Abstract
Objectives
The absorbance profile of photoinitiators prior to, during and following polymerization of light curable resin-based materials will have a significant effect on the cure and color properties of the final material. So-called “colorless” photoinitiators are used in some light-activated resin-based composite restorative materials to lessen the yellowing effect of camphoroquinone (CQ) in order to improve the esthetic quality of dental restorations. This work characterizes absorption properties of commonly used photoinitiators, an acylphosphine oxide (TPO) and CQ, and assesses their influence on material discoloration.
Methods
Dimethacrylate resin formulations contained low (0.0134 mol/dm 3 ), intermediate (0.0405 mol/dm 3 ) or high (0.0678 mol/dm 3 ) concentrations of the photoinitiators and the inhibitor, butylated hydroxytoluene (BHT) at 0, 0.1 or 0.2% by mass. Disc shaped specimens ( n = 3) of each resin were polymerized for 60 s using a halogen light curing unit. Dynamic measurements of photoinitiator absorption, polymer conversion and reaction temperature were performed. A spectrophotometer was used to measure the color change before and after cure.
Results
GLM three-way analysis of variance revealed significant differences ( p < 0.001), where photoinitiator concentration (df = 2; F = 618.83) > photoinitiator type (df = 1; F = 176.12) > % BHT (df = 2, F = 13.17). BHT concentration affected the rate of polymerization and produced lower conversion in some of the CQ-based resins. Significant differences between photoinitiator type and concentrations were seen in color (where TPO resins became yellower and camphoroquinone resins became less yellow upon irradiation). Reaction temperature, kinetics and conversion also differed significantly for both initiators ( p < 0.001). Despite TPO-based resins producing a visually perceptible color change upon polymerization, the color change was significantly less than that produced with CQ-based resins.
Significance
Although some photoinitiators such as TPO may be a more esthetic alternative to CQ, they may actually cause significant color contamination when used in high concentrations and therefore manufacturers should limit its concentration in order to improve its esthetic quality.
1
Introduction
The significant increase in the use of photoactive resin-based composites (“white-fillings”) over dental mercury amalgams in restorative dentistry is primarily due to patients’ esthetic demands, the dentists’ material preference and governmental legislation on the use of mercury . The development of resin composite dental restoratives has continued since they were first introduced in the 1960s with much research being carried out in order to improve the setting reaction and photoinitiator chemistry, which is the primary absorber of light in resin based composites (RBCs) .
The α-diketone, camphoroquinone (CQ) is currently the most widely and successfully used photoinitiator in resin composite materials but has a distinct yellow tint, which does not bleach fully upon irradiation and may cause problems in color matching to natural teeth. Such discoloration may reduce esthetic quality and affect light transport through material thickness. Furthermore, the use of CQ also requires amine co-initiators such as dimethylamino ethyl methacrylate (DMAEMA) to efficiently produce free radicals to initiate polymerization. The use of such co-initiators is known to produce colored reaction by-products in the presence of light or heat which may further reduce esthetic quality and also cause problems in color matching to natural teeth . More recently alternative “colorless” photoinitiators have been introduced in attempts to improve esthetic quality and aid color matching particularly in bleach white and translucent shades. These photoinitiators may be based on either iodonium salts , onium compounds or Norrish Type I photoinitiator systems such as acylphosphine oxides and have recently been used by manufacturers either synergistically with CQ or as a stand-alone photoinitiator system. Additionally, the class of alternative photoinitiators based on acylphosphine oxides such as TPO (2,4,6-trimethylbenzoyl-diphenylphosphine oxide) do not require the use of amine co-initiators, which further increases the potential for esthetic quality. As well as improving esthetic quality, such initiators may have additional benefits of improved polymerization kinetics and mechanical properties , which further allows them to be a viable alternative to CQ.
Free-radical polymerization of methacrylate monomers used in dental RBCs is initiated by the excitation of suitable photoinitiator systems by light despite the monomer itself being capable of forming reactive species, although this is deemed inefficient . The photoinitiator chemistry affects light transmission, polymerization kinetics and overall conversion of monomer to polymer thus affecting material properties . It is well known that optimizing the concentration of the photoinitiator or co-initiator in a given shade/opacity of composite resin may lead to improved polymerization kinetics , esthetics , mechanical and/or biological properties . Furthermore, it is also known that increasing the spectral overlap between the light curing unit and the initiating system (either synergistically using more than one photoinitiator or using a more adapted emission spectrum centered on the photoinitiators absorption peak ) may also improve polymerization efficiency. Above a certain optimal level, any increase in photoinitiator concentration does not benefit final conversion and mechanical properties and esthetic quality may be compromised in the case of pigmented photoinitiators.
CQ has an absorption range from approximately 400 to 500 nm with a maximum excitation wavelength of 470 nm. Consequently, most dental light curing units are adapted for the excitation of CQ. TPO seems to be an interesting molecule despite its shorter wavelength absorption range since its higher molar absorptivity may provide greater efficiency to polymerize dental resins . Moreover, recent work has highlighted the potential of TPO-based (unfilled) polymers to be polymerized in thick layers , although inferior cure depths have been observed in resins and resin composites containing TPO compared with CQ . Although increased polymerization shrinkage stress of larger material volume may be a cause for concern , curing in bulk is an attractive option as it may reduce the need for the dentist to polymerize multiple thin layers in sequence and hence makes the treatment more efficient and less time consuming for patient and operator alike. Additionally, the use of TPO is not compatible with most conventional light emitting diode (LED) light curing units, which have an emission range limited to between 450 and 500 nm. Several manufacturers have now introduced so-called “third generation” wavelength LED units by combining LEDs with two or more different wavelengths that are capable of exciting alternative photoinitiators .
Consequently, the aim of this study was to analyze the photo-absorption characteristics and photo-bleaching characteristics of CQ and TPO throughout polymerization using a dynamic spectroscopy technique, which may have some significance for improved light transmission in depth and the perceived improvement of esthetic quality using alternative, non-yellowing photoinitiator systems. The two hypotheses that were tested were:
- (i)
CQ will exhibit a decrease in yellowing throughout and following polymerization.
- (ii)
TPO will not result in a significant change in color throughout or following polymerization.
2
Experimental procedure
2.1
Materials
Resin formulations were prepared in a ‘dark room’ using a 50/50 bis-GMA and TEGDMA mixture containing low (0.0134 mol/dm 3 ), intermediate (0.0405 mol/dm 3 ) and high (0.0678 mol/dm 3 ) concentrations of the photoinitiators 2,4,6-trimethylbenzoyldiphenyl phosphine oxide (TPO) and camphoroquinone (CQ) combined with the amine co-initiator, dimethylaminoethyl methacrylate (0.2:0.8 mass ratio). Resins were obtained from Sigma–Aldrich (Gillingham, UK) and either used as received or containing the inhibitor butylated hydroxytoluene (BHT) at 0.1% and 0.2% by mass. Prior to irradiation, resins were refrigerated in lightproof containers to limit premature activation. The chemical structures of the photoinitiators and the mechanism by which free radicals are produced and terminated are shown in Fig. 1 . A resin containing no photoinitiator and no inhibitor was used as the negative control. A quartz–tungsten halogen light curing unit (XL2500, 3M) having an irradiance of ∼800 mW/cm 2 at the exit surface of a 12 mm diameter fiber-bundle light source guide was measured on a handheld radiometer (Coltolux light meter) and verified using a UV–vis spectrometer (USB4000, Ocean Optics) and subsequently used to polymerize the specimens for 60 s. The light curing unit was chosen specifically for its broad-spectrum irradiance (∼400–530 nm) which overlaps the absorption spectrums of both photoinitiators (albeit only partially with TPO). The emission spectrum was measured by calibrating the UV–vis spectrometer using a deuterium tungsten light source (according to NIST standard) with known spectral output in the UV–vis and NIR range (Mikropack DH2000-CAL, Ocean Optics, Dunedin, USA). During the measurement of absolute irradiance, the measured irradiance of the light curing unit was controlled by stacking reflective neutral density filters (Thorlabs, UK) which produced a 6-fold reduction in irradiance over the whole spectrum. Static absorption spectra of the photoinitiators and the inhibitor BHT were determined in methyl methacrylate (Sigma–Aldrich, UK) using UV–vis spectroscopy.

2.2
Degree and rate of polymerization
Resins were carefully pipetted into cylindrical white Teflon molds (12 × 1.4 mm) with upper surface covered with glass cover slips and lower surfaces covered by glass microscope slides (1.2 mm thick). Degree of conversion ( DC ) and rate of polymerization ( R p ) were measured in real-time by Fourier Transform near infrared spectroscopy (FT-NIRS; Fig. 2 a ) by monitoring the height of the peak at 6164 cm −1 , which corresponded to the decomposition of the vinyl CH 2 absorbance. The progress of polymerization was monitored for up to 180 s post irradiation. DC was recorded 120 s post irradiation although it is known to evolve for up-to 24 h post irradiation with a log related decline in ‘dark cure’ .

2.3
Temperature measurements
Temperature rise was monitored dynamically during polymerization (Δ T max ) using a split ring mold containing a thermocouple ( Fig. 2 b). The thermocouple was glued in place so that the bead of the thermocouple (1.5 mm diameter) was in contact with the outer edge of the sample. The thermocouple was calibrated against a standard mercury thermometer. The change in temperature was recorded for an additional 240 s post irradiation. The temperature rise in resins containing no photoinitiator was 0.43 ± 0.1 C due to heat generated by the light curing unit and was used to correct resins containing photoinitiator to obtain temperature rise during polymerization.
2.4
Photoinitiator absorption
Photoinitiator absorption was measured in real time during irradiation at the maximum point of overlap between the photoinitiators and the light curing unit (CQ: 470 nm; TPO: 420 nm) by a technique adapted from Chen et al. . The UV–vis spectrometer (USB400, Ocean Optics) was set up so that a 200 μm fiber optic cable, which had a cosine corrector attachment (CC3-UV; diameter: 3.9 mm) was able to measure light of the appropriate wavelength from the center of the light curing unit ( Fig. 2 c). Due to the relatively high intensity of the light curing unit, reflective neutral density filters were stacked directly above the cosine corrector. The spectrometer was then calibrated with a sample within a cylindrical teflon mold (12 × 1.4 mm) containing no photoinitiator (covered with a microscope slide on the lower surface and cover slip on the upper). The distance between the top of the sample and the light curing unit was kept to 1 mm, which facilitated the use of a shutter that was placed between the light tip and top cover slip prior to irradiation. A shutter was used as preliminary studies identified fluctuations in light intensity during the first 10 s of light activation. After the first 10 s of light activation, the shutter was removed and light activation of the sample began. For sample measurements, real time absorbance was recorded using Spectrasuite software during irradiation of the sample.
2.5
Color analysis
A spectrophotometer (CM-2600d; Konica, Minolta) was used to measure the tristimulus values of the International Commission on Illumination (CIELAB) values based on D65 standard illuminant and a 10° observer with a 3 mm SAV aperture. The tristimulus values represent the amount of three primary stimuli (red, green and blue) required to give color match with the color stimulus. The spectrophotometer was first calibrated against a standard white tile provided by the instrument manufacturer. Color measurements were made before and after real time photoinitiator absorption measurements. The measurements were made whilst the samples were contained within the molds and the glass microscope slides but were placed on top of a white ceramic backing tile before each measurement. Color change was calculated according to the following equation:
Δ E = ( L 2 − L 1 ) 2 ( a 2 − a 1 ) 2 + ( b 2 − b 1 ) 2
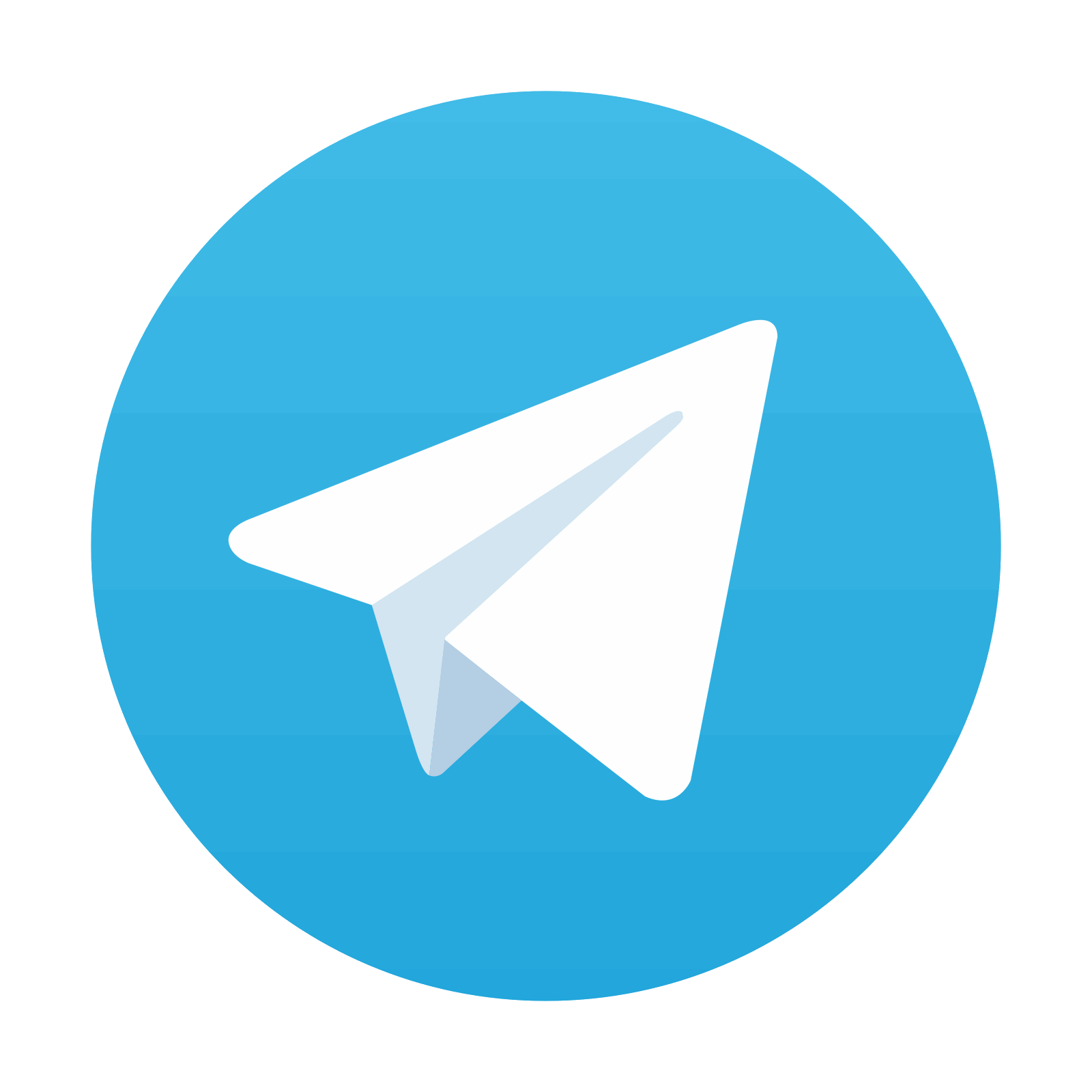
Stay updated, free dental videos. Join our Telegram channel

VIDEdental - Online dental courses
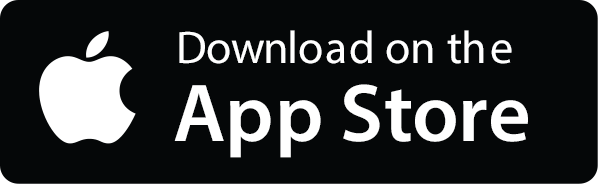
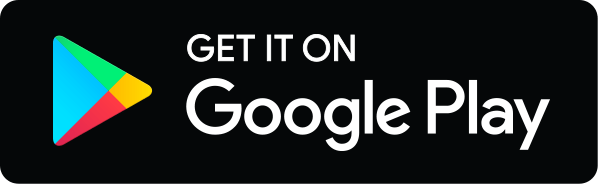