4
Clinical, Microbiological, and Immunological Risk Indicators of Peri‐implant Mucositis and Early Peri‐implant Bone Loss
Georgios N. Belibasakis1, Daniel Manoil1,2, Ali Gürkan3, and Nagihan Bostanci1
1 Division of Oral Diseases, Department of Dental Medicine, Karolinska Institute, Huddinge, Stockholm, Sweden
2 Division of Cariology and Endodontics, University Clinics of Dental Medicine, Faculty of Medicine, University of Geneva, Geneva, Switzerland
3 Department of Periodontology, School of Dentistry, Ege University, Bornova, Turkey
Introduction
Osseointegrated dental implants have undoubtedly advanced reconstructive dentistry, by adding a powerful treatment alternative to the armament of the clinician. They can be further considered in the treatment planning for restoring masticatory, occlusal, and esthetic functions of missing teeth. A unique feature, amid other types of endosseous implants, of being partially exposed to a microbial‐rich environment, the oral cavity, which naturally renders them prone to biofilm colonization. Not unexpectedly, the increasing number of implants inserted worldwide coincides with an overall increase in peri‐implant infections [1].
Because dental implants are expected to functionally substitute to natural teeth, there is also a tendency to perceive diseases of ailing implants as pathologies analogous to gingivitis and periodontitis [2]. Yet, a number of factors attributed to the structural and immunological variability of the peri‐implant tissues render them more susceptible to endogenous oral infections, compared to natural teeth [2–4]. However, as further described in this chapter, the etiopathological basis of these two groups of oral diseases is not entirely the same. Increasing evidence indicates that the peri‐implant ecosystem differs from the natural one established in the gingival crevice around periodontal tissues, reflecting a compositionally distinct microbiota [5]. Whereas this microbiota may evolve in homeostasis with the host, ecological changes may readily trigger and dysbiotic compositional shifts that further induce inflammatory alterations and ultimately lead to the destruction of the implant‐supporting tissues.
Diagnostic Epidemiological Aspects of Peri‐implant Infections
Diagnosis of peri‐implant infections primarily relies on the clinical and radiographical signs of the induced inflammatory changes. Accordingly, diagnostic criteria for peri‐implant mucositis include bleeding on probing (BOP), yet without radiographic signs of crestal bone loss beyond the initial remodeling [6]. In contrast, radiographic evidence of osteolysis, typically appearing as a symmetrical “saucer‐shaped” crestal defect around the implant, is central to the diagnosis of peri‐implantitis. Clinically, an increased probing pocket depth (PPD), as compared to previous examinations is also indicative of peri‐implantitis; however, the angulation, insertion depth, and threads of some implants may render peri‐implant probing challenging [6]. BOP‐positive peri‐implant sites run a higher probability to be diagnosed with peri‐implantitis [7], whereas suppuration appears to be very much limited to peri‐implantitis cases [8], and its presence is a good discriminant between health or peri‐implant mucositis and actual peri‐implantitis [9]. The prevalence of peri‐implantitis has been reported at 28–56% of implant‐baring patients, or 12–43% of the total number of implants [10], with a weighted mean prevalence at 22% [11]. Overall, about 1/3 of all patients and 1/5 of all implants will be affected by peri‐implantitis [12]. Major risk factors associated with these epidemiological observations include past history of periodontitis, smoking, and diabetes, particularly in the absence of adequate oral hygiene [13–17]. Ill‐fitting or ill‐designed fixed and cement‐retained restorations are also evident factors of local plaque accumulation [12].
Early Peri‐implant Bone Loss and Associated Risk Factors
The process of wound healing following implant insertion is accompanied by bone remodeling that may eventually lead to a slight reduction of the marginal bone level and mild immunological reactions. As described further in this section, the process of early bone loss is multifactorial and involves intrinsic as well as extrinsic causes.
Stability of peri‐implant crestal bone (PICB) level is one of the pivotal issues that dictate both biological and esthetic long‐term success of dental implants [18]. In order to reach this goal clinicians should thoroughly understand the biological mechanisms of peri‐implant bone behavior to local and systemic factors associated with its remodelation as well as how to manage them.
Immediately after dental implant surgery, bone and soft tissue structures surrounding the implant site go through acute alterations. As the consequence of surgical intervention both submerged and non‐submerged implant placement procedures trigger wound healing and remodeling mechanisms in bone and mucosa that includes initiation and ultimately resolution of inflammation, matrix deposition, bone resorption/apposition, neurogenesis, and angiogenesis. This healing response is mediated by activated resident and migrating cells and related biological cascades involving cell–cell and cell–tissue interactions which are modulated by various cytokines, chemokines, and growth factors [19, 20].
Generally, 12 weeks after implant and/or healing abutment installation, integration of implant (osseointegration) at the bone‐implant interface and formation of attachment apparatus/biological seal at the soft tissue‐transmucosal part interface are almost entirely completed [20–23]. However, tissues, particularly peri‐implant crestal bone, continue to undergo dimensional changes, a phenomenon called “early/initial peri‐implant bone loss” (EPBL), which is generally accepted to continue up to one year following occlusal loading [24–26]. On marginal bone level changes around dental implants, EPBL indicates that the establishment of the implant integration with surrounding tissues to attain homeostasis is not a static state that is reached in several weeks following implant insertion, but rather a dynamic equilibrium process that may last more than a year. After one year of definitive prosthesis placement tissue, stability is expected. Minimal modeling of crestal bone around a successful implant of no more than 0.1–0.2 mm per year is favorable in the following periods of implant function [27, 28]. Cochran and colleagues have reported that the most significant amount of PICB which was independent from arch, type of restoration, or implant length, accounting for the 86% of the total bone loss in five years, occurred between the time of implant placement and the time that the final prosthesis was placed [29].
Peri‐implantitis is frequently defined as inflammation of the peri‐implant soft tissues associated with progressive marginal bone loss (Figure 4.1) [30]. The changes in PICB at early healing and maturation phases often occur as a consequence of physiological processes alone or in combination with individual adaptive response to intrinsic and extrinsic factors [26]. Since this reduction in peri‐implant bone level is not primarily of infectious origin and limited (<2 mm), it is believed to be a “condition” or a “complication” rather than a disease and quite distinct from peri‐implantitis‐related bone loss (Figure 4.2) [26, 31, 32]. EPBL may be indicative of a remodeling response and does not represent a clinical condition that needs therapeutic intervention. Therefore, it is pivotal to distinguish between limited early physiological bone remodeling and advancing PICB namely peri‐implantitis, with the latter implying a pathological state of infectious origin. According to “Statements from the Estepona Consensus Meeting on Peri‐Implantitis, 2012” it is not appropriate to name entire universe of peri‐implant bone losses as “peri‐implantitis” [33]. Therefore, to name “EPBL” as “peri‐implantitis” at the initial stage is not convenient; however, the scenario that peri‐implant tissue inflammation may occur due to plaque accumulation on previously exposed rough implant surface as a result of early bone loss and can serve as a “locus minoris resistensiae” for further progressive bone loss can not be entirely ruled out [25, 26, 34, 35]. This assumption of secondary infectious etiology may be questioned by some experts because presence of increased plaque accumulation and inflammation does not necessarily lead to peri‐implantitis and initially remodeled crestal bone may be maintained unchanged for prolonged periods in the absence of other risk factors [35–37]. There is a tendency to admit that bone resorption around dental implants beyond “early peri‐implant bone loss” which is within acceptable limits following the implants’ first year in function, ≥2 mm may represent a disease condition namely peri‐implantitis [38]. This type of additional marginal bone loss coupled with inflammation and profuse bleeding has been defined as a situation in which clinicians should be alerted and carefully monitored if the site needs therapeutic intervention [39].
Evidence also suggests that progressive PICB loss in the absence of mucosal inflammation is not likely to occur [30]. Also, inflammation may be present but may only be contained in the mucosal region farther from PICB which is in a steady equilibrium state thus limited EPBL may remain stable and may exhibit a non‐progressing character [35]. However, presence of combined potential risk factors that will result in disequilibrium of the host‐implant interaction such as smoking, history of periodontal disease, lack of proper plaque removal and maintenance may favor a secondary opportunistic microbial etiology in time and thus jeopardize the long‐term survival of the implants by extending the borders of the immune‐inflammatory response to crestal bone [32, 39, 40]. From this point of view, EPBL especially occurring at pre‐loading period may act as a predictor for future and advancing bone loss, leading to the development of peri‐implantitis and resulting in implant failure (Figure 4.3) [25, 41, 42]. Wandael et al. have reported that implant surface exposure as a consequence of EPBL exceeding 0.5‐ and 1‐mm threshold results in higher prevalence and odds ratio (four to six times higher) of peri‐implantitis and implant loss, especially in combination with smoking and/or a history of periodontitis. Non‐smokers presenting <5 mm EPBL showed the least probability of future peri‐implantitis [40]. Similarly, it has been shown that almost all implants with an 0.44 mm or more at 6 months after loading had shown progression in PICB loss and lost >2 mm marginal bone at 18 months post‐loading [43].

Figure 4.1 Peri‐implantitis refers to a biofilm‐driven pathological state that causes inflammation of the peri‐implant connective tissues, increased probing depths, and/or mucosal recession associated with progressive marginal bone loss. Whereas distal implants show peri‐implantitis and associated clinical and radiological findings, mesial aspects of the mesial implants remain otherwise unaffected.

Figure 4.2 PICB alterations during early stages of healing and maturation are often consequences of adaptive responses to intrinsic and extrinsic factors. The above case showing a limited bone loss (<2 mm) of non‐infectious cause is to be distinguished from peri‐implantitis‐driven PICB osteolysis. EPBL may represent a remodeling response that does not require therapeutic intervention. Therefore, it is indicative of a “condition” or “complication” rather than a “pathological state” or a “disease”. Following submerged and subcrestal placement (top left), uncovering implants and connecting healing abutments (top right, bottom left), slight radiological PICB loss and exposure of the implant neck six months following functional loading is not accompanied by inflammation, bleeding, or increased probing depths (bottom middle and right).
Although earlier literature has reported up to 1.5–2 mm of early bone loss to be within the limits of the acceptable thresholds, parallel to contemporary implant technology and increasing esthetic demands clinicians expect to maintain crestal bone for prolonged periods and to minimize or prevent PICB loss occurring at early periods of implant function to ensure the longevity of implants. EPBL is a multifactorial entity depending on factors related to patients, implant design, surgical protocols, and restorative protocols which are generally manageable or avoidable (Figure 4.4) [42, 44, 45]. In the following parts, these factors and recommended strategies to maintain the stability of peri‐implant tissues will be discussed in detail.
Factors Related to Early Peri‐implant Bone Loss
Several factors associated with EPBL should be taken into consideration from the planning phase to the loading stage of the dental implant rehabilitation in order to keep the PICB loss rate at minimum.

Figure 4.3 Schematic representation of early peri‐implant bone loss and putative mechanism underlying the predisposition to peri‐implantitis. MBL, marginal bone loss.

Figure 4.4 Factors that may result in early bone loss and that thereby may favor further biofilm accumulation may ultimately predispose to peri‐implantitis. Adverse effects of these factors can be mitigated by an appropriate risk assessment/management of the patient, implant selection, and controlling surgical and restorative factors.
Patient
Patient‐related factors might be more effective in the long‐run since their potential effects last longer than the locally‐acting factors however clinicians might question their possible association with EPBL in order to assess the risk. Smoking is a well‐documented risk factor for oral and periodontal health. Although smoking strongly shifts the peri‐implant microbiome to a disease‐associated state even in healthy sites, smokers and non‐smokers may exhibit similar probing depth and inflammation scores [46, 47]. No convincing evidence has been reached to date for smoking as being a risk factor/indicator of peri‐implant bone loss [30]. Similarly, the association of peri‐implantitis with diabetes and patient genetics is somewhat unclear. In contrast to the above factors cause and effect relation between peri‐implantitis and history of periodontitis, poor plaque control and lack of regular maintenance therapy is robust [30]. The relation between peri‐implantitis and cardiovascular disease, rheumatoid arthritis, osteoporosis, thyroid disorders, hepatitis, radiation therapy, chemotherapy, body mass index, hyperlipidemia, obesity, vitamin deficiency, serotonin reuptake inhibitors, and proton pump inhibitors present limited evidence and needs to be further cleared [21, 30].
Patients should be instructed thoroughly about the proper post‐operative care to avoid flap dehiscence and infection‐related EPBL including protection of the surgical site from trauma borne by wearing dentures and chewing on hard foods, usage of anti‐inflammatory drugs and abstaining from consumption of hot beverages to minimize the tissue edema, gentle brushing in conjunction with application of antiseptic agents and antibiotic prescription when necessary.
Implant Design
Two‐piece implant systems mainly consist of an abutment interlocking with the fixture by screws and anti‐rotational geometry or friction only. Under high chewing load vectors, the interface between the two components shows elastic deformations (micromovement) and forms a void (micro‐gap) that opens and closes repeatedly [48]. This gap interconnects the inner part of the implant and peri‐implant tissues which may lead to transfer of the bacteria, bacterial toxins, and acidic compounds (microleakage) in close proximity to the peri‐implant bone where they may induce inflammatory bone loss [49]. Although none of the different contemporary IAC’s (integrated abutment crown) designs are free of gap and totally prevent leakage through the interface, conical (morse taper) connections are likely to present increased resistance to micromovements and reduced micro‐gap/microleakage formation compared both to external and non‐conical internal connections thus may be associated with reduced bone loss (Figure 4.5) [50–52]. All morse taper‐like connections form a narrower abutment diameter namely horizontal offset (platform switch‐PS) at the IAC due to internal cone structure. PS‐structured implants have been linked with reduced bone loss compared to non‐PS ones, but the association seems to be indirect [53]. Proposed mechanisms of actions for PS in decreasing bone loss are; (i) movement of the IAJ thus microleakage away from crestal bone, modification of the biological width by adding a horizontal component, and more desirable distribution of forces which may interfere with the integrity of bone‐implant contact at the crestal portion of the implant [42, 50].
Based on the design of the two‐piece implants the vertical position of micro‐gap relative to bone may vary. The gap is located at the mucosal margin for the “soft tissue level” implants, above the crest but below the mucosal margin for the “polished collar” implants, and at or below the bone crest for the “fully rough‐surfaced” implants [54]. Theoretically “soft tissue level” implants harbor the most coronal micro‐gap location, which is equimucosal; however, this design can not prevent bone loss in both early and late periods [55]. In addition, sub‐crestal positioning of transmucosal part of soft tissue level implants and polished collar implants should be avoided since this approach increases bone resorption possibly due to lack of appropriate distribution of occlusal stress associated with absence of threads, approximation of the micro‐gap to bone and increased possibility of submucosal cement remnants [55–57].

Figure 4.5 Abutment and fixture frequently interconnect by screws and geometrical structures in two‐piece implants to provide a tight and stable seal. Contemporary internal connections are widely preferred types of interfaces although, to some extent, all types of internal connections display microgaps. Conical internal connections are likely to present increased resistance to micromovements and reduced microgap/microleakage formation over non‐conical IAC’s. However, engineering quality of the implants incorporating a well‐designed IAC may be of greater importance than the type of IAC to minimize both technical and biological complications. Note the lack of crestal bone loss around an implant with an internal, non‐conical IAC after six years of loading.
Neck portion design of the implants have been long focused as a site where the majority of forces are transmitted and cortical crestal bone‐ implant‐connective tissue intersects. Closely placed tiny threads at the most coronal part (micro‐threads) of the implants and gradual decreasing of the thread width from implant apex to the neck are designs directed toward reducing the excess pressure at the neck portion. Also, laser‐microtextured implant design both at collar area and abutment have been introduced. Although rough‐collared implants present lower PICB loss compared to smooth‐collar ones, evidence is lacking on the addition of micro‐threads and laser micro‐texture to rough collar regarding PICB loss [58–60].
Surgical Protocols
Abundant vascularization of soft and hard tissues is critical for healing and remodeling of peri‐implant bone. Therefore, proper 3D positioning of the implants in bone relative to cortical plates and nearby teeth and/or implants is critical to keep the EPBL at minimum. Implants at the crestal portion often neighbor dense cortical bone with a limited blood supply, thereby making the bone to be more prone to necrosis when excessive insertion torque is applied during placement of implants especially those of large implant diameter [45]. Hence EPBL related to surgical trauma is observed more pronounced at sites where cortical bone proportion is much greater than the cancellous bone (Figure 4.6) [61]. In order to facilitate preparation of properly shaped implant osteotomy and to reduce strain on crestal bone, usage of adequate final, pre‐tap, and cortical drills according to the manufacturer’s recommendations are the practical solutions to overcome necrosis of dense bone. In addition, overheating of the bone should be avoided by copious irrigation to prevent bone damage. When implants are placed following osteotome‐assisted crestal sinus lift or crest splitting increased trauma to bone may be anticipated thus slight submerged placement might be encouraged (Figure 4.7). Novel strategies alternative to conventional drilling such as osseo‐densification and piezosurgery might offer some benefits. Biomaterial and biomechanical considerations to prevent risks in implant therapy [31, 62, 63].
Spontaneous exposure of cover screw is another issue associated with EPBL (Figure 4.8) [64]. To overcome this complication, principles for atraumatic surgical flap management from incision to suturing and correct vertical positioning of the implant should be strictly followed particularly when the mucosa is thin (Figure 4.9). Mucosal height determines whether the bone resorption will occur during the establishment of the biological width around the transmucosal portion of implants as evidenced by the presence of thick (≥3 mm) mucosa results in lower EPBL (Figure 4.10) [65]. Also, keratinized mucosa (KM) width <3 mm might be associated with EPBL [66]. Therefore, practitioners should be familiar with the techniques to augment the mucosa and to modify the implant site accordingly to reduce the bone loss related to mucosal height and KM insufficiency.

Figure 4.6 Early peri‐implant bone loss related to surgical trauma may be more noticeable at sites with dense cortical bone due to the limited blood supply. Therefore, large diameter implants may be a preferred choice at maxillary posterior sites, which bare abundant cancellous bone more frequently than at mandibular posterior sites. Radiographs of a 6 × 8 mm plateau design, press‐fit, locking‐taper implant immediately after placement (left), and uncovering surgeries (right).

Figure 4.7 Implant’s bed can be prepared with osteotoms in the maxilla in order to place an implant, which length would exceed the vertical bone height below the neighboring maxillary sinus (left). In case of insufficient bone width crest splitting may be applied. Because these techniques increase intra‐operative trauma, placing a slightly submerged implant may help compensating for post‐operative bone remodeling (right).

Figure 4.8 Traumatic surgical procedures may result in flap dehiscence, exposure of cover‐screws and eventually in early peri‐implant bone loss. The patient from the intra‐oral photograph was referred 10 days following implant insertion for correction of the soft tissue problem.

Figure 4.9 Following basic principles in incision, flap reflection, management, suturing, and tension‐free closure of the mucosal flap serves atraumatic implant surgery, allows sufficient vascularization, and predisposes to an uneventful and timely healing. Primary flap closure with 5.0 polypropylene interrupted, crisscross, and horizontal mattress sutures (top) and healed site at four weeks (bottom).
Restorative Protocols
Abutment height that is surprisingly seemed to not relate with mucosal thickness and space for biological width establishment is a pivotal determinant of EPBL since it may influence the vertical position of the crown margin and short and divergent abutments may exert excess pressure on surrounding bone [67–70]. Intermittent connection and then disconnection of the healing abutments during the preparation of the final prosthesis causes disruption and then reestablishment of the mucosal seal which then in turn may lead to EPBL [71, 72]. In this context, choice of definitive abutment placement instead of healing abutment may be a viable method [73, 74].
Planning and restorative principles should be carefully followed to decide the optimal timing of loading, to provide a suprastructure design that presents a natural restoration emergence profile angle, to prevent improper marginal fit and abutment seating, to form a concave configuration of the transmucosal component with a narrow abutment profile, to exclusively select the retaining method (cemented versus screwed) in the context of excess cement avoidance and to allow for fabricating a prosthesis with a hygienic contour (Figure 4.11) [75–80]. In addition, prosthetic factors associated with, material selection, occlusal relations, distribution of occlusal forces, crown to implant ratio should be analytically considered to prevent technical complications leading to loosening of the components and titanium particle release/metallic leakage and ultimately to inflammation‐associated EPBL [25, 31,81–83].

Figure 4.10 In order to avoid PICB loss related to the formation of the biological width following abutment placement, presence of at least 3 mm of mucosa thickness is critical. Implants surrounded with mucosa <3 mm of height experience crestal bone loss whereas implants with peri‐implant mucosal thickness ≥3 mm show no loss associated with the establishment of the biological width (BW) (purple arrow: Vertical dimension of BW = 3 mm).

Figure 4.11 Restorative and surgical principles are critical to the fabrication of prosthetic crowns that respect a natural restoration emergence angle (REA) to minimize EPBL. A REA <30° is favorable in terms of avoiding early peri‐implant bone loss. Stability of the crestal bone around a 4 × 9 mm, subcrestally‐placed, platform switch, conical connection, implant after three years (left) and seven years (right) of loading can be observed.
Radiologically‐detected EPBL is a multifactorial entity that constitutes one of the academical controversial issues of implantology. It should be underlined that early limited reductions in PICB are not indicative of peri‐implant disease. Each individual factor given above seems to exert a minimal effect on PICB loss however may be of clinical significance regarding long‐term success when several of them conjoins. To sum‐up, total precise engineering of the implants incorporating an IAC which presents a stable connection with biological benefits and a crestal module that allows appropriate distribution of the forces and tight tissue integrity may be critical in the longevity of implants. Additionally, since EPBL might be associated with surgical trauma utmost attempt should be given in order not to jeopardize the healing capacity and vascularization of peri‐implant tissues. Restorative protocols should be followed with the consideration of eliminating and controlling prosthesis‐related issues on PICB loss. Reducing the number of peri‐implant inflammatory situations by minimizing EPBL related to above factors may lower the incidence of PI. Therefore, implant practitioners are responsible to be competent in surgical and restorative interventions in the context of life‐long learning attitude, to work with well‐documented implant systems in which they have comprehensive and analytical knowledge, to perform adequate planning regarding aesthetics and restoration‐driven implantology, to properly perform the risk assessment of the patient and plan the maintenance schedule on the patient basis to reach the goal of “predictable and long‐term implant stability” (Figure 4.12).

Figure 4.12 Predictable and long‐term implant stability are paramount goals that should be considered in any surgical implant insertion. Appropriate training in surgical and restorative interventions, utilizing well‐documented implant systems, an adequate planning of aesthetics and restoration outcomes as well as a cautious assessment of the patient’s risks and a well‐understood maintenance plan are all decisive factors to avoid post‐operative complications such as early peri‐implant bone loss. MBL, marginal bone loss.
The Oral Environment and Microbial Ecology
The oral environment represents is a unique ecosystem, which is both spatially and ecologically diverse, as it is continuously bathed in saliva [84, 85], lined by shedding epithelia of various degrees of keratinization, and framed by the non‐shedding dental surfaces, whereas the gingival sulcus interface between teeth and the gingiva physiologically contains a nutrient‐rich, serum‐derived transudate [86]. Occasionally, dental implants will replace natural teeth, and their metallic surface will also constitute a non‐shedding surface in the oral cavity, with a peri‐implant crevice that is deeper than that of the natural gingival sulcus. These anatomical and spatial diversities generate ecological gradients of temperature, redox potential, pH, and nutrient availability, which differentially support growth of the residing oral microbiota [87]. The spatial gradients of these conditions determine the distinct distribution and composition of microbial communities among the different niches of the oral cavity [88], described as “biogeography” [89].
The Microbial Ecosystem of the Peri‐implant Niche
Dental implants offer a pristine, non‐shedding titanium surfaces onto which a salivary pellicle rapidly adsorbs, just like onto natural teeth, with nonetheless slight compositional variations (apparent absence of low‐molecular‐weight mucins and cystatins) [90]. The dynamics of bacterial colonization on implant surfaces are initially slower than on natural teeth [91], although the sequence and composition of early colonizing communities appear unaffected [92, 93]. We now understand that early colonizers adhere to implant surfaces within thirty minutes following implant insertion [94], and evolve toward the formation of organized biofilm communities in the next two weeks [91]. The composition of the microbial communities that have colonized the peri‐implant niche at this stage resemble those of healthy neighboring gingival sulci [95], but they are less diverse [96]. Typically, in times of peri‐implant health, the submucosal microbiota displays high relative abundances of Leptotrichia spp., Veillonella dispar, V. parvula, Fusobacterium spp., Neisseria spp., and Streptococcus spp. (including S. sanguinis, S. parasanguinis, S. gordonii), and to a lesser extent species of Rothia (R. aeria, R. dentocariosa) [97–99]. These species are all commensals and from an ecological standpoint, they mostly catabolize saccharides as nutrient source and thrive in aerobic environments, aside from Leptotrichia spp. that favors anaerobic milieus despite aerotolerant abilities [100]. Whereas microbial colonization and biofilm formation are completely normal processes compatible with peri‐implant health, ecological shifts may drive this microbial ecosystem into a state of “dysbiosis,” as described further in this chapter.
Peri‐implant Microbial Biofilms
Early work on the peri‐implant microbiota relied on anaerobic culture‐based techniques and phase contrast microscopy [101, 102]. Based on these early methodological observations, the established notion was that the healthy peri‐implant sub‐mucosa is populated by Gram‐positive cocci, non‐motile bacilli, and few Gram‐negative anaerobic species [103, 104
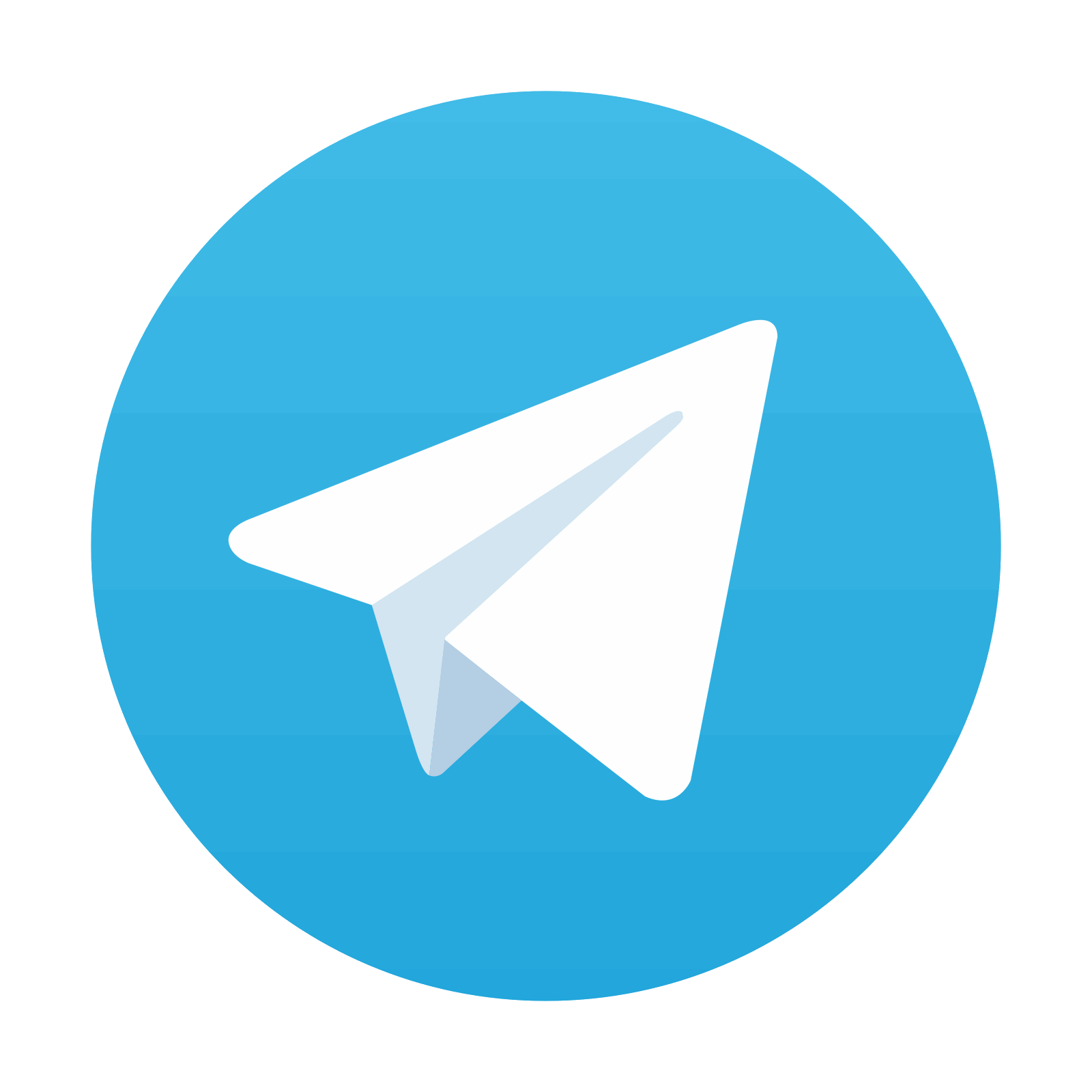
Stay updated, free dental videos. Join our Telegram channel

VIDEdental - Online dental courses
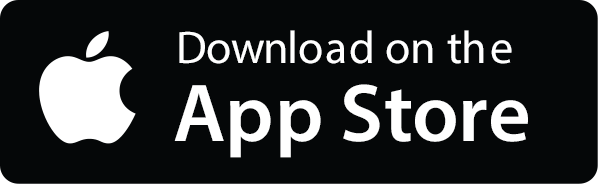
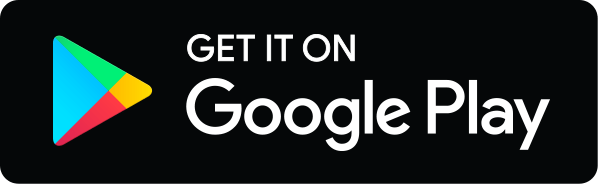