Abstract
Objective
Restorative materials exhibit contraction and expansion due to polymerization and water absorption. Each process deforms and stresses a restored tooth structure in opposite direction. This study evaluated the tooth deformations during these competing processes.
Methods
Large MOD cavities were prepared in 10 extracted molars. Five were restored with a hydrophobic resin composite (Filtek Supreme, 3M ESPE) and the others with a hydrophilic resin-modified glass-ionomer (Ketac Nano, 3M ESPE). The restored molars and two unrestored controls were stored in water for 24 weeks. The molars were digitized with an optical scanner at baseline, after preparation, restoration, and at 1, 2, 4, 8, 16, and 24 weeks water immersion. The digitized buccal, lingual, and restoration surfaces were analyzed to determine their deformation patterns. The results were statistically analyzed using ANOVA followed by Student–Newman–Keuls post hoc tests ( p = 0.05).
Results
The buccal and lingual tooth surfaces moved 13–14 μm inward after restoration. After water immersion, cuspal deformation in the resin composite group gradually decreased, reversing the shrinkage deformation within four weeks. The immersed resin-modified glass ionomer group reversed shrinkage deformation within one week, and continued to expand further to 28 μm after 24 weeks. Cuspal deformations after water immersion were significantly different with the two restoratives. Restoration surfaces also expanded after water immersion, while the control teeth showed no significant deformation.
Significance
Polymerization shrinkage deformation was compensated by hygroscopic expansion within 4 weeks in teeth restored with a hydrophobic resin composite, while a hydrophilic restorative over-compensated polymerization shrinkage within 1 week causing tooth expansion.
1
Introduction
Teeth are structurally designed for a stressful environment, because their function is to apply chewing and biting forces. However, additional non-functional stresses can be introduced during restorative procedures. Since such stresses are in addition to normal function, they can increase the overall stress levels which may lead to premature structural failure of the tooth structure, restoration, or the interface between tooth and restoration. Dimensional changes of restorative materials, which cause internal pressure in a restored tooth structure, are a major source for non-functional stresses. Since dimensional changes are common in most restorative materials during their setting reactions and when they absorb water in the humid oral environment, the study of dimensional changes is highly relevant for the clinical performance of restoratives.
Consequently, contraction of resin-based restorations during polymerization has been extensively studied. Polymerization shrinkage has been associated with various clinical signs and symptoms such as gap formation, microleakage, enamel crack propagation, and post-operative sensitivity . In a humid oral environment, such shrinkage may be alleviated by hygroscopic expansion from water absorption. Whether hygroscopic expansion can compensate polymerization shrinkage has been a topic of interest for several decades . It has been suggested that polymerization shrinkage stresses in bonded resin-based composites is relieved by hygroscopic expansion . More than 97% of the cuspal deflection caused by polymerization shrinkage of a resin composite restoration was reported to have recovered after 6 months immersion in water . Marginal gaps caused by polymerization shrinkage have also been reduced by hygroscopic expansion of composite, compomer, and resin-modified glass ionomer restoratives .
The amount of hygroscopic expansion and thus its clinical impact may vary with material characteristics. Hygroscopic expansion in materials that readily take up water (hydrophilic) can exceed the amount of polymerization shrinkage . Such over-compensation could introduce internal expansion stresses. The outward forces from hygroscopic expansion have raised concerns about the integrity of restored teeth . A giomer material exhibited extensive hygroscopic expansion able to crack enclosing glass cylinders after two weeks immersion in water . Cusp fracture in endodontically-treated teeth has been attributed to hygroscopic expansion of a temporary filling material , while cracks in all-ceramic crowns have been linked to hygroscopic expansion of compomer and resin modified glass ionomer materials used as core build-up and/or luting cements . In addition, a 3-year clinical performance study also suggested hygroscopic expansion as a possible cause for cusp fracture in 13 of 67 teeth restored with an ion-releasing composite .
Dimensional changes, both shrinkage and expansion, of a restorative material can thus have a major impact on the clinical success of a restored tooth. The objective of this study was to evaluate how these competing dimensional changes will affect the stress state in the tooth structure by measuring the deformation of teeth restored with two types of restoratives, where a null-hypothesis presumes no effect on tooth deformation from either the shrinkage/expansion or the materials. The two restorative material types were hydrophobic or hydrophilic, representing different affinity for water absorption.
2
Materials and methods
Two restorative materials were chosen: a hydrophobic resin-based composite Filtek Supreme Plus Universal Restorative (3M ESPE, St Paul, MN), and a hydrophilic nanofilled resin-modified glass-ionomer Ketac Nano Light-Curing Glass Ionomer Restorative (3M ESPE). Details of materials used in this study are listed in Table 1 . These two materials were used to restore teeth in an in vitro study where tooth deformation due to polymerization shrinkage and hygroscopic expansion were recorded over a half year period.
Type | Product a | Description and composition b | Shade | Lot |
---|---|---|---|---|
Resin composite | Filtek Supreme Plus Universal Restorative | Visible-light activated nanocomposite Resin system: BIS-GMA, BIS-EMA, UDMA, TEGDMA Fillers: non-agglomerated/non-aggregated 20 nm nanosilica fillers and loosely bound agglomerates of 5–20 nm zirconia/silica nanoclusters (particle size range 0.6–1.4 μm) Filler loading 78.5% by weight |
A2D | 8BE |
Resin-modified glass ionomer | Ketac Nano Light-Curing Glass Ionomer Restorative | Resin modified light-cure glass ionomer with bonded nanofillers Resin component: methacrylate modified polyalkenoic acid, HEMA, deionized water Fillers: 27% by weight acid reactive fluoroaluminosilicate glass and 42% by weight methacrylate functionalized non-agglomerated/non-aggregated 5–25 nm silica/zirconia nanofillers and loosely bound agglomerates of nano-sized zirconia/silica nanoclusters (particle size range 1.0–1.6 μm) Filler loading 69% by weight |
A1 | AK8AT |
Primer | Ketac Nano Glass Ionomer Primer | One part, visible light-cure primer comprised of methacrylate modified polyalkenoic acid, HEMA, water, and photoinitiators | – | 8AU |
Etchant | Scotchbond Etchant | Etching gel 35% by weight phosphoric acid thickened with fumed silica and a water soluble surfactant |
– | 5EW |
Adhesive | Adper Single Bond Plus Adhesive | Total etch, visible-light activated adhesive BIS-GMA, HEMA, dimethacrylates, ethanol, water, methacrylate functional copolymer of polyacrylic and polyitaconic acids, and photoinitiator system 10% by weight of 5 nm silane-treated spherical silica particles |
– | 6HA |
2.1
Tooth mounting and optical scanning
Twelve extracted human molars were secured in custom-made stainless steel rings ( Fig. 1 (A)) and kept in deionized water throughout the protocol to avoid desiccation. The use of extracted human teeth was approved by the University of Minnesota Institution Review Board (exempt category study number 0707E12482). Each ring contained four embedded spheres surrounding the tooth sample, which functioned as stable reference areas. The spheres were sandblasted and the tooth enamel was etched with 37% phosphoric acid solution to achieve dull surfaces suitable for optical scanning. The tooth samples along with their reference spheres were digitized using an optical scanning system LavaScan ST (3M ESPE), with an estimated lateral resolution of 60 μm and an accuracy of 5 μm ( Fig. 1 (B)). The first scan (baseline) was recorded for all mounted teeth while still intact.
2.2
Tooth preparation, restoration, and water storage
Two mounted teeth were left intact during the study as a control. Large, slot shape mesio-occluso-distal (MOD) cavity preparations were cut into the other ten teeth. The preparations were approximately 4 mm deep and 4 mm wide in buccal-lingual dimension, with 6 mm extensions into buccal and lingual grooves. Each cavity was prepared with a new #245 carbide bur using a high-speed handpiece under copious amounts of water. After preparation, the teeth were digitized.
Five teeth were restored with Filtek Supreme Plus Restorative following manufacturer’s instructions. Scotchbond Etchant ( Table 1 ) was applied to the cavity surface for 15 s and rinsed with water for 10 s. The cavity surface was blotted with Kimwipe. Adper Single Bond Plus Adhesive ( Table 1 ) was applied to the cavity surface for 15 s, lightly air dried for 5 s, and light cured (600 mW/cm 2 ; XL3000, 3M ESPE) for 10 s twice in two locations to cover the entire cavity surface. Filtek Supreme Plus Restorative was filled to a 2 mm thick layer in the cavity, adapted to the cavity walls and margins, and light cured for 40 s twice over the material surface to ensure complete coverage of the restoration. Another 2 mm thick layer of the supreme plus composite was then added, contoured to the anatomical surface and light cured for 40 s twice. The restoration was not polished.
The other five teeth were restored with Ketac Nano Light-Curing Glass Ionomer Restorative following manufacturer’s instructions. Ketac Nano Primer ( Table 1 ) was applied to the cavity surface for 15 s, lightly air dried for 10 s, and light cured for 10 s twice in two locations to cover the entire cavity surface. Ketac Nano Restorative was hand mixed, filled to a 2 mm thick layer in the cavity, adapted to the cavity walls and margins, and light cured for 20 s twice over the material surface to ensure complete coverage of the restoration. Another 2 mm thick layer of the Ketac nano restorative was then added, contoured to the anatomical surface and light cured for 20 s twice. The restoration was not polished.
Immediately after restoration, the teeth were digitized and then individually stored submersed in deionized water at room temperature. The teeth were digitized again after 1, 2, 4, 8, 16, and 24 weeks storage time. The deionized water was periodically changed.
2.3
Data analysis
The digitized surfaces were analyzed with a software program (Cumulus, copyright Regents of the University of Minnesota), using the stainless steel reference spheres to accurately align the digitized samples obtained at different stages. The Cumulus software fit the sphere surfaces in three dimensions by minimizing their root-mean-square difference. Fitting the unchanged surfaces positioned the digital models to show changes in the tooth surfaces during the experiment. These changes were visualized with a linear color scale. The buccal and lingual surfaces above the gingival level of the restoration up to the cusp ridges were selected to calculate the lingual and buccal tooth surface flexure using software custom developed for this project (CuspFlex). The average tooth surface deflection was determined by calculating the volume change divided by the surface area.
2.4
Statistical analysis
The surface deformations of the teeth restored with two different restoratives and the controls at various experimental stages were compared using ANOVA followed by Student–Newman–Keuls post-hoc tests at a significance level of 0.05.
2
Materials and methods
Two restorative materials were chosen: a hydrophobic resin-based composite Filtek Supreme Plus Universal Restorative (3M ESPE, St Paul, MN), and a hydrophilic nanofilled resin-modified glass-ionomer Ketac Nano Light-Curing Glass Ionomer Restorative (3M ESPE). Details of materials used in this study are listed in Table 1 . These two materials were used to restore teeth in an in vitro study where tooth deformation due to polymerization shrinkage and hygroscopic expansion were recorded over a half year period.
Type | Product a | Description and composition b | Shade | Lot |
---|---|---|---|---|
Resin composite | Filtek Supreme Plus Universal Restorative | Visible-light activated nanocomposite Resin system: BIS-GMA, BIS-EMA, UDMA, TEGDMA Fillers: non-agglomerated/non-aggregated 20 nm nanosilica fillers and loosely bound agglomerates of 5–20 nm zirconia/silica nanoclusters (particle size range 0.6–1.4 μm) Filler loading 78.5% by weight |
A2D | 8BE |
Resin-modified glass ionomer | Ketac Nano Light-Curing Glass Ionomer Restorative | Resin modified light-cure glass ionomer with bonded nanofillers Resin component: methacrylate modified polyalkenoic acid, HEMA, deionized water Fillers: 27% by weight acid reactive fluoroaluminosilicate glass and 42% by weight methacrylate functionalized non-agglomerated/non-aggregated 5–25 nm silica/zirconia nanofillers and loosely bound agglomerates of nano-sized zirconia/silica nanoclusters (particle size range 1.0–1.6 μm) Filler loading 69% by weight |
A1 | AK8AT |
Primer | Ketac Nano Glass Ionomer Primer | One part, visible light-cure primer comprised of methacrylate modified polyalkenoic acid, HEMA, water, and photoinitiators | – | 8AU |
Etchant | Scotchbond Etchant | Etching gel 35% by weight phosphoric acid thickened with fumed silica and a water soluble surfactant |
– | 5EW |
Adhesive | Adper Single Bond Plus Adhesive | Total etch, visible-light activated adhesive BIS-GMA, HEMA, dimethacrylates, ethanol, water, methacrylate functional copolymer of polyacrylic and polyitaconic acids, and photoinitiator system 10% by weight of 5 nm silane-treated spherical silica particles |
– | 6HA |
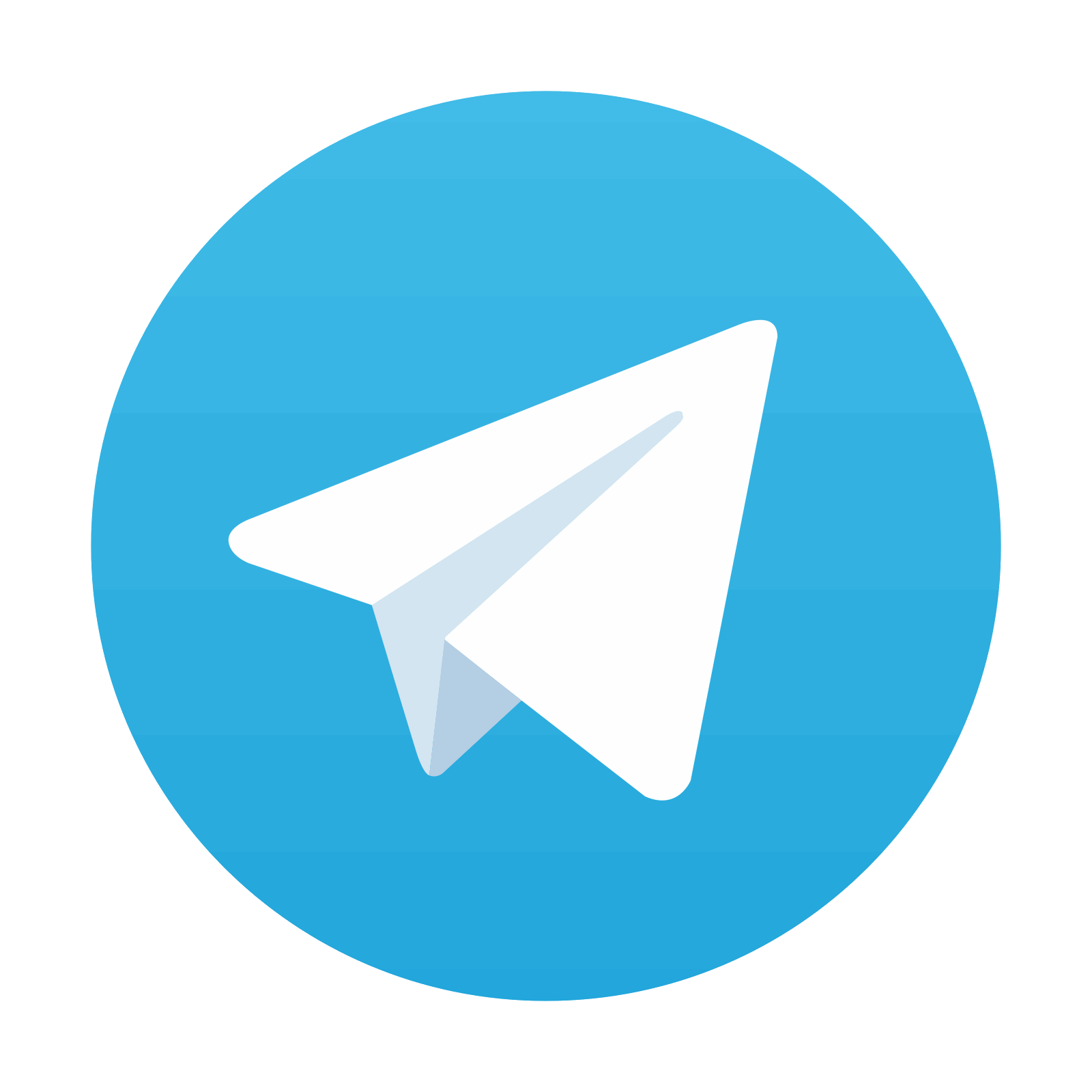
Stay updated, free dental videos. Join our Telegram channel

VIDEdental - Online dental courses
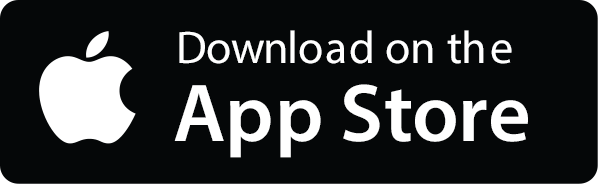
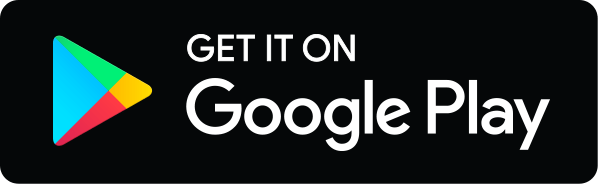
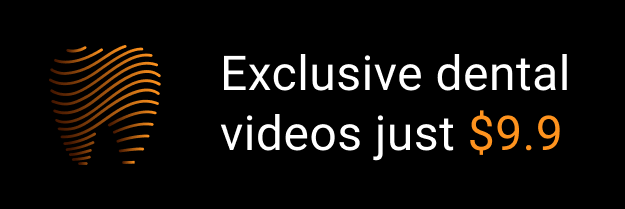