26
Ceramic Implants
Joan Pi Anfruns
Private Practice, Los Angeles, CA, USA and
Department of Oral and Maxillofacial Surgery, UCLA School of Dentistry, Los Angeles, CA, USA
Introduction
Without a doubt, titanium dental implants have been considered the gold standard for tooth replacement since their introduction in the 1960s, demonstrating excellent biocompatibility and offering a wide range of treatment options for patients. Nonetheless, reports on possible unwanted reactions to titanium have increased in recent years. Hypersensitivity and allergic reactions, corrosion, Ti particle release, and unfavorable esthetic results have been reported [1–4]. In addition, the emerging trend of a holistic or metal‐free approach to dentistry has prompted patients and clinicians to seek for an alternative to titanium and other metals.
The quest for an alternative to metal implants dates back to the 1930s, when M. Rock applied for a patent on Aluminum Oxide. In 1965, Prof. Sami Sandhaus, considered the father of ceramic implantology, patented a screw‐shaped dental implant made of alumina powder (Degussit AL23). This would be the first step in a new era in ceramic implantology [5]. Sandhaus continued his investigations with aluminum oxide and introduced the Crystalline Bone Screw (C.B.S) in 1968, with a reported success rate of only 25% after five years [6]. Sandhaus would later commercialize these implants under the brand name CeraSand in Switzerland, but no long‐term data exists on their performance [7]. These efforts were followed by Schulte and Heimke in 1976, who introduced the Tübingen implant (Frialit I; Friadent, Mannheim, Germany) for immediate implant placement in the maxillary anterior region (Figure 26.1). A success rate of 92.5% was reported after an investigation period of 10 years [8]. The Bionit system, another aluminum oxide implant, was introduced by Müller and co‐workers in the late 1980s in Germany [9], but little data exists regarding their long‐term behavior. In conjunction with the aforementioned efforts with aluminum oxide, single crystal alumina (Sapphire) implants were also investigated as a means for tooth replacement. The BioCeram implant, developed by Kyocera (Kyoto, Japan) and introduced in the 1970s was perhaps the most documented of them all (Figures 26.2 and 26.3). Steflik et al. [10] reported 10‐year results with 81% success rates for tooth–implant–supported fixed partial dentures. Fartash and Arvidson [11] reported on the treatment of total or partial edentulism with fixed prosthesis with a follow‐up of 10 years, demonstrating a survival rate of 100% of their tooth‐implant restorations. The same group later reported only implant–supported fixed partial dentures, with an estimated survival rate of 96.6%. The Bioceram implant system was also utilized for the treatment of complete edentulism. Berge and Gronningsaeter [12] reported on 30 patients who had been treated with implant‐assisted mandibular overdentures, with a cumulative survival rate of 69% over a period of 11 years. All of the above systems were eventually removed from the market due to poor mechanical properties and unfavorable long‐term clinical performance [13].
Zirconia
Introduced in the 1990s as a dental material, its current clinical applications range from posts, frameworks, and abutments to dental implants. Zirconia is white, has excellent biocompatibility, low affinity to plaque, and is able to achieve osseointegration levels comparable to its titanium counterparts [4, 13].

Figure 26.1 The Tübingen implant.
Source: SpotImplant.


Figures 26.2–26.3 The BioCeram implants, developed by Kyocera (Kyoto, Japan). Available in blade and root form.
Source: KYOCERA Corporation.
Manufacturing
Zircon is the raw material by which Zirconia is obtained. A mineral belonging to the group of neosilicates, its chemical name is zirconium silicate (ZrSiO4), and is a source of the metal zirconium (Zr) and the ceramic Zirconia (ZrO2). About 80% of the world’s Zircon mining occurs in Australia and South Africa. To obtain the Zr metal, the material is separated via the Van Arkel process. The result is a zirconium (Zr) sponge called the Zirconia Crystal Bar, which can be melted to obtain zirconium rods. Pure zirconium is used in nuclear reactors as containers of nuclear fuel and in combination with other metals to form compounds. Once the metal is obtained, it can undergo a calcination process, by which an oxide is produced. The result is zirconium dioxide or Zirconia (ZrO2), a ceramic with no metallic properties. Zirconia occurs in three temperature‐dependent forms: monoclinic (room temperature to 1170 °C), tetragonal (1170 °C–2370 °C), and cubic (2370 °C – up to melting point) [14].
Zirconia enters the monoclinic phase after the calcination process of zirconium. At this stage, the material is very fragile and requires stabilization with other compounds to enter the tetragonal phase and make it suitable for dental applications. Ytria and Alumina are most commonly utilized to stabilize zirconia. Zirconia dental implants today are manufactured either with Y‐TZP (Ytria‐stabilized Tetragonal Zirconia Policrystals) or ATZ (Alumina‐Toughened Zirconia). The manufacturing process occurs via subtractive milling. A zirconia blank is milled to achieve its final macro and microscopic characteristics. This blank is then subjected to a sintering process and Hot Isostatic Pressing (HIP), where a controlled shrinkage occurs by about 15–20%. The implants are then post‐processed to achieve the final surface characteristics via sandblasting, acid etching, laser treatment, or a combination of those.
Advancements in manufacturing technology such as the introduction of injection molding for the production of zirconia implants have streamlined the production processes. Injection molding is a novel technology that eliminates the post‐processing procedures that take place after sintering. The macro, micro, and nano‐characteristics of the implants are all incorporated into a pre‐designed mold. The raw material is a ceramic feedstock that is injected at high pressure (2500 PSI) into the molds and then sintered at high temperature for a controlled shrinkage. Since there is no post‐processing via sandblasting/acid etching, this process yields an impurity‐free surface. Injection molding also provides a reduction in the manufacturing costs, tool wear, and lowers the environmental impact of the manufacturing process (Figure 26.4).
Commercially available Zirconia implants can be one or two‐piece. One‐piece zirconia implants with an integrated abutment and pre‐determined restorative margin have limitations due to their inability to allow for angle correction, require a high degree of surgical precision, and only accept a cementable restoration. Two‐piece systems with either cement or screw‐retained abutments allow clinicians to broaden their prosthetic options, including angle correction via angled abutments, the ability to deliver screw‐retained restorations, as well as improved options for soft tissue management. Commercially available two‐piece systems can accept either a cementable or screw‐retained abutment. Spies and coworkers [15] compared the fracture resistance of cement and screw‐retained systems with one‐piece implants. Despite the fact that one‐piece implants outperformed the two‐piece systems, the bending moment values for the two‐piece system remained above the maximum values measured in the mouth. The authors concluded that the fracture‐resistance values of two‐piece systems could withstand physiological bite forces in vivo. The stability of screw retention in two‐piece systems was further investigated by Joos et al. [16]. Two‐piece zirconia and titanium implants were subjected to artificial aging, including thermal cycling and chewing simulation/mechanical loading equivalent to five years of function. Fracture tests resulted in mean forces well above physiologic thresholds and the stability of the connection between the restoration and the implant seemed comparable for both zirconia and titanium implants, making them suitable for clinical application (Figures 26.5–26.12).

Figure 26.4 One and two‐piece zirconia implants manufactured via Ceramic Injection Molding. The macro, micro, and nano‐ characteristics are incorporated into the mold.
Source: Zinova Zirconia Implant System.
Osseointegration
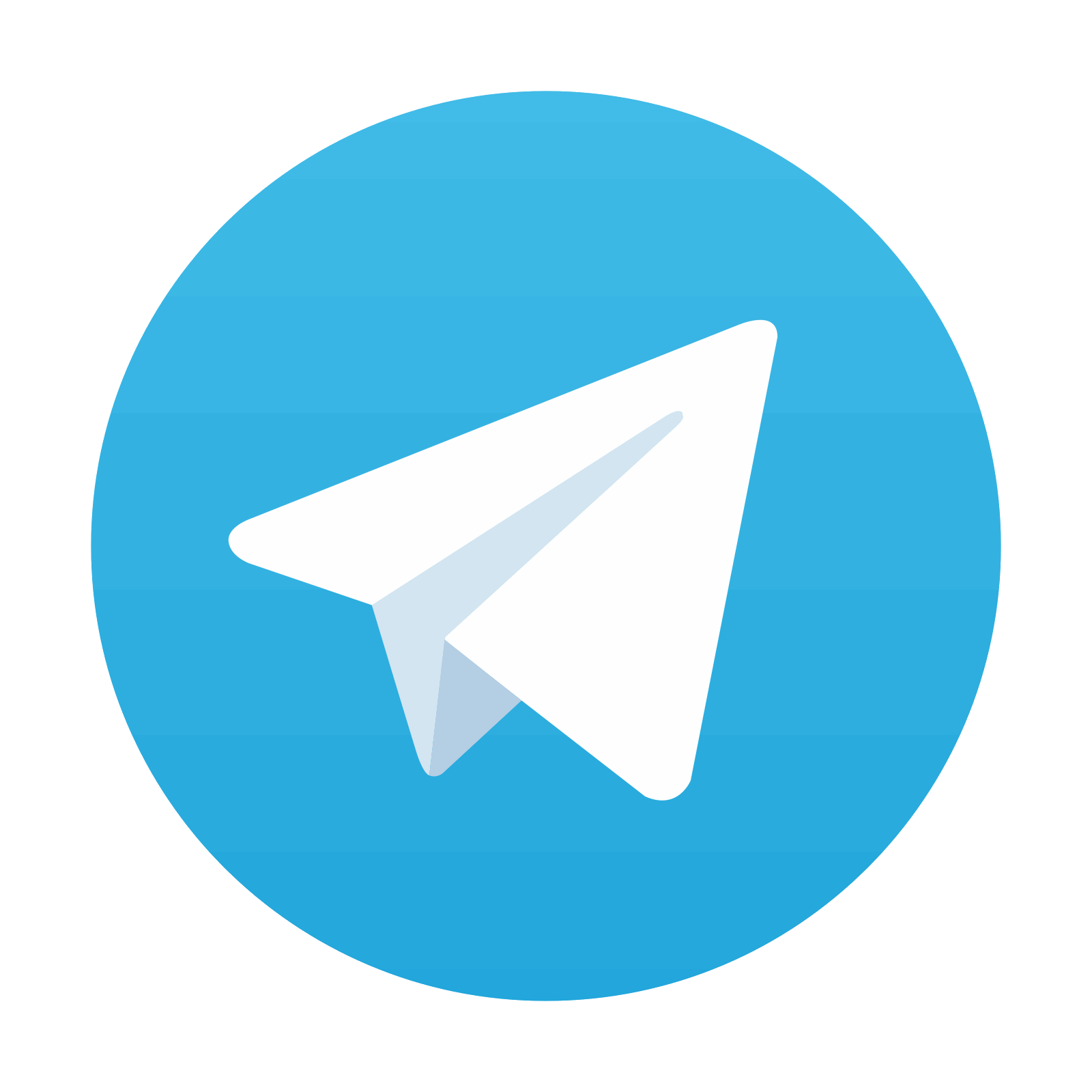
Stay updated, free dental videos. Join our Telegram channel

VIDEdental - Online dental courses
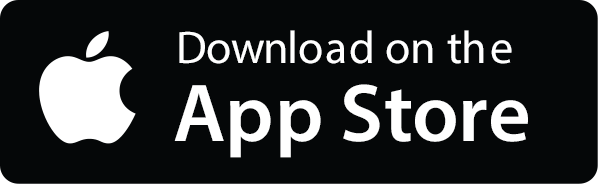
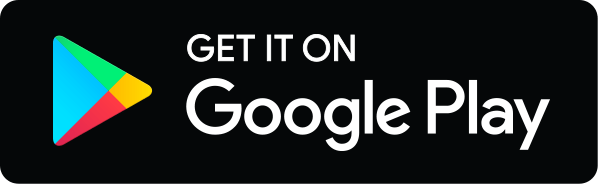