Reconstruction of soft tissue defects following tumor ablation procedures in the trunk and extremities can challenge the microsurgeon. The goal is not just to provide adequate soft tissue coverage but also to restore form and function and minimize donor site morbidity. Although the principles of the reconstructive ladder still apply in the trunk and extremities, free tissue transfer is used in many cases to optimally restore form and function. Microsurgery has changed the practice in soft tissue tumors, and amputation is less frequently necessary.
Key points
- •
Soft tissue tumors of the trunk and extremities represent a challenge because of the paucity of soft tissue and the relative close proximity with critical structures.
- •
A multidisciplinary team approach should be adopted, especially for the trunk and lower extremity.
- •
Every attempt should be made to preserve a limb. When amputation is inevitable, the remaining limb must be optimized to maintain function or to improve prosthesis control.
- •
Several flaps can be used to cover soft tissue defects in the trunk and extremity. Biological and synthetic materials can add to the wide variety of options in the armamentarium of the reconstructive surgeon.
General considerations in oncologic reconstruction
Thorough evaluation of the patients’ health status, functional demands, location, and extent of the tumor as well as the tissue loss expected with tumor ablation procedures must be done before attempting any course of treatment. A multidisciplinary team approach also helps the reconstructive surgeon in selecting the appropriate flap for the defect and minimizing donor site defects, especially for trunk and lower extremity reconstruction. Accurate assessment of the defect, meticulous dissection of the recipient site, precise microvascular anastomoses, and proper flap insets are essential factors for an optimal outcome. The timing of the reconstruction depends on the surgeon’s preference and the patients’ medical status, but it is preferable to do the reconstruction immediately after tumor resection. This approach decreases the number of operations and minimizes contamination of deep tissues and structures.
Reconstruction in patients with cancer is unique, as adjuvant chemotherapy and radiation therapy can affect wound healing and flap survival; neoadjuvant radiation therapy usually creates a zone of injury that extends beyond the margins of resection. Furthermore, patients with cancer are well known to be hypercoagulable and, therefore, have a higher risk for venous thromboembolism. Chemotherapy, radiation, and immobilization further increase the risk of thromboembolic events in this special patient population. All of these factors make oncologic reconstruction more challenging and necessitates careful planning and individualization of treatment plans.
General considerations in oncologic reconstruction
Thorough evaluation of the patients’ health status, functional demands, location, and extent of the tumor as well as the tissue loss expected with tumor ablation procedures must be done before attempting any course of treatment. A multidisciplinary team approach also helps the reconstructive surgeon in selecting the appropriate flap for the defect and minimizing donor site defects, especially for trunk and lower extremity reconstruction. Accurate assessment of the defect, meticulous dissection of the recipient site, precise microvascular anastomoses, and proper flap insets are essential factors for an optimal outcome. The timing of the reconstruction depends on the surgeon’s preference and the patients’ medical status, but it is preferable to do the reconstruction immediately after tumor resection. This approach decreases the number of operations and minimizes contamination of deep tissues and structures.
Reconstruction in patients with cancer is unique, as adjuvant chemotherapy and radiation therapy can affect wound healing and flap survival; neoadjuvant radiation therapy usually creates a zone of injury that extends beyond the margins of resection. Furthermore, patients with cancer are well known to be hypercoagulable and, therefore, have a higher risk for venous thromboembolism. Chemotherapy, radiation, and immobilization further increase the risk of thromboembolic events in this special patient population. All of these factors make oncologic reconstruction more challenging and necessitates careful planning and individualization of treatment plans.
Goals of reconstruction in the trunk
The primary goals of chest wall reconstruction are stabilization of thoracic skeletal defects that may alter respiratory mechanics, obliteration of intrathoracic dead space, protection of vital intrathoracic structures and suture lines, and soft tissue coverage of extrathoracic defects. Other considerations include avoiding lung herniation, counteracting substantial shrinking of the thorax, leading to thoracoplastylike effect, preventing entrapment of the scapula in posterior resections, protecting mediastinal organs against external impact, and maintaining a good cosmetic chest contour. Additional challenges exist when reconstructing the lower extremities. These challenges include restoring painless function be it ambulation in regard to the lower extremity or fine dexterity and hand function in regard to the upper extremity. The slower rate of nerve regeneration and the higher incidence of flap failure and wound complications add to the complexity of extremity reconstruction.
Free tissue transfer for trunk reconstruction
Chest wall tumors account for most indications for chest wall reconstruction in large retrospective series, which include primary lung cancer, primary chest wall tumors, contiguous breast cancer, and metastases. The most common benign chest wall tumors are osteochondromas, chondromas, fibrous dysplasia, and desmoid tumors. The most common primary malignancies are soft tissue sarcoma, chondrosarcoma, and Ewing sarcoma. Wide local excision is the mainstay of treatment. High-grade malignancies and desmoid tumors typically require 4-cm margins of normal tissue, whereas low-grade malignancies are typically resected with 1- to 2-cm margins. Bony involvement for many lesions necessitates resection of the entire rib or sternum with resection margin guidelines of one normal rib above and below the level of involvement. The excision of tumors with a wide margin of normal tissue leads to large defects that routinely require complex reconstruction of the chest wall and soft tissue coverage. Chest wall reconstruction after tumor resection should be approached in a systematic fashion. Depending on the type of tumor, extent of resection, and history of radiation, reconstructive options include primary closure, skin grafts, local flaps, pedicled muscular, musculocutaneous flaps, and free flaps.
The chest wall is divided into the anterior, lateral, and posterior regions. Prosthetic skeletal reconstruction is typically reserved for anterior chest wall defects greater than 5 cm or involving 3 or more contiguous ribs because of the increased chance of paradoxic chest wall motion. Posterior chest wall defects can tolerate up to a 10-cm diameter resection because of support from the scapula unless the defect extends beyond the fourth rib where entrapment of the scapula can occur during movement of the arm. However, the reconstructive plan must be tailored to each individual patient. Patients with borderline pulmonary function may require reconstruction for smaller defect to avoid postoperative insufficiency, and others with rigid chest walls from radiation or adhesions may tolerate larger defects without affecting pulmonary function. Synthetic options for skeletal support include polytetrafluoroethylene, polypropylene, Vicryl, mesh-methyl methacrylate sandwich, methyl methacrylate neo-ribs, osteosynthesis systems, and dedicated sternal prostheses. Biological options include autogenous tensor fascia latae, rib grafts, bovine pericardium, or acellular dermal matrix.
Intrathoracic infection from ongoing airway or esophageal leak is a life-threatening condition often attributed to the presence of a persistent pleural space and continuing empyema. Intrathoracic muscle transposition can augment the closure of the leak with well-vascularized tissue and prevent recurrence and the long-term sequelae of ongoing infection. This transposition can be performed with pedicled pectoralis major, serratus anterior, latissimus dorsi, and omentum flaps. In complex cases after multiple operations, local muscles are often no longer available for reconstruction because of transection or previous attempts at reconstruction. Intrathoracic free tissue transfer has been shown to provide an abundance of well-vascularized tissue with the versatility of accessing the entire intrathoracic cavity and reducing morbidity by covering the fistula repair and obliterating the pleural dead space in a single operation.
Extrathoracic soft tissue coverage should be anticipated preoperatively and requires a combined effort between the extirpative surgeon and the reconstructive surgeon for coverage of prosthetic devices, maintenance of intrathoracic integrity, and restoration of aesthetic contours while improving survival and minimizing donor site morbidity. Anticipation of further resections for recurrence is necessary to maximize the use of local flaps while preserving options for future reconstruction, and a variety of reconstructive algorithms have been described.
Recurrence is common after resection of soft tissue sarcoma in the chest, with 23% of patients experiencing a recurrence and 38% of those presenting as a local recurrence an average of 11.6 months after resection. The only factor improving survival after surgical resection of high-grade soft tissue sarcomas is wide negative margins in primary, recurrent, and metastatic lesions. The workhorse flaps of thoracic reconstruction are the pectoralis major, latissimus dorsi, and vertical rectus abdominis muscle (VRAM) or transverse rectus abdominis muscle (TRAM) flaps. Local options are limited when local muscles are invaded by tumor or have previously been sacrificed. Irradiated local muscle has also been shown to have an increase in complications when compared with nonirradiated muscle transposition for soft tissue coverage even leading to complete flap loss. In these cases of compromised local options, microsurgical augmentation of superiorly based rectus abdominis flaps with supercharged inferior epigastric vessels or free flap reconstruction can provide well-vascularized soft tissue coverage from outside the zone of injury ( Fig. 1 ).
Recipient vessels for anterior chest reconstruction are most commonly the internal mammary and thoracodorsal arteries. If these vessels have been included in the resection, the proximal transected stumps can be used end to end for arterial inflow. Other options include the lateral thoracic, subclavian, thoracoacromial, external carotid, and transverse cervical arteries. Posterior chest wall reconstruction is most commonly performed with the thoracodorsal artery as the recipient vessel. The circumflex scapular or a regional intercostal artery can also be used. The venous comitantes for the arteries are most commonly used for recipient veins. Other options include transposition of the external jugular and the cephalic veins.
Complex microsurgical reconstruction after tumor resection in the trunk reduces the morbidity of prolonged hospital stays, multiple operations for debridement, and open wounds in patients in whom local flap reconstruction is not feasible. Three microsurgical methods are generally used in chest wall reconstruction. Microvascular augmentation of blood flow in pedicled flaps via supercharging/turbocharging, free flap reconstruction of complex intrathoracic defects, and free flap reconstruction of extrathoracic soft tissue defects.
Large central chest wall defects can be challenging when local flaps are not possible because of resection or vascular compromise. Pedicled VRAM/TRAM flaps serve well in this setting because they provide the volume to obliterate dead space and provide very large skin paddles. In patients with abdominal scars from prior abdominal operations, microvascular augmentation with supercharging/turbocharging of the inferior epigastric vessels and even using bilateral inferior epigastric vessels as a bipedicled flap has been shown to improve perfusion. It is worth noting that vascular insufficiency can be identified before ligation of vessels by placing clamps on the inferior epigastric artery and vein and observing the flap. When performing pedicled VRAM/TRAM flaps in patients with history of internal mammary artery radiation or abdominal scars, microsurgical augmentation of inferior epigastric vessels can salvage flaps showing vascular insufficiency or enable transposition of a larger, well-perfused musculocutaneous bipedicled flap. In these circumstances, abandoning attempts of relying on these vessels for pedicle flap perfusion may be ill advised and free tissue transfer should be considered.
Complications after pneumonectomy or partial pneumonectomy can result in chronic empyema and bronchopleural fistula requiring multiple thoracotomies, drainage, and attempts at repair.
Outcomes for trunk reconstruction
Buttressing airway and esophageal leak and pleural space obliteration improve patient outcomes. Arnold and Pairolero reported a 73% success rate in 100 consecutive cases when performing open drainage with fistula repair, buttressing the repair with intrathoracic muscle transposition, and obliterating the pleural space. Another study by Chen and colleagues reported a 100% success rate in treatment of bronchopleural fistulae and chronic empyema with one-stage free latissimus dorsi and serratus anterior muscle flaps in 5 patients with 4 to 7 prior thoracotomies. Similarly, Hammond, and colleagues reported an 80% success in treating 5 patients with bronchopleural fistulae and empyema with free rectus abdominis and latissimus dorsi flaps. In addition, 2 patients with complex intrathoracic wounds were successfully treated with free omentum flap and latissimus dorsi flap coverage. Finally, Walsh and colleagues reported 100% resolution using free flaps in the treatment of empyema and bronchopleural fistula in 6 patients. In patients with bronchopleural fistulae and chronic empyema and no local options, free flaps can reliably provide the necessary volume of well-vascularized muscle to buttress fistula repair and obliterate dead space in a single stage.
Free flap extrathoracic reconstruction is infrequent but indicated when local muscles have previously been excised, irradiated, transected, or used in prior reconstruction attempts. Cordeiro and colleagues identified 2 groups that benefitted from free flaps over a 10-year experience of 192 chest wall reconstructions with 20 free flaps, including 13 unilateral rectus abdominis musculocutaneous flaps, one bilateral rectus abdominis muscle flap, 2 contralateral latissimus dorsi flaps, and 4 forearm filet flaps. The first group were best reconstructed with free rectus abdominis musculocutaneous flaps for large central defects with transected internal mammary vessels and sacrificed latissimus dorsi muscles from prior posterolateral thoracotomies. The second group is best served with forearm filet flap reconstruction of the shoulder when oncologically safe after forequarter amputations ( Fig. 2 ).
Free tissue transfer provides the benefit of distant nonirradiated tissue with the versatility of flap selection and choice of recipient vessel location allowing unrestricted extrathoracic soft tissue coverage in patients with no local options. The ability to use various flaps can allow the reconstructive team to simultaneously raise the flap while the extirpative surgeon is resecting the chest wall tumor reducing operative time and need for position changes. The reconstructive surgeon who is able to use these microsurgical techniques can provide reliable well-vascularized coverage in the most complex chest wounds. It is important to adhere to the basic principles of chest wall reconstruction and use the simplest, reliable option of intrathoracic or extrathoracic soft tissue coverage. Complete reconstruction in a single stage at time of resection should be the goal in order to shorten the duration of hospitalization and recovery in patients who often have already had a prolonged course.
Microsurgical extremity reconstruction
Soft Tissue Tumors of the Extremities
Extremities are complex structures, and tumors can arise from any of the several tissue types that comprise them. Fortunately, most upper extremity tumors are benign, the most common ones being ganglion cysts, enchondromas, and lipomas. Malignant tumors are fairly uncommon and account for less than 3% of upper extremity tumors. Sarcomas represent most upper extremity malignancies, with 15% of soft tissue sarcomas occurring in the upper extremity; most of these tumors are located proximal to the wrist. Soft tissue sarcomas, however, occur more frequently in the lower extremities in comparison with the upper extremities with a 3:1 ratio. With the exception of nerve sheath tumors, all musculoskeletal tumors have a mesodermal cell origin; therefore, benign and malignant tumors present similarly. Malignant tumors can be misdiagnosed as lipoma, ganglion cyst, or even infection in some cases, which highlights the importance of always maintaining a high index of suspicion.
Amputation Versus Limb Salvage
Once a malignant lesion in an extremity has been identified, the treatment plan usually involves radical resection or wide excision of the malignant mass. In the extremities, radical resection often means amputation, either above or below the elbow or knee. Historically, amputation of the involved extremity was the mainstay of treatment of soft tissue sarcomas because local excision resulted in high rates of recurrence.
Reconstructive surgeons who participate in the management of soft tissue defects following tumor resection are often confronted with the dilemma of whether to attempt to salvage a limb or proceed with amputation. The decision to attempt heroic measures to save the limb or to amputate generally depends on the location of the tumor, degree of invasion, number of compartments involved with the inherent loss of function, and the soft tissue defect that is expected to result from tumor resection. Moreover, if limb-preserving surgery would result in significant loss of function and morbidity, it should not be attempted and radical resection with amputation should be taken into consideration. When the decision to proceed with amputation has been made, the residual limb should be optimized for an eventual prosthesis fitting. Every attempt should be made to provide adequate bony length below a joint to allow for the attachment of the prosthesis sleeve. In case of below-knee amputation, a 14-cm residual limb length is required to allow for weight bearing with a prosthetic limb and to minimize energy expenditure with ambulation.
Residual limb coverage goals vary between upper and lower extremities. In both the upper and lower extremities, a primary objective is to provide adequate coverage and padding of bony prominences without excess or redundant soft tissue. The lower extremity residual limb, on the other hand, will be used for ambulation and weight bearing; thus, careful selection of flaps should be done to minimize late complications. Despite the numerous advances in prosthetics, the currently available prostheses still fall short in restoring form, function, and sensation, especially in regard to the upper extremity. Therefore, every attempt should be made to preserve the limb. When amputation is inevitable, the principle of spare parts should be used when the soon-to-be amputated limb can be used for reconstruction of large defects, obliterate dead space, and provide coverage for the residual limb (see Fig. 2 ). This practice allows for prosthesis fitting and faster return of functionality. It is possible to harvest the flap before amputation, which significantly reduces ischemia time.
Advancements in microsurgery and the increased efficacy of the available chemoradiation therapies have made limb salvage operations the gold standard in treating soft tissue tumors. Wide tumor resection, often referred to as an en bloc resection, is the removal of the mass together with at least a 2- to 3-cm surrounding margin of normal tissue. The hand and foot represent a challenge because they are composed of multiple compartments with little soft tissue in each compartment and specialized, glabrous skin for weight bearing and grasp. Moreover, important structures exist in close proximity; the delicate biomechanical balance that is necessary to produce a fine movement requires multiple structures to work in concert. Wide margin excision disrupts this balance because of loss of tendons, muscles, nerves, and bone, which eventually results in severe functional impairment. This unique set of challenges makes the role of the reconstructive microsurgeon more important; a successful reconstruction is not just measured by adequate wound coverage and padding of bony prominences but also by preservation of function, sensation, and cosmesis.
In the lower extremity, several studies have found no difference in the functional outcome between patients who were treated with amputation versus those in whom limb salvage surgeries were done. Soltanian and colleagues found that outcomes depend more on patient socioeconomic status and resources rather than on the initial treatment plan. The knee plays a critically important role in ambulation, and every attempt to salvage the knee should be made to improve the patients’ ability to ambulate independently.
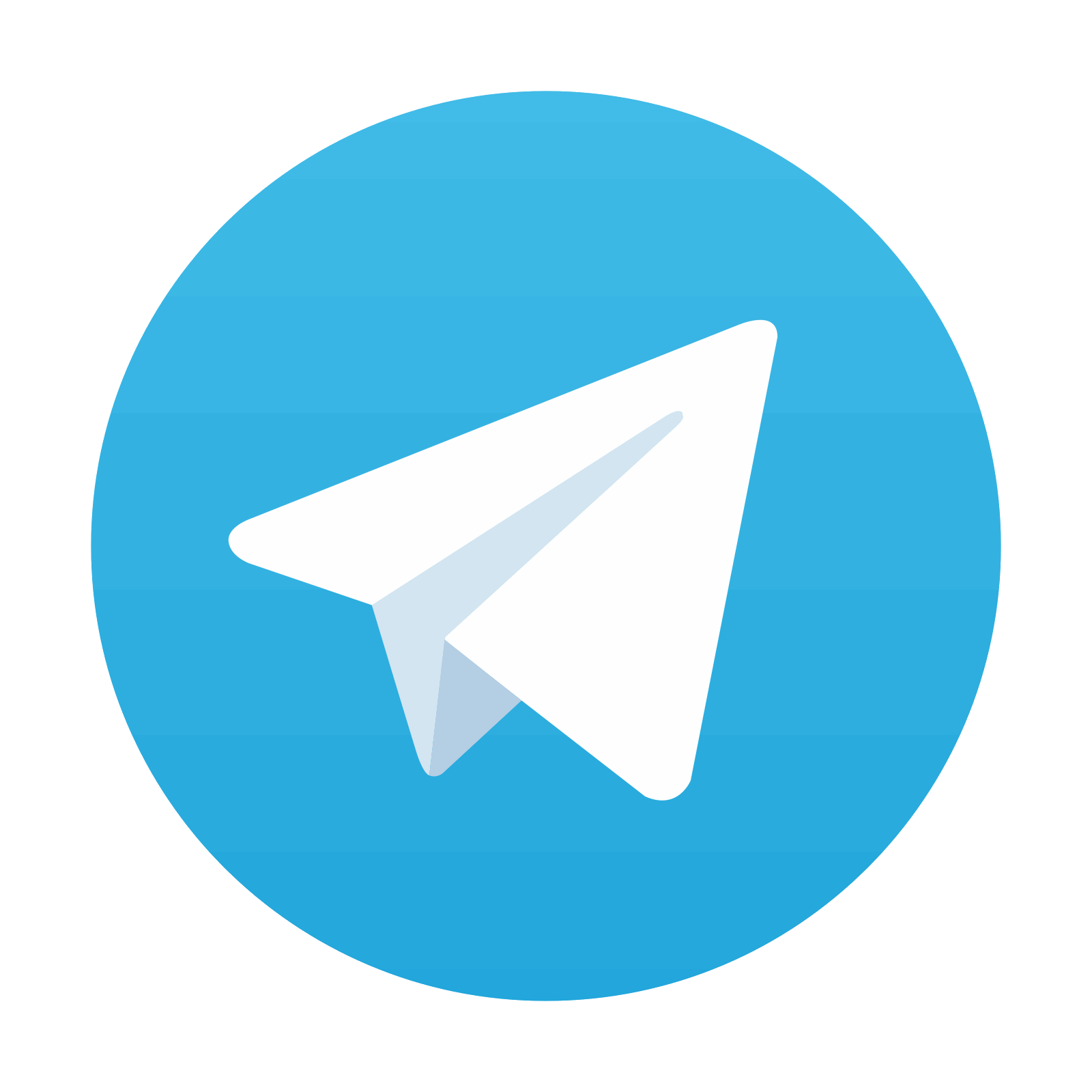
Stay updated, free dental videos. Join our Telegram channel

VIDEdental - Online dental courses
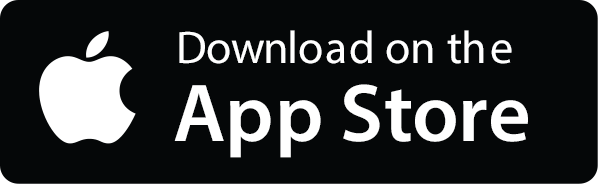
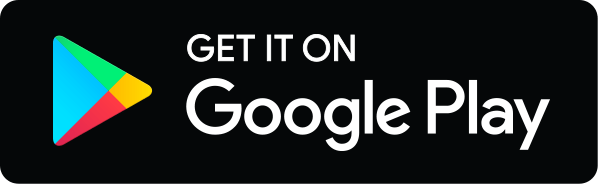