Introduction
The 3-dimensional load system (forces and moments) was quantified at the canine bracket during space closure with sliding mechanics with elastomeric chain on dry and wet stainless steel and beta-titanium 0.019 × 0.025-in archwires.
Methods
A custom-made maxillary dentoform simulating first premolar extractions was attached to an orthodontic force tester. The canine was attached to a load cell through an adaptor with silicone simulating the periodontal ligament. The orthodontic force tester simultaneously measured the entire load system produced at the canine bracket by an elastomeric chain. The effects of archwire material, time (activation and 1 hour), and lubrication were analyzed by using 3-way repeated measures analysis of variance (ANOVA, α = 0.05).
Results
Stainless steel provided a greater ( P = 0.001) load system than did beta-titanium. The force was greatest on the canine in the lingual axis. The greatest moment was about the y-axis. The moment-to-force ratio, the most critical ratio, was greater for beta-titanium than stainless steel; however, both were close to the ideal 10:1.
Conclusions
With a stainless steel archwire for retraction sliding mechanics, a canine will experience a greater load system in all 3 dimensions than with a beta-titanium wire. Both archwires produced a moment-to-force ratio adequate for translation.
The methods used to retract canines into an extraction space typically use sliding mechanics or closing loops. Although several studies have evaluated moments and forces on abutment teeth with various loops, there is little information on these load systems in 3 dimensions when elastomeric power chain is used to produce sliding mechanics on a continuous archwire in the wet and dry states. The use of sliding mechanics continues to increase in popularity, and so a better understanding of the 3-dimensional effect on the load system at the level of the bracket is needed. The use of sliding mechanics ideally involves the best combination of archwire material, archwire size, and force to permit the tooth to translate along the archwire with minimal friction and minimal loss of anchorage.
The size and cross-sectional geometry of the archwire are key components in sliding mechanics. As the size of the archwire increases, the forces required to overcome friction for a bracket to slide also increase. According to Kojima and Fukui, the tipping of the canine and the movement of the anchor teeth decreased when the wire size increased. The ideal archwire size would minimize unfavorable side effects from sliding mechanics to achieve the best translation of teeth into extraction sites.
Another variable when using sliding mechanics for canine retraction is the unpredictability of frictional forces. Frictional forces are generally greater with increased wire sizes and are influenced by the properties of archwire material. Stainless steel wires have a lower coefficient of friction than do beta-titanium-molybdenum alloy (beta-titanium) wires regardless of the bracket material. Increased friction leads to an increase in the force needed for sliding mechanics. Stainless steel wires have excellent formability, good corrosion resistance, moderate cost, and high values of stiffness and resilience. Stainless steel wires also have a high yield strength and a high modulus of elasticity, but lower maximum elastic strain compared with beta-titanium. Beta-titanium wires offer a highly desirable combination of strength, high springback (maximum elastic strain), and formability with low stiffness. However, many clinicians choose stainless steel wires rather than beta-titanium wires during space closure because the coefficient of friction is lower and the stiffness is greater compared to beta-titanium. The general thought is that stainless steel wires allow less tipping of teeth into the extraction sites. However, Kula et al suggested that beta-titanium wires could be as good as stainless steel wires for closing extraction spaces.
The use of elastomeric power chain is a possible source of force when using sliding mechanics. However, elastomeric power chain can permanently deform, resulting in a gradual loss of effectiveness over time. The significant force decay in the power chain is a clinical consideration. The previously mentioned studies examined the properties of elastomeric power chain, stainless steel wire, and beta-titanium wire separately. However, there is little information concerning the effects of elastomeric power chain and the types of wires in the 3-dimensional load system.
When testing friction, another variable is lubrication. In a comparative friction study, the resistance to sliding increased for both stainless steel and beta-titanium wires when tested with artificial saliva and whole human saliva. The increase in resistance was attributed to the adhesions and attractions among polar materials, water, and other liquids that can increase the friction. However, the authors of these studies assumed continuous contact between large areas of wires and brackets, not the 2-point contact between brackets and wires that occurs clinically.
There appears to be a general lack of information about the 3-dimensional load system concerning sliding mechanics and its many variables. The purpose of this pilot study was to determine the 3-dimensional load system created on a bracket when using sliding mechanics to close space on stainless steel and beta-titanium wires in dry and wet environments.
Material and methods
The force and moment components were measured on the canine bracket of a custom-made dentoform by using an orthodontic force tester ( Fig 1 ). The orthodontic force tester was designed and built for the load cell to simultaneously measure the moment and force components in the x, y, and z axes acting on the tooth attached to an archwire ( Fig 2 ).
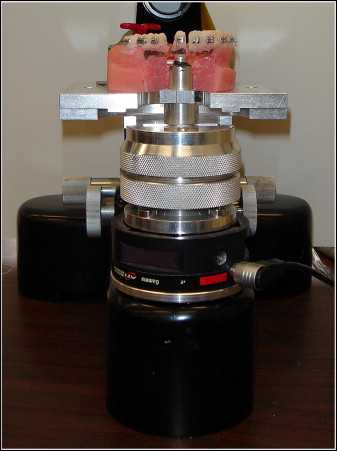
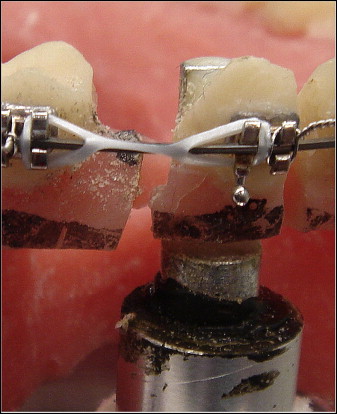
The orthodontic force tester had a moment/force load cell (multi-axis force/torque Gama; ATI Industrial Automation, Apex, NC) with resolutions of ± 0.2 N for the force components and ± 0.0025 N-m for the moment components. The canine was cut from a solid, 1-piece dentoform and attached to the load cell with an adaptor. The adaptor consisted of an artificial root connecting to the canine, an artificial periodontal ligament made of 0.3-mm silicon (Loctite Gasket Sealant 2; Henkel, Dusseldorf, Germany), and an aluminum socket connected to the load cell. This complex fulfilled 2 purposes: it connected the canine and the load cell, and it allowed a slight movement similar to that of a natural tooth in bone because of the periodontal ligament. The crown displacement was about 0.12 mm under a 3-N force, which was close to the 0.13 mm reported by Christiansen and Burstone. The custom-made dentoform represented an ideal maxillary arch already leveled and aligned, second molar to second molar, after the first premolars were extracted. A Trueform I arch form (G & H Wire, Greenwood, Ind) of 0.019 × 0.025-in stainless steel wire served as the guide for the fabrication of the dentoform. The archwire form was traced and then cut from a piece of 22-gauge steel to form a template to position the brackets. Omniarch 0.022-in brackets with the MBT prescription (GAC International, Bohemia, NY) were used for all teeth and placed onto the steel template. After autopolymerizing acrylic (Duralay; Reliance Dental Manufacturing, Worth, Ill) was placed onto the mesh of each bracket, the steel template with the attached brackets was aligned to the acrylic teeth, and the acrylic was allowed to cure. Once the brackets were cured onto the dentoform, an impression was made with low-viscosity polyvinylsiloxane (Examix NDS; GC America, Tokyo, Japan) along the buccal surfaces of the teeth, and the polyvinylsiloxane was allowed to set. When the polyvinylsiloxane was set, a thin layer of petroleum jelly was applied to its outer layer. A second impression by using medium-viscosity polyvinylsiloxane (Examix NDS; GC America) was made over the initial impression. The initial impression served as a blockout material registering the proper placement of the brackets in addition to permitting easy removal of the impression material without distortion. The low-viscosity impression was removed, and duplicate brackets were placed in this custom guide. With this guide, the secondary impression was placed over the initial impression, and tooth-colored autopolymerizing acrylic was poured into the impression. After polymerization of the teeth, the remainder of the impression was filled with a pink, gingiva-colored, autopolymerizing resin and allowed to set under pressure to minimize further dimensional changes. The 1-piece dentoform, with brackets, was removed from the impression after polymerization. To allow room for the load cell, some acrylic was removed from the first premolar to the lateral incisor. The canine was then cut entirely from the dentoform after the apical base was attached to the load cell through the adaptor, which ensured alignment of the canine with the rest of the teeth.
Two types of 0.019 × 0.025-in preformed archwires were chosen for this study: Trueform I OrthoForce stainless steel and Trueform I Orthoforce BT3 beta titanium (beta-titanium) (G & H Wire), with each material type from the same lot. These wires were chosen after visual comparison between the arch forms for stainless steel vs beta-titanium. G & H wires had the greatest consistency between the 2 wire materials and the chosen arch form when compared with similar arch forms from GAC and Ormco (Glendora, Calif). Ten stainless steel and 10 beta-titanium wires were tested in the dry state, and 10 stainless steel and 10 beta-titanium wires were tested in the wet state, for a total of 40 wires. Before each wire was tested, the orthodontic force tester was reset to clear previous readings. The moments and forces on the canine were evaluated upon initial activation and after 1 hour. Lubricant was placed only on the canine bracket and wire by using a medical dropper to dispense 2 drops of the distilled water at initial placement and 1 hour. Since the use of cleaners to remove saliva residues could affect the sensor, distilled water was chosen over artificial or human saliva because of the sensitivity of the load cell.
Similar to a clinical situation, the first molar and the second premolar were ligated together by using 0.010-in stainless steel ligatures over the archwire to provide anchorage for the posterior movement of the canine. The mesial wings of the canines were also tied with 0.010-in stainless steel ligatures over the archwire. Elastomeric power chain (Alastik; 3M Unitek, Monrovia, Calif), 2 modules in length, was ligated from the mesial wing of the second premolar bracket to the distal wing of the canine bracket ( Fig 2 ). This form of ligature was chosen because it has been a choice of some clinicians. The force was measured by using a tension gauge (Dontrix 16-oz; E.T.M., Monrovia, Calif). The 2 links provided 3.33 N of force upon initial activation. The tension was not measured after 1 hour of activation.
The initial placement and 1-hour time intervals were determined after evaluation of the preliminary studies. The preliminary data showed consistency of the transducer readings within the specified resolutions during a 3-hour period without a load. However, additional studies showed that the transducer was the most consistent when readings were taken within an hour. This testing duration was sufficient to evaluate changes caused by sliding mechanics.
Statistical analysis
A 3-way repeated-measures analysis of variance (ANOVA) was performed to evaluate the effects of the wires (beta-titanium and stainless steel), time (0 and 1 hour), and wet vs dry states on each outcome. Pair-wise comparisons between treatment combinations were adjusted for multiple comparisons by using the Tukey procedure, assuming an overall significance level of alpha = 0.05. Analyses were performed on the ranks of the measurements to convert to a nonparametric test.
Results
The coordinate system for the forces and moments at the bracket are shown in Figures 3 and 4 , respectively. The results for each force and moment component of each archwire material, duration, and lubrication are shown ( Tables I-III ). All outcomes were analyzed with 3-way ANOVA. When interactions between factors (wire type, duration, and lubrication) were not significant, the results were presented as an overall effect. However, when the interactions were significant, the comparisons were examined at the levels of the other factors involved.
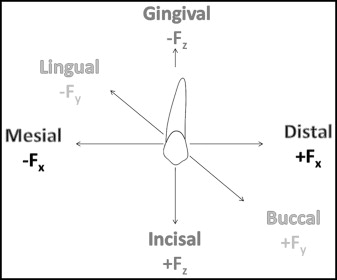
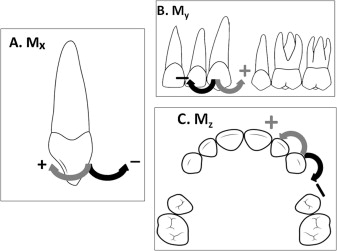
F x (N) | F y (N) | F z (N) | M x (N-mm) | M y (N-mm) | M z (N-mm) | |
---|---|---|---|---|---|---|
Stainless steel (mean ± SE) | 2.09 (0.03) | −5.29 (0.08) | −1.88 (0.09) | −3.52 (0.28) | −20.10 (0.28) | 10.11 (0.11) |
Beta-titanium (mean ± SE) | 1.63 (0.04) | −3.79 (0.08) | −1.24 (0.07) | −1.19 (0.28) | −16.84 (0.35) | 8.65 (0.19) |
Difference | 0.46 (0.08) | 1.49 (0.21) | 0.64 (0.08) | 2.33 (0.51) | 3.26 (0.85) | 1.46 (0.45) |
% difference | 22 | 28 | 34 | 66 | 16 | 14 |
P value | 0.0001 | 0.0001 | 0.0001 | 0.0001 | 0.0003 | 0.0002 |
F x (N) | F y , stainless steel (N) | F y , beta-titanium (N) | F z (N) | M x (N-mm) | M y (N-mm) | M z (N-mm) | |
---|---|---|---|---|---|---|---|
0 hour (mean ± SE) | 1.95 (0.05) | −5.31 (0.11) | −3.79 (0.12) | −1.55 (0.09) | −1.92 (0.25) | −19.07 (0.36) | 9.36 (0.18) |
1 hour (mean ± SE) | 1.78 (0.04) | −5.26 (0.11) | −3.79 (0.11) | −1.56 (0.09) | −2.79 (0.37) | −17.88 (0.38) | 9.40 (0.18) |
Difference | 0.17 (0.01) | 0.05 (0.01) | 0.00 (0.01) | 0.01 (0.01) | 0.87 (0.32) | 1.19 (0.13) | 0.05 (0.08) |
% difference | 9 | 1 | 0 | 1 | 45 | 6 | 0 |
P value | 0.0001 | 0.0001 | 0.99 | 0.0063 | 0.0166 | 0.0001 | 0.47 |
F x (N) | F y (N) | F z (N) | M x (N-mm) | M y (N-mm) | M z (N-mm) | |
---|---|---|---|---|---|---|
Dry (mean ± SE) | 1.75 (0.07) | −4.68 (0.19) | −2.33 (0.08) | −3.42 (0.50) | −18.69 (0.59) | 9.44 (0.31) |
Wet (mean ± SE) | 1.90 (0.04) | −4.47 (0.13) | −1.17 (0.05) | −1.68 (0.28) | −18.41 (0.40) | 9.32 (0.15) |
Difference | 0.15 (0.08) | 0.21 (0.20) | 1.17 (0.08) | 1.75 (0.52) | 0.28 (0.81) | 0.12 (0.43) |
% difference | 9 | 4 | 50 | 51 | 1 | 1 |
P value | 0.34 | 0.4 | 0.0001 | 0.006 | 0.51 | 0.28 |
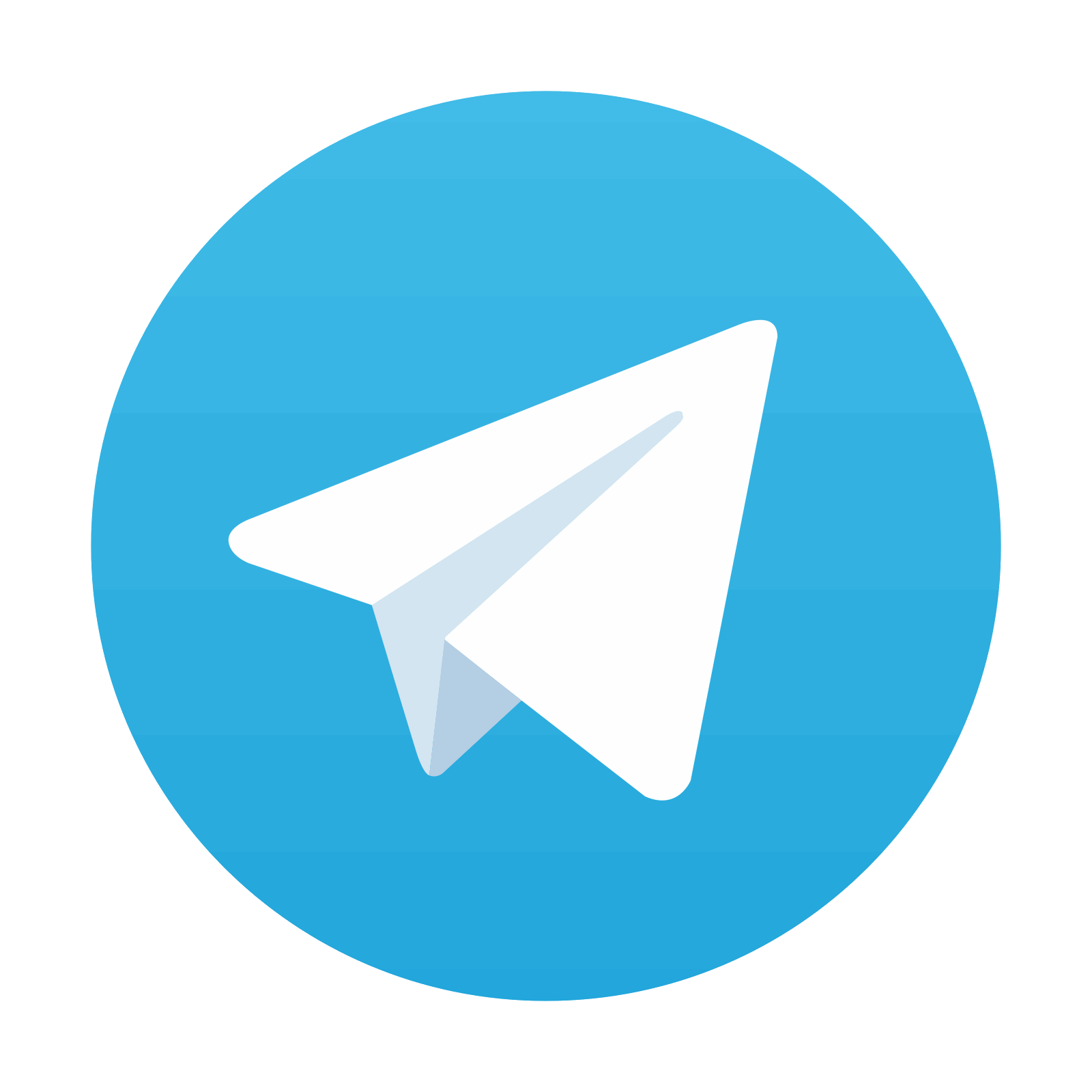
Stay updated, free dental videos. Join our Telegram channel

VIDEdental - Online dental courses
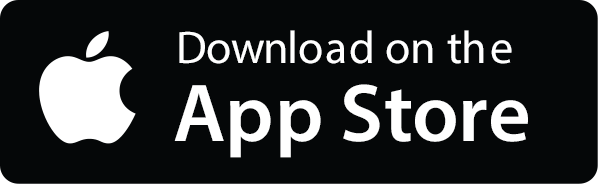
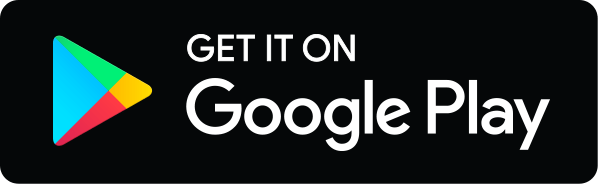
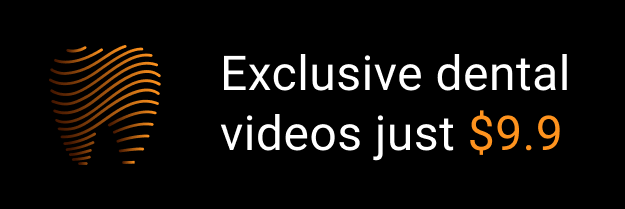