Highlights
- •
Collagen and collagenase both formed complexes with CSnp.
- •
Both native collagen-fibrils and dentin collagen after crosslinking showed higher resistance to collagenase degradation.
- •
CSnp crosslinking on collagen stabilized the ultrastructure and enhanced the resistance of dentin matrix.
Abstract
Antibacterial and chelating properties of chitosan has been widely studied for various dental applications.
Objective
To characterize the interaction between chitosan-nanoparticles (CSnp) and collagen, and understand their stabilizing effect against collagenase degradation for dentin matrix stabilization.
Methods
Phase-1: a single Type I collagen-fibril model was used to study the interaction with CSnp along with carbodiimides crosslinking treatment. Degradation of the crosslinked fibrils was studied with bacterial collagenase enzyme and monitored using Fourier Transform Infrared (FTIR) spectroscopy, turbidity measurement (400 nm), ninhydrin assay and Atomic Force Microscopy (AFM). Interaction of CSnp with collagenase and Type I collagen, were evaluated using SDS-PAGE, and proteolytic cleavage potential of a synthetic peptide. Phase-2: degradation of dentin collagen crosslinked with/without CSnp was evaluated using FTIR, ninhydrin assay and Scanning Electron Microscopy (SEM). Glutaraldehyde crosslinking was used as a positive control.
Results
Both native collagen-fibrils and dentin collagen after crosslinking showed higher resistance to collagenase degradation, as observed in turbidity measurements and FTIR spectra. AFM images showed the interaction of CSnp with single collagen-fibril and crosslinked collagen resisted collagenase degradation up to 54 h. The collagen and collagenase both formed complexes with CSnp resulting in thickening of bands and reduction in collagen degradation. CSnp treated collagenase showed significantly reduced cleavage of the fluorescent peptides. Dentin collagen was coated with CSnp following crosslinking with significant increase in resistance to collagenase degradation.
Significance
Crosslinked CSnp on collagen stabilized and enhanced the resistance of dentin matrix against bacterial collagenase degradation due to non-specific interaction with both collagen and collagenase.
1
Introduction
The structural framework of dentin that is provided by the inorganic and organic fraction, is crucial to maintain the mechanical integrity of teeth . Clinical observations have shown varying degrees of dentin collagen degradation depending upon the years of function . In addition, caustic chemicals such as sodium hypochlorite (NaOCl), chelating agent such as ethylenediamine tetra-acetic acid (EDTA), and high pH medicaments such as calcium hydroxide (Ca(OH) 2 ), when used for treatment purposes are known to induce surface changes on root dentin . These disease (non-iatrogenic) and treatment-mediated (iatrogenic) processes, results in compromised physical and mechanical characteristics of dentin, which increases the risk of fracture in root-filled teeth . Clinical studies have highlighted that approximately 11% of the extracted root-filled teeth show signs of vertical root fractures .
Type I collagen is the major structural protein comprising up to 90% of the organic fraction of dentin . Exposed collagen from dentin matrix following disease or iatrogenic procedures is susceptible to degradation. Proteases from bacteria, and saliva or host derived matrix metalloproteinases are known to degrade dentin collagen with time . Degradation of dentin occurs due to the dissolution or denaturation of the organic proteins and proteoglycan molecules, which bonds the collagen network with the hydroxyapatite crystals in dentin . The degradation of collagen on root canal dentin surface may lead to interfacial failures at the restoration–dentin interface, leading to microbial penetration and restorative failures in root filled teeth . Any treatment attempt to stabilize the ultrastructure of dentin collagen and neutralize the effect of bacterial collagenase on dentin collagen will significantly improve the mechanical integrity of root filled teeth . Thus, crosslinking has been applied to improve the mechanical and biological stability of dentin collagen .
Crosslinking methods such as chemical, physical or photocrosslinking will induce intra- and intermolecular crosslinks in collagen, and have been successfully used in tissue engineering to stabilize biological tissues and artificial constructs . Glutaraldehyde has been shown as an effective crosslinking agent to increase the tensile properties and stiffness of demineralized dentin . However, glutaraldehyde is highly cytotoxic due to the reaction by-products, limiting their application in vivo . Another chemical crosslinker, 1-ethyl-3-(3-dimethylaminopropyl) carbodiimide (EDC) in the presence of N-hydroxysuccinimide (NHS) are known to stabilize collagen by the formation of additional crosslinks . They are non-toxic, leave no residues following treatment and forms amide-type crosslinks with collagen . A recent study has demonstrated increased resistance to fatigue cracks growth in resin–dentin interface following carbodiimide conditioning even after aging for 6 months in artificial saliva . These crosslinking techniques have also been employed to incorporate natural biopolymers into collagen scaffold. These crosslinked biopolymers would act as fillers that further enhanced the mechanical characteristics of the scaffold .
Chitosan is a hydrophilic biopolymer (2-amino-2-deoxy-β- d -glucopyranose) with large number of free hydroxyl and amino groups that can form crosslinks with other reactive molecules. The free reactive groups in chitosan can interact to form chemical bonds with collagen . The chitosan incorporated and crosslinked dentin collagen displayed superior mechanical properties as compared to crosslinking alone, as well as resisted degradation by bacterial collagenase . In addition, chitosan nanoparticles exhibited excellent antibiofilm properties and also inhibited bacterial adherence on dentin . In spite of all these interests, the specific role of chitosan-nanoparticles (CSnp) in combination with chemical crosslinking to stabilize collagen ultrastructure and its ability to inhibit bacterial collagenolytic activity has not been fully characterized. Crosslinking CSnp on dentin collagen in situ, prior to endodontic procedures or adhesive restorations could provide several advantages such as resistance to interfacial degradation, prevent bacterial recolonization at the interface and improve mechanical properties of dentin matrix.
The purpose of this study was twofold: (1) to characterize the interaction and stabilizing effect of CSnp crosslinking on type I collagen and effect of CSnp on collagenase enzyme activity. (2) To study the effect of CSnp crosslinking on dentin collagen towards bacterial collagenase degradation. The null hypothesis is that CSnp crosslinking does not have any effect on the degradation of Type I collagen.
2
Materials and methods
All the chemicals were purchased from Sigma Aldrich (St. Louis, USA) unless mentioned otherwise. CSnp was synthesized based on a previously published protocol . Bovine type I collagen (Purecol, 3 mg/mL) fibrils were assembled in 200 mM sodium phosphate buffer (pH 7.3) . Collagenase from clostridium histolyticum with an activity of 125 CDU/mg solid (P/N C-0130) was prepared in 50 mM HEPES buffer (pH 7.4) containing 0.36 mM CaCl 2 . EDC and NHS were used for chemical crosslinking .
2.1
Native type I collagen fibril model
2.1.1
Effect of crosslinking and CSnp treatment on collagen
Native collagen fibrils were synthesized as described in the literature . Briefly, a reaction buffer containing 150 μL of water, 500 μL of 200 mM Na 2 HPO 4 and 250 μL of 400 mM KCl was prepared and 100 μL collagen monomer was added. The reaction mixture was incubated at 37 °C. Once the clear and colorless solution turned cloudy, native collagen fibrils were collected by centrifugation and taken for degradation study.
Collagen specimens were divided into five treatment groups ( n = 6): Group-1: Control (No treatment); Group-2: glutaraldehyde: Group-3: EDC/NHS; Group-4: CSnp + EDC/NHS and Group-5: CSnp. The collagen fibrils were subjected to crosslinking for 12 h with 100 μL of one of the following: glutaraldehyde (2.5%), and CSnp (0.3 mg/mL). After crosslinking, the collagen fibrils were gently washed 3 times with deionized-water. For EDC/NHS containing groups, collagen fibrils were immersed into 40% (v/v) ethanol containing 50 mM 2-morpholinoethane sulfonic acid (MES) buffer (pH 5.0), 33 mM EDC and 8 mM NHS with/without CSnp (0.3 mg/mL). After crosslinking, the collagen fibrils were neutralized with 0.1 M Na 2 HPO 4 (pH 9.1) for 1 h, followed by repeated washing with 40% ethanol and deionized-water. All the collagen fibrils were stored at 4 °C until further use. The collagen fibrils on the mica sheets were also subjected to similar treatments ( n = 3).
2.1.2
Degradation analysis: chemical, spectroscopic and topographical analysis
The native collagen degradation rate for the following treatment groups was evaluated using three different methods: (1) turbidity measurement (to determine the rate of collagen degradation), (2) ninhydrin assay (amino acid release following degradation) and (3) Fourier transform infrared (FTIR)-Attenuated Total Reflectance (ATR) spectroscopy. AFM was used to monitor changes in the nano-topography of collagen fibril during degradation.
Pellets of cross-linked collagen specimens were spread uniformly covering the bottom of the 96-well plate. Collagenase (0.3 mg/mL) was added and turbidity at 400 nm was measured at 37 °C every 5 minutes interval for 100 minutes using a microplate reader (InfiniteH M1000, Tecan, Mannedorf, Switzerland). The supernatant of collagen samples after 2 h collagenase treatment was used for Ninhydrin assay ( n = 3) to quantify the amino acid released as described in the literature . The chemical analysis following crosslinking and degradation on collagen fibrils was determined by FTIR-ATR spectroscopy. The spectra of crosslinked collagen were obtained under hydrated condition using a FTIR spectrometer (Spectrum One, Perkin-Elmer, Waltham, MA) at a resolution of 4 cm −1 by applying a thin film of collagen directly on the sensing window of ATR accessory (Perkin Elmer, Universal ATR sample accessories).
Atomic force microscopy (AFM) analysis was carried out on a single collagen fibril. For the single collagen model, collagen solution (50 μl) was deposited on a freshly cleaved mica sheet bonded to a glass slide and dried under a gentle stream of nitrogen gas prior to AFM imaging . The collagen specimens exposed to collagenase solution for pre-determined time were observed in situ in tapping mode with a Nanowizard II AFM (JPK instruments, Germany), using NP-type silicon nitride tips (Digital Instruments, Santa Barbara, CA). Tips with a nominal spring constant of 0.58 N/m and a tip radius of approximately 10 nm were used. One single fibril was imaged before and after various crosslinking steps and subsequently after degradation process. The scan rate of 1 Hz, scan size of 10 × 10 μm 2 and a resolution of 512 × 512 pixels was used to image minimum of two collagen fibrils per view. The image was subsequently zoomed in to a scan size of 2 × 2 μm 2 to observe the banding periodicity of the collagen. Height and amplitude images were recorded simultaneously. Amplitude mode was used to evaluate the topography of the specimens and height mode images were used to obtain quantitative measurements. Three samples were used for each group.
2.1.3
Interaction of CSnp with collagenase
Collagenase from Clostridium histolyticum (250 μg/mL and 50 μg/mL) was incubated with CSnp (1 mg/mL and 0.3 mg/mL) for 2 h, 1 h and 30 min at 37 °C. The mixture was centrifuged (14,000 rpm for 10 min) and the pellets were suspended in 10 mM Tris–HCl (pH 7.4). 10 μL of the solution was used to assess the remaining collagenase activity using an MMP-13 fluorogenic substrate (2.5 μM) (Mca-Pro-Cha-Gly-Nva-His-Ala-Dpa-NH 2 ; SIGMA) in 10 mM Tris–HCl (pH 7.4), 2 mM CaCl 2 , and 0.01% Brij 35 substrate buffer. Fluorescence readings were recorded as specified by the manufacturer (excitation 325 nm/emission 393 nm). Collagenase incubated with chitosan without centrifugation was also used as a control in this experiment.
SDS-PAGE was used to analyze the changes in the collagen and collagenase molecular structure following interaction with CSnp. Degradation of collagen by collagenase following interaction of either collagen or collagenase with CSnp at 2 h, 1 h and 30 min was evaluated. CSnp were dispersed in deionized-water at concentrations 0.3 or 1 mg/mL. The CSnp, collagen and collagenase solution was centrifuged; supernatant was taken and 50 μL of the solution was subjected to SDS-PAGE analysis using 10% acrylamide gel following initial characterization experiments. The bands were visualized by Coomassie Brilliant Blue R-250 staining and analyzed by Bio-Rad imaging system (Bio-Rad, Hercules, CA). Prestained protein ladder was used for size determination. The resistance of collagen to degradation following interaction with CSnp was evaluated on the basis of appearance or absence of α1 and α2 bands in SDS-PAGE .
2.2
Dentin collagen model
2.2.1
Effect of crosslinking and CSnp treatment on dentin collagen
Freshly extracted caries-free human incisors were collected for the study following University ethical guidelines committee (#26363). The teeth were stored in PBS at 4 °C until use. Dentin sections of 0.5 mm thickness were prepared from either side of the root canal using a slow speed diamond-wafering blade (Buehler, Coventry, UK) under continuous water irrigation . The sections were further ground into dimensions of 8 mm × 5 mm × 0.5 mm using wet emery paper of grit sizes 400, 800 and 4000 under continuous water irrigation. These dentin sections were treated with 5.2% NaOCl (20 min) and 17% EDTA (2 min), to simulate clinical practice . Following chemical treatments, the dentin sections were thoroughly rinsed with water and stored in sterile deionized-water at 4 °C before testing. Dentin collagen specimens ( N = 24) were divided into four groups: Group-1: Control (No treatment); Group-2: glutaraldehyde (2.5%); Group-3: EDC/NHS; and Group-4: CSnp (0.3 mg/mL) + EDC/NHS. The dentin collagen specimens were subjected to crosslinking as in case of native collagen fibrils for 12 h.
2.2.2
Degradation analysis: spectroscopic and morphological analysis
All crosslinked and control dentin collagen specimens were degraded with collagenase solution (100 μg/mL) for different time periods until 15 days at 37 °C. The supernatant at each time point was collected for Ninhydrin assay to quantify the amino acid released following enzymatic degradation. In addition to the amino acid analysis, the post dentin collagen degradation (15 days) specimens ( n = 3) were examined using Scanning Electron Microscopy (SEM) (Hitachi S-2500, Tokyo, Japan). Towards SEM analysis, the specimens were washed with deionized-water and serially dehydrated using ascending series of aqueous ethanol solution of increasing concentration. Final dehydration was carried out using increasing concentration of hexamethyldisilazane in ethanol. The processed specimens were sputter-coated with carbon for 10 s prior to microscopic examination. The remaining three specimens in each group were stored in a vacuum desiccator overnight, characterized using FTIR-ATR technique.
2.3
Statistical analysis
The data was analyzed by ANOVA and Tukey–Kramer’s test for intergroup variance. For AFM data, the average from three measurements were calculated and subjected to t -test (2 tailed). Significance levels was set at p ≤ 0.05.
2
Materials and methods
All the chemicals were purchased from Sigma Aldrich (St. Louis, USA) unless mentioned otherwise. CSnp was synthesized based on a previously published protocol . Bovine type I collagen (Purecol, 3 mg/mL) fibrils were assembled in 200 mM sodium phosphate buffer (pH 7.3) . Collagenase from clostridium histolyticum with an activity of 125 CDU/mg solid (P/N C-0130) was prepared in 50 mM HEPES buffer (pH 7.4) containing 0.36 mM CaCl 2 . EDC and NHS were used for chemical crosslinking .
2.1
Native type I collagen fibril model
2.1.1
Effect of crosslinking and CSnp treatment on collagen
Native collagen fibrils were synthesized as described in the literature . Briefly, a reaction buffer containing 150 μL of water, 500 μL of 200 mM Na 2 HPO 4 and 250 μL of 400 mM KCl was prepared and 100 μL collagen monomer was added. The reaction mixture was incubated at 37 °C. Once the clear and colorless solution turned cloudy, native collagen fibrils were collected by centrifugation and taken for degradation study.
Collagen specimens were divided into five treatment groups ( n = 6): Group-1: Control (No treatment); Group-2: glutaraldehyde: Group-3: EDC/NHS; Group-4: CSnp + EDC/NHS and Group-5: CSnp. The collagen fibrils were subjected to crosslinking for 12 h with 100 μL of one of the following: glutaraldehyde (2.5%), and CSnp (0.3 mg/mL). After crosslinking, the collagen fibrils were gently washed 3 times with deionized-water. For EDC/NHS containing groups, collagen fibrils were immersed into 40% (v/v) ethanol containing 50 mM 2-morpholinoethane sulfonic acid (MES) buffer (pH 5.0), 33 mM EDC and 8 mM NHS with/without CSnp (0.3 mg/mL). After crosslinking, the collagen fibrils were neutralized with 0.1 M Na 2 HPO 4 (pH 9.1) for 1 h, followed by repeated washing with 40% ethanol and deionized-water. All the collagen fibrils were stored at 4 °C until further use. The collagen fibrils on the mica sheets were also subjected to similar treatments ( n = 3).
2.1.2
Degradation analysis: chemical, spectroscopic and topographical analysis
The native collagen degradation rate for the following treatment groups was evaluated using three different methods: (1) turbidity measurement (to determine the rate of collagen degradation), (2) ninhydrin assay (amino acid release following degradation) and (3) Fourier transform infrared (FTIR)-Attenuated Total Reflectance (ATR) spectroscopy. AFM was used to monitor changes in the nano-topography of collagen fibril during degradation.
Pellets of cross-linked collagen specimens were spread uniformly covering the bottom of the 96-well plate. Collagenase (0.3 mg/mL) was added and turbidity at 400 nm was measured at 37 °C every 5 minutes interval for 100 minutes using a microplate reader (InfiniteH M1000, Tecan, Mannedorf, Switzerland). The supernatant of collagen samples after 2 h collagenase treatment was used for Ninhydrin assay ( n = 3) to quantify the amino acid released as described in the literature . The chemical analysis following crosslinking and degradation on collagen fibrils was determined by FTIR-ATR spectroscopy. The spectra of crosslinked collagen were obtained under hydrated condition using a FTIR spectrometer (Spectrum One, Perkin-Elmer, Waltham, MA) at a resolution of 4 cm −1 by applying a thin film of collagen directly on the sensing window of ATR accessory (Perkin Elmer, Universal ATR sample accessories).
Atomic force microscopy (AFM) analysis was carried out on a single collagen fibril. For the single collagen model, collagen solution (50 μl) was deposited on a freshly cleaved mica sheet bonded to a glass slide and dried under a gentle stream of nitrogen gas prior to AFM imaging . The collagen specimens exposed to collagenase solution for pre-determined time were observed in situ in tapping mode with a Nanowizard II AFM (JPK instruments, Germany), using NP-type silicon nitride tips (Digital Instruments, Santa Barbara, CA). Tips with a nominal spring constant of 0.58 N/m and a tip radius of approximately 10 nm were used. One single fibril was imaged before and after various crosslinking steps and subsequently after degradation process. The scan rate of 1 Hz, scan size of 10 × 10 μm 2 and a resolution of 512 × 512 pixels was used to image minimum of two collagen fibrils per view. The image was subsequently zoomed in to a scan size of 2 × 2 μm 2 to observe the banding periodicity of the collagen. Height and amplitude images were recorded simultaneously. Amplitude mode was used to evaluate the topography of the specimens and height mode images were used to obtain quantitative measurements. Three samples were used for each group.
2.1.3
Interaction of CSnp with collagenase
Collagenase from Clostridium histolyticum (250 μg/mL and 50 μg/mL) was incubated with CSnp (1 mg/mL and 0.3 mg/mL) for 2 h, 1 h and 30 min at 37 °C. The mixture was centrifuged (14,000 rpm for 10 min) and the pellets were suspended in 10 mM Tris–HCl (pH 7.4). 10 μL of the solution was used to assess the remaining collagenase activity using an MMP-13 fluorogenic substrate (2.5 μM) (Mca-Pro-Cha-Gly-Nva-His-Ala-Dpa-NH 2 ; SIGMA) in 10 mM Tris–HCl (pH 7.4), 2 mM CaCl 2 , and 0.01% Brij 35 substrate buffer. Fluorescence readings were recorded as specified by the manufacturer (excitation 325 nm/emission 393 nm). Collagenase incubated with chitosan without centrifugation was also used as a control in this experiment.
SDS-PAGE was used to analyze the changes in the collagen and collagenase molecular structure following interaction with CSnp. Degradation of collagen by collagenase following interaction of either collagen or collagenase with CSnp at 2 h, 1 h and 30 min was evaluated. CSnp were dispersed in deionized-water at concentrations 0.3 or 1 mg/mL. The CSnp, collagen and collagenase solution was centrifuged; supernatant was taken and 50 μL of the solution was subjected to SDS-PAGE analysis using 10% acrylamide gel following initial characterization experiments. The bands were visualized by Coomassie Brilliant Blue R-250 staining and analyzed by Bio-Rad imaging system (Bio-Rad, Hercules, CA). Prestained protein ladder was used for size determination. The resistance of collagen to degradation following interaction with CSnp was evaluated on the basis of appearance or absence of α1 and α2 bands in SDS-PAGE .
2.2
Dentin collagen model
2.2.1
Effect of crosslinking and CSnp treatment on dentin collagen
Freshly extracted caries-free human incisors were collected for the study following University ethical guidelines committee (#26363). The teeth were stored in PBS at 4 °C until use. Dentin sections of 0.5 mm thickness were prepared from either side of the root canal using a slow speed diamond-wafering blade (Buehler, Coventry, UK) under continuous water irrigation . The sections were further ground into dimensions of 8 mm × 5 mm × 0.5 mm using wet emery paper of grit sizes 400, 800 and 4000 under continuous water irrigation. These dentin sections were treated with 5.2% NaOCl (20 min) and 17% EDTA (2 min), to simulate clinical practice . Following chemical treatments, the dentin sections were thoroughly rinsed with water and stored in sterile deionized-water at 4 °C before testing. Dentin collagen specimens ( N = 24) were divided into four groups: Group-1: Control (No treatment); Group-2: glutaraldehyde (2.5%); Group-3: EDC/NHS; and Group-4: CSnp (0.3 mg/mL) + EDC/NHS. The dentin collagen specimens were subjected to crosslinking as in case of native collagen fibrils for 12 h.
2.2.2
Degradation analysis: spectroscopic and morphological analysis
All crosslinked and control dentin collagen specimens were degraded with collagenase solution (100 μg/mL) for different time periods until 15 days at 37 °C. The supernatant at each time point was collected for Ninhydrin assay to quantify the amino acid released following enzymatic degradation. In addition to the amino acid analysis, the post dentin collagen degradation (15 days) specimens ( n = 3) were examined using Scanning Electron Microscopy (SEM) (Hitachi S-2500, Tokyo, Japan). Towards SEM analysis, the specimens were washed with deionized-water and serially dehydrated using ascending series of aqueous ethanol solution of increasing concentration. Final dehydration was carried out using increasing concentration of hexamethyldisilazane in ethanol. The processed specimens were sputter-coated with carbon for 10 s prior to microscopic examination. The remaining three specimens in each group were stored in a vacuum desiccator overnight, characterized using FTIR-ATR technique.
2.3
Statistical analysis
The data was analyzed by ANOVA and Tukey–Kramer’s test for intergroup variance. For AFM data, the average from three measurements were calculated and subjected to t -test (2 tailed). Significance levels was set at p ≤ 0.05.
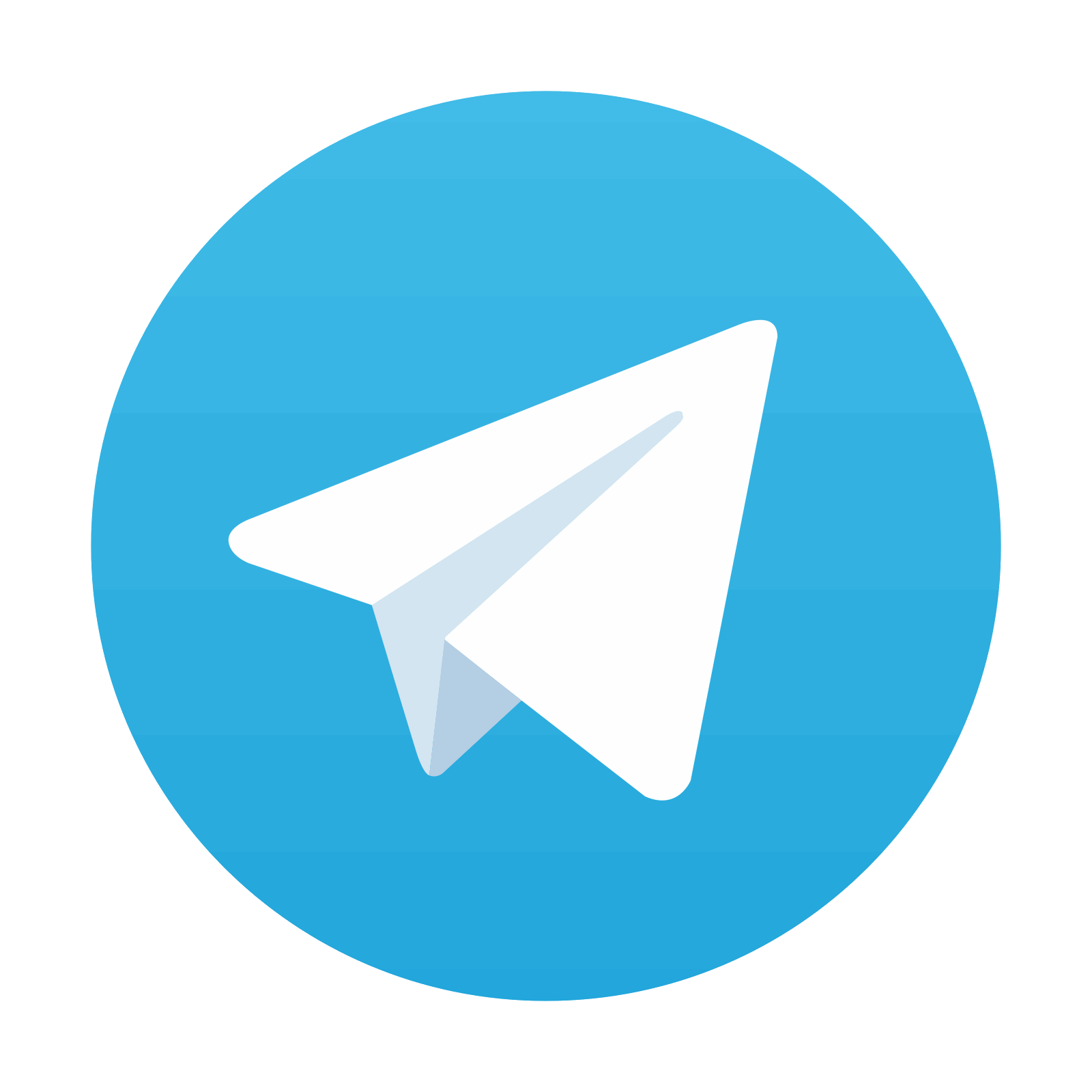
Stay updated, free dental videos. Join our Telegram channel

VIDEdental - Online dental courses
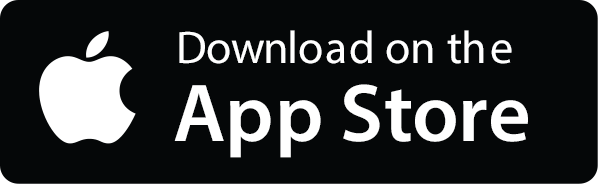
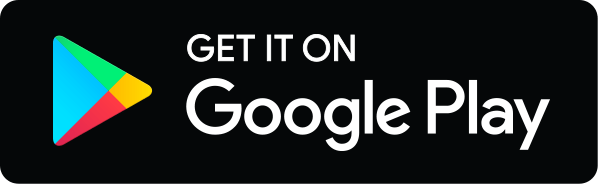