Abstract
This study evaluated the clinical outcome and the crestal bone resorption of implants placed with high insertion torque (up to 80 N cm). 102 patients were treated with 156 tapered implants. 42 implants (control group) presented insertion torque between 30 and 45 N cm (mean = 37.4 SD 8.2). 114 implants (experimental group) were placed with insertion torque between 50 and 80 N cm (mean = 74.8 SD 7.9). All implants were early loaded after 2 months. Peri-implant marginal bone levels were assessed immediately after surgery, and at 6- and 12-month follow up examinations. At the 12-month follow up all implants were clinically stable. After 12 months, patients in the experimental group lost an average of 0.41 mm (CI 95% 0.522; 0.263) of crestal bone compared with 0.45 mm (CI 95% 0.561; 0.286) for those in the control group. There were no significant differences between the two groups. No direct or inverse relationship was observed between the insertion torque values and crestal bone resorption. The results show that the use of high insertion torque (up to 80 N cm) did not prevent osseointegration and did not increase bone resorption around tapered implants early loaded up to 1 year after implant placement.
Primary implant stability and lack of micromovements are considered to be two of the main factors necessary for achieving predictable high success in immediate and early loading protocols. A successful osseointegrated oral implant is anchored directly to bone, however, in the presence of movement a soft tissue interface may encapsulate the implant causing its failure. It has been demonstrated that micromovements are not dangerous and do not interfere with healing if they are within a range of 50–150 μm. Implant micromovements below this range are presumed to be innocuous to bone remodeling and may even account for the denser osseous interface observed around implants immediately loaded as compared with delayed loading. Clinicians are faced with the dilemma of how to attain good primary stability of the implant and avoid excessive micromovements. Factors that affect primary implant stability involve geometrical features related to implant, bone (density, quality and quantity) and surgical procedures (drill diameter, depth of the preparation and tapping of the implant site). The clinical perception of implant stability is commonly related to rotational resistance (insertion torque) during implant placement. Considering that implant stability is influenced by the interplay between implant design and the surrounding bone, it has been suggested that by increasing the insertion torque it is possible to improve the implant’s primary stability and integration. To achieve good primary stability, it has been suggested that implants have to be inserted with a torque of at least 35 N cm for an immediate loading protocol. There is no consensus on the maximal insertion torque allowed. Recently, the use of a slightly narrower final drill with a tapered implant design has been associated with elevated insertion torque and high success rates. A histological study in rabbits found that bone condensation (underpreparation of the implant site), improved peri-implant bone formation during the 8 weeks after implantation. If localized stress is too great, however, it could lead to ischemia and localized bone necrosis at the implant-bone interface. This phenomenon is generally viewed as being limited to cortical bone. High compression caused by an insertion torque higher than 40–45 N cm has been said to disturb the local microcirculation, leading to necrosis of the osteocytes and to bone resorption. Although these concepts are widely accepted in the literature, no study has been published proving these hypotheses. The aim of this prospective study was to compare the clinical outcome and the crestal bone resorption of implants placed with high insertion torque (50–80 N cm) and implants placed with insertion torque of 45 N cm or less. The present study reports the outcome up to 10 months after loading; 12 months after implant placement. This could be a first step to investigate if high insertion torque cause increased bone resorption due to pressure necrosis and higher failure rates. The present article is reported according to the STROBE statement for improving the quality of observational studies ( http://www.strobe-statement.org ).
Materials and methods
This was a nonrandomized, prospective clinical study. Patients were recruited and treated in two different private practices in Italy between October 2009 and January 2011. The principles outlined in the Declaration of Helsinki on clinical research involving human subjects were adhered to. The study was exempt from institutional review board approval and chart review because the operators did not vary the torque on purpose. They simply recorded the insertion torque and then assigned the implants to high torque and low torque groups, based on torque value. All patients received thorough explanations and gave written informed consent before being enrolled in the trial.
Candidates were partially edentulous patients who presented with one or more missing teeth in the maxillary and/or mandibular jaw. All patients were subjected to a preliminary evaluation that included careful review of their medical and dental histories, detailed clinical examination, and evaluation of oral hygiene. Patients were included consecutively in the study, provided that they fulfilled the following inclusion criteria: at least 18 years of age; sufficient bone volume for placement of implants at least 8 mm in length and 3.7 mm in diameter (bone dimensions were measured on preoperative computer tomography (CT) scans); healed bone sites, at least 4 months postextraction; and adequate oral hygiene, i.e. plaque index ≤2. Exclusion criteria were patients with: systemic disease that could compromise osseointegration; previous irradiation in the head and neck area; treatment or under treatment with intravenous amino-bisphosphonates; uncontrolled diabetes; substance abuse; heavy smoking (over 20 cigarettes daily); and acute or chronic infection/inflammation in the area intended for implant placement.
All patients were instructed to use 0.2% chlorhexidine mouth rinse twice daily, commencing 3 days before the intervention and thereafter for a 2-week period. Anti-microbial prophylaxis was obtained with the use of 1 g β-lactam antibiotic (amoxicillin, Pfizer Manufacturing, Puurs, Belgium) twice daily for 6 days, starting 1 h before surgery. On the day of surgery, the patient was anesthetized by local infiltration using articaine with adrenaline (1:100,000). A midcrestal incision was performed, followed by elevation of a mucoperiosteal flap that was kept small to preserve the periosteal vascular supply. The osteotomy was prepared and the implants were placed at the crestal level. Care was taken to undersize the osteotomy to achieve high insertion torque values. Operators followed the drilling sequence recommended by the implant manufacturer, but they underprepared with a drill of one size less than the one recommended at implant sites characterized by soft bone quality. All patients received tapered self-tapping implants (JDEvolution ® , JDentalCare, Modena, Italy) with dual thread. The final insertion torque was measured with a calibrated torque wrench (JDTorque ® , JDentalCare). The wrench used was able to perform torque measurement within a range of 15–80 N cm, with 5% precision. Implants placed with an insertion torque of 45 N cm or less were inserted in the control group, implants placed with an insertion torque of 50 N cm or more were inserted in the experimental group. After placement, a healing collar was attached to the implant and the soft tissues were sutured around it with 4–0 vicryl sutures (Ethicon, Inc., Somerville, NJ, USA). The implant was allowed a non-submerged healing period of 2 months.
The definitive prostheses were delivered 2 months after surgery according to an early loading protocol. Patients were recalled for prophylaxis and instruction by a dental hygienist every 3 months.
Patients were recalled 6 and 12 months after surgery to assess the following outcome measures. Implant failure was assessed by implant mobility and removal of stable implants dictated by progressive marginal bone loss or infection. The metallic handles of two instruments were used to assess implant stability. Biological or prosthetic complications wre noted. Peri-implant marginal bone level changes were evaluated on intraoral radiographs taken with the paralleling technique at implant placement, 6 and 12 months after implant placement. The measurements of bone level changes were made by an independent outcome assessor. Radiographs were scanned, digitized in JPG format, converted to TIFF format with a 600 dpi resolution and stored in a personal computer. Peri-implant marginal bone levels were measured using Image J 1.42 software (National Institute of Mental Health, Maryland, USA). The software was calibrated for every image using the known implant length. Measurements of the mesial and distal crestal bone levels adjacent to each implant were made to the nearest 0.01 mm and averaged at patient level and then group level. The measurements were taken parallel to the implant axis. Reference points for the linear measurements were the most coronal margin of the implant collar and the most coronal point of bone-to-implant contact.
Statistical analysis
Statistical analysis was performed using the statistical package StatView (version 5.01.98, SAS Institute Inc., Cary, NC, USA). Significance was considered at p < 0.05. The paired-samples t test was used to evaluate the bone level changes. The mesial and the distal measurements on each implant were averaged, then were averaged at group level. The relationship between insertion torque and marginal bone resorption was tested with Pearson’s correlation coefficient.
Results
107 patients were screened for eligibility, but 5 patients were not included because they were hesitant about receiving implant treatment. 102 patients were considered eligible and were enrolled in the study consecutively. All patients were treated according to the allocated intervention, no dropout occurred up to 1 year after implant placement and the data from all patients were evaluated in the statistical analyses.
Patients were recruited and subjected to intervention from October 2009 to January 2011. The main patient characteristics are presented in Table 1 . Patients were generally healthy, though 11 patients had medication controlled hypertension and 5 patients had controlled non-insulin diabetes. For 26 patients (15 women; 11 men) with an average age of 55.3 years (range 43–67 years; SD 7.4), 42 implants (39 in maxilla, 3 in the mandible) were placed with insertion torques between 30 and 45 N cm (control group). For 76 patients (49 women; 27 men) with an average age of 51.8 years (range 39–65 years; SD 8.3) 114 implants (35 in the maxilla, 79 in the mandible) were placed with insertion torques between 50 and 80 N cm (experimental group). The mean age of the patients at the time of surgery was 62.3 years (SD = 9.4; n = 47). The dimensions (diameter and length) of the inserted implants are listed in Table 2 . Measurements of insertion torque were averaged at group level. Average insertion torque was 37.4 (SD 8.2) for the control group and 74.8 N cm (SD 7.9) for the experimental group. The follow up focused on the period between implant placement and 12 months thereafter. Participants attended clinic visits at baseline and after 6 and 12 months.
Experimental group | Control group | |
---|---|---|
Patients | 76 | 26 |
Males | 27 | 11 |
Females | 49 | 15 |
Mean age at implant insertion | 51.8 | 55.3 |
Age range (years) | 39–65 | 43–67 |
Smokers (less than 20 cigarettes/day) | 17 | 6 |
Controlled diabetes | 4 | 1 |
Hypertension | 7 | 4 |
Number of implants by length (mm) | Number of implants by diameter (mm) | ||||||
---|---|---|---|---|---|---|---|
Study group | 8 | 10 | 11.5 | 13 | 3.7 | 4.3 | 5 |
Control | 10 | 6 | 15 | 11 | 11 | 29 | 2 |
Experimental | 19 | 42 | 27 | 26 | 25 | 82 | 7 |
After 10 months of function, all implants ( n = 156) were clinically stable. The radiographic data are summarized in Table 3 . Measurements from postoperative radiographs were analysed in order to verify any differences between the control and experimental groups at baseline: there were no significant baseline imbalances between the two groups ( p value = 0.854). The average value at baseline is −0.002 mm in the experimental group and −0.001 mm in the control group, which means that the implants were positioned at crestal level or slightly apical to it. After 12 months, implants in the experimental group lost an average of 0.41 mm (CI 95% 0.522; 0.263) of peri-implant bone compared with 0.45 mm (CI 95% 0.561; 0.286) for those in the control group. There were no statistically significant differences between the two groups for peri-implant bone level changes at the 12-month follow up ( p = 0.9). No direct or inverse relationship was observed between the insertion torque values and peri-implant bone resorption (Pearson’s correlation coefficient = 0.6481). No relationship was observed when the same analysis was performed using the values for peri-implant bone resorption observed after 6 months in case necrosis due to a high torque value had occurred at earlier time points after surgery (Pearson’s correlation coefficient = 0. 4705).
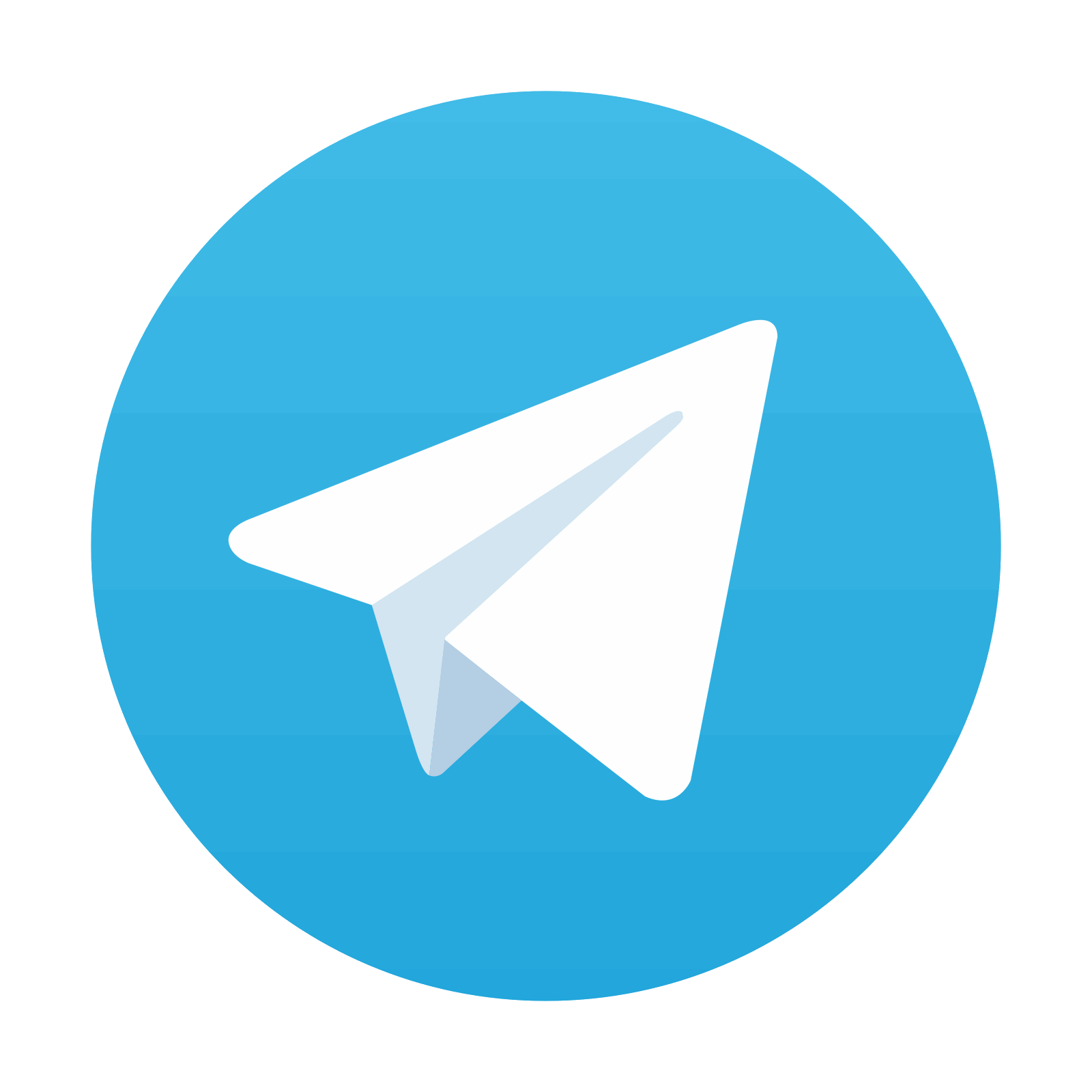
Stay updated, free dental videos. Join our Telegram channel

VIDEdental - Online dental courses
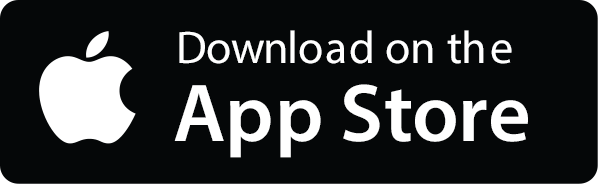
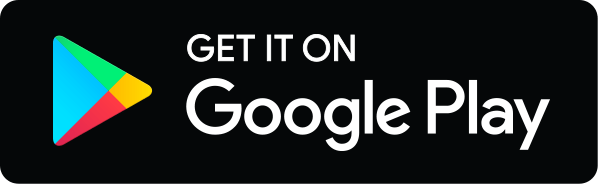
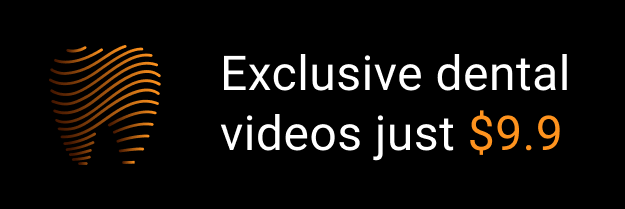