through the sclerosed coronal portion of the tooth, leading to accurate location of the canal. He concluded that the risk of lateral perforation was reduced and the damage to, and consequent weakening of, the portion of the tooth supporting the crown was decreased. The access cavity and location of canals were more safely and predictably performed, thus reducing the risk of iatrogenic errors and potentially improving the long-term prognosis of the tooth in such a complex case. Another case report was published in that same year by Nahmias [22] showing the potential of the DNS on locating and treating calcified canals. He used the NavidentTM system (ClaroNav, Inc., Toronto, ON, Canada) and precisely described the methodology and the advantages of the DNS comparing to traditional techniques, which are very complex, skill-dependent, and time consuming. A small volume CBCT was requested for the patient with slice thickness to be no more than 0.4 mm and the export format to include digital imaging and communications in medicine (DICOM) files in a standard multi-file configuration. The DICOM files were imported from the CBCT to the NavidentTM software and proceeded to develop a digital planning for the best path to take in order to locate the calcified canal. Obviously, the quality of the CBCT image is important, even if the system is designed to work with images from any CBCT. Small FOV scans are also preferable to reduce radiation dose.
Planning is the first step when using a DNS system (Figure 9.15) and the endodontist must determine the goals of the procedure, and plan the direct access. The software precisely indicates to which depth the operator would have to prepare to pass the calcification and locate the canal; the direction and angulation of the burs is also visualised in three dimensions on the computer screen. The second step is to match the CBCT images with the real jaw of the patient, which is also a fundamental procedure to ensure precision of the treatment (Figure 9.16). In the case report mentioned, an optical tracking tag (‘Head-Tracker’) was secured on the patient’s head to enable the NavidentTM system to track the patient’s maxilla. The black and white pattern on the tag is recognised and being constantly tracked by the system’s optical tracking sensor, providing the system with the accurate position of the patient’s jaw at any time during the procedure. The patient’s jaw is represented on the Navident’s screen by the CBCT image, functioning as a map, with the operator’s instrument, such as a bur, superimposed on it. The tracker can also be attached, using resin composite and adhesive bonding, to a tooth from the contralateral side; this tracker is mainly used for mandibular cases. Prior to starting the navigated access, the CBCT scan has to be mapped to the actual jaw in a process called registration. Traditionally, registration is achieved via a metallic fiducial marker that has to be attached to the patient’s jaw during the acquisition of the CBCT scan, and the radiographic image is later recognised by the software in the CBCT scan. Registration can be performed using such a fiducial marker, but NavidentTM recently developed an alternative registration method, called ‘trace registration’ (Figure 9.17). This method does not require the use of an artificial fiducial marker. Instead, it utilises the already existing, radiographically distinct anatomical structures in the jaw, such as teeth, abutments, and even bone. Therefore, it can avoid another visit because it eliminates the need for a special second scan to be taken with a metallic fiducial marker affixed to the jaw with a thermoplastic stent. Besides reducing the exposure to radiation, it reduces the chance for errors caused by stent dislocation during the scan and allows for the use of a small volume CBCT. As a consequence, it also minimises time and cost to the procedure.

Figure 9.15 DNS: plan.

Figure 9.16 DNS: trace.

Figure 9.17 DNS: trace registration.
In the NavidentTM software, three maxillary teeth must be marked on the CBCT scan (even if in endodontics it is probably better to select six markers for improved accuracy). These tooth surfaces are then traced with a tracer tool that is also tracked by an optical positioning sensor. During the tracing, the system continuously samples and records points on the traced teeth, which are then matched with the CBCT data, to register it with the physical patient’s jaw. The whole process usually takes only 1–2 minutes, but it must be carefully performed to ensure the best precision. The next step is burs/instruments calibration; the latest development of the software allows the use of both high-speed and low-speed handpieces, the use of piezoelectric devices (including surgical microtips), and even nickel titanium (NiTi) rotary files can be calibrated. In the case reported by Nahmias [22], a high-speed handpiece, also tracked by the system, was calibrated in a short two-step process: the axis was calibrated first, followed by calibration of the tip of the bur. This lets the system continuously track the direction and position of the bur and to report it to the user on the computer screen. The tip of a white 31-mm Munce Discovery bur (CJM Engineering Tech, CA, USA) was tracked and this bur was used to locate the calcified canal, but clinicians can use any kind of bur (in terms of shape, diameter, length) for the procedure. Before starting the actual drilling, a final accuracy check is recommended by placing the tip of the bur on the tooth to be accessed to make sure that it corresponds to the exact point on the tooth to be treated on the computer screen. This is done by touching the bur tip on distinct rigid landmarks (such as tooth cusps) in the jaw to be treated, and comparing the actual physical location of the bur to its on-screen position. This check can be repeated at any time during the procedure if the clinician is worried when drilling deep inside a small root canal.
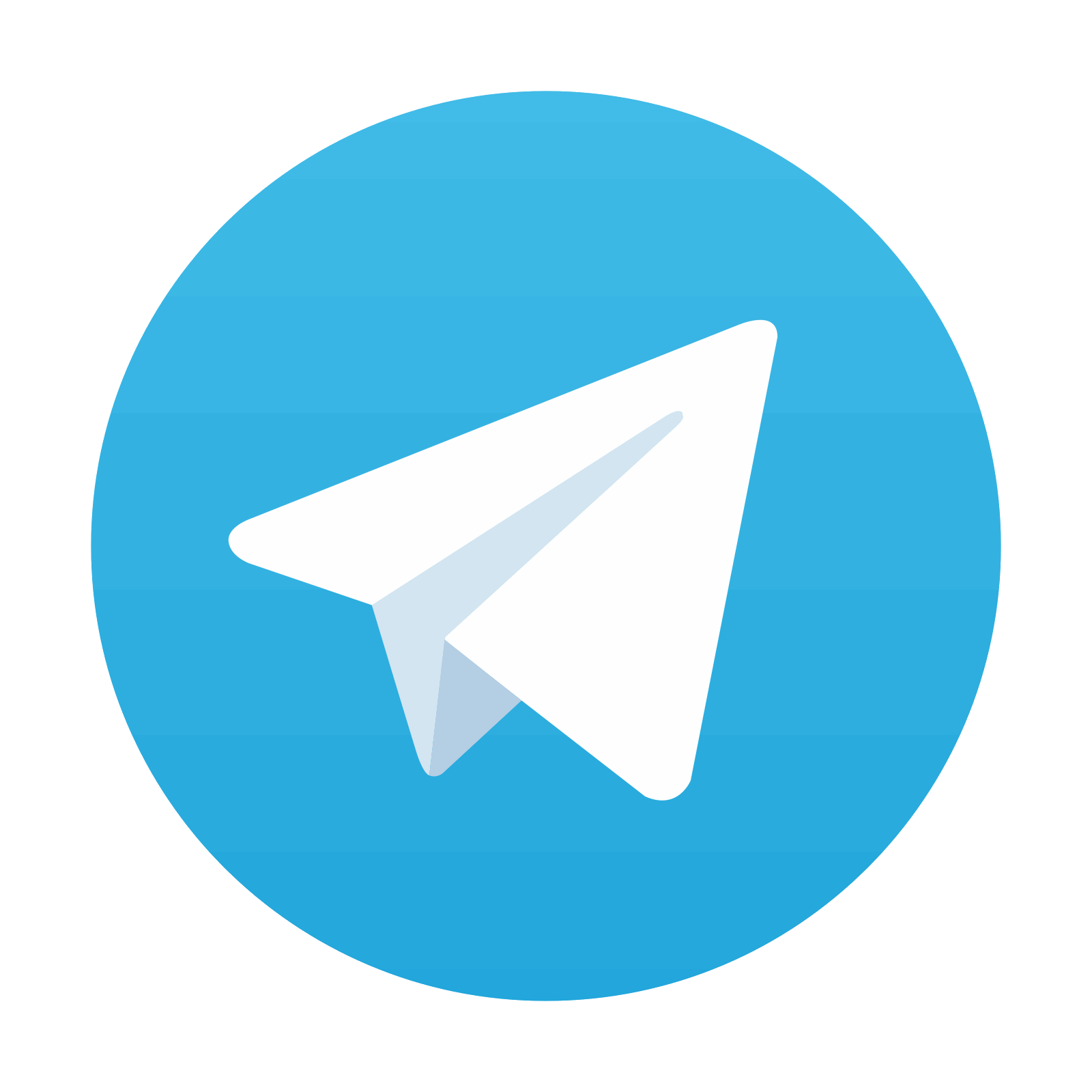
Stay updated, free dental videos. Join our Telegram channel

VIDEdental - Online dental courses
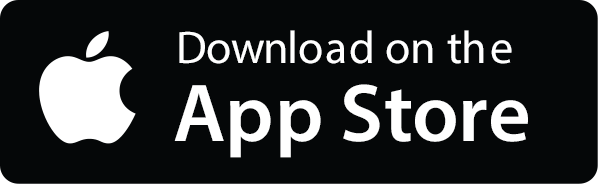
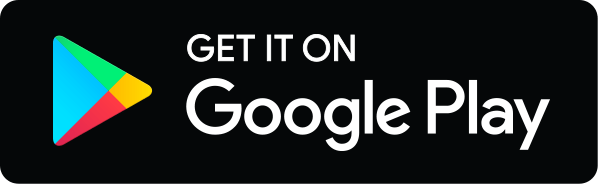