2
Calcium Silicate‐Based Dental Bioceramics: History, Status, and Future
Abubaker Qutieshat, Shahab Javanmardi, and Gurdeep Singh
Adult Restorative Dentistry, Oman Dental College, Muscat, Oman
2.1 Introduction
Dental bioceramics based on calcium silicate are a type of material that belong to the category of regenerative bioactive materials. Conventional type 1 Portland cement served as the foundation for the very first commercially available calcium silicate‐based dental bioceramic. Their superior sealing ability, regenerative capabilities, and antibacterial properties have led to an increase in their application in contemporary endodontic procedures. However, because of factors such as cost, handling properties, extended setting time (during which they run the risk of being washed out of their placement site), concerns over potentially harmful elements contained in the material, and aesthetic considerations, researchers nowadays are developing calcium silicate‐based materials that are more user‐friendly. Enhancing calcium silicate‐based materials has involved the removal of potentially toxic elements, the engineering of the materials’ particle size, the addition of setting accelerants, mixing and flow enhancing polymers, and stabilization mixing gels. All these methods have been effectively utilized in the creation of some newer formulations which are demonstrating a tremendous amount of potential in terms of replacing the conventional types that are currently on the market.
The chemical elements used to make calcium silicate‐based bioceramic materials are only a small subset of the periodic table, consisting of silicon, a metalloid, and calcium, an alkaline earth metal. After a specific need has been identified, the process of developing a new calcium silicate‐based material begins. Following that, an idea for a potential material is developed with the goal of using the new material in dental applications. It is only after selecting the appropriate chemical composition in conjunction with structural and compositional controls that it is determined whether the new biomaterial is suitable or not, and this only after a lengthy cycle of trial and error. A common occurrence in materials science nowadays is that when an improvement in certain chemical and biological properties is achieved from one perspective, it may result in a negative effect on the physical parameters of the material in question from another perspective, which poses a significant challenge. In this chapter, we aim to provide an up‐to‐date view of calcium silicate‐based materials and to provide the reader with the opportunity to acquire a better understanding of the temporal changes that occur in material properties whenever a compositional change is implemented.
The current reliance on the capacity of cells to tolerate cytotoxic substances will be replaced as the new standard of measurement with the capacity of tissues to regenerate themselves. Therefore, the utilization of bioactive materials in dental work will become more prevalent in the future.
2.2 Bioceramics: The What and the Why
Ceramics are produced when compounds, either metallic or nonmetallic, are subjected to high temperatures to facilitate their combination into a single substance [1]. This ultimately results in the formation of a material complex that is made up of the individual compounds being sintered together into a single entity. Bioceramics are any ceramics that are used in the treatment, reconstruction, or repair of diseased or damaged components of the musculoskeletal system. The term “bioceramics” refers to any ceramics that fall under this category. In addition, they can be bioinert (like zirconia), bioresorbable (like tricalcium phosphate), bioactive (like hydroxyapatite), bioconductive (like porous hydroxyapatite coated ceramics), or any combination of these properties [2].
2.3 Endodontic Repair Materials with Ideal Properties
Periodontal tissue is frequently brought into direct and consistent contact with various endodontic materials. The perfect material would be dimensionally stable, nontoxic, and free of carcinogens. It would also prevent the leakage of microorganisms and the associated microbial by‐products; it would be biocompatible with tissue fluid; and it would prevent leakage [3, 4]. Regeneration of tissue complexes, ease of use, and visibility of the material on radiographs are now also included as desirable properties of the material [5].
Throughout the years, numerous materials have been investigated, tried, and evaluated for use as root‐end fillings and for repairing perforations in the root canal system. Studies on the biocompatibility, microleakage, and handling characteristics of a variety of materials that have historically been used in perforation and periradicular surgery have shown that there is not one material that is the master of all. Instead, the studies have shown that some materials are adequate for some tasks, but not for all applications. The fact that the tissues in which materials are implanted are able to tolerate those materials is the best that can be said about those materials. Cell viability assays have been used to determine biocompatibility and bioactivity, as have investigations of genotoxicity [6, 7].
2.4 Calcium Silicate‐based Materials: A Look Back in History
In the April issue of the Deutsche Vierteljahrsschrift für Zahnheilkunde (German Quarterly for Dentistry) in 1878, a dentist named Witte from Hanover, Germany, reported a new method for filling root canals with Portland cement [8]. He praised the method and said it should be used by all dentists. According to the report, Portland cement is finely ground before being stored in a corked bottle to prepare it for dental applications (Figure 2.1). As per Witte’s instructions, and in describing the once novel method back then, a small amount of creosote or carbolic acid and a few drops of water mixed with Portland cement make the cement workable and gradually change its color from ash‐gray to dark brown. The mixture is then placed on a serviette to absorb any excess liquid. After the canal that needs to be filled has dried, the cement is placed inside and covered with gauze for a few minutes to dry. Then, the gauze is removed, the excess cement is removed, and the access cavity is sealed with gold restoration. Witte believed that using this cement as a root canal filling produced better results than using any of the other materials available during his time, such as zinc chloride, which easily corroded, gold, which caused problems because it was a heat conductor, or cotton, which would likely rot.

Figure 2.1 A snippet from Witte’s paper as it appeared in the April issue of the Deutsche Vierteljahrsschrift für Zahnheilkunde (German Quarterly for Dentistry) in 1878 [8].
Interestingly, Witte appears to have reported a case of vital pulp therapy in his paper when attempting to treat a very deep cavity close to the pulp, or what is now known as an irreversibly inflamed tooth. He first tried to treat the patient by filling the deep cavity with amalgam, which caused severe pain, and this is when he decided to use his “novel” method (i.e. by using Portland cement), which resulted in a favorable outcome and the absence of any symptoms for an extended period of time [8].
Schlenker reviewed Witte’s technique two years later and published a paper titled “Filling the root canals with Portland cement according to Dr. Witte” that proposes some modifications to Witte’s original technique [9] (Figure 2.2). It was reported that Witte’s mixture is friable, making its application into the root canal difficult. It was also reported that the crumbly mixture could not completely fill the root canal space. The suggested modification is to pour one or two drops of carbolic acid (not creosote) on a clean glass slab and then pour what runs off back into the bottle. After that, one drop of water is added. Following the mixing of these two liquids, cement is added and mixed to a consistency that does not crumble but is also not too flowy. The mixture is then transferred into the root canal space with a small excavator. As per Schlenker’s instructions, and in describing the modified Witte’s method, the first addition should be pushed deep into the canal with a tweezer‐held small cotton pellet. The cotton pellet can be left inside to absorb excess water before being removed to apply the second and subsequent additions. The excess cement is removed, and the cement filling is capped with zinc phosphate before being thoroughly washed with water to remove any remaining zinc phosphate cement before introducing the gold restoration. Interestingly, Schlenker warned of the risk of tooth discoloration if excess Portland cement is not removed. In this modified technique, zinc phosphate cement is added to provide the required seal that Portland cement would not provide because of its porous nature.

Figure 2.2 A snippet from Schlenker’s paper as it appeared in the January issue of the Deutsche Vierteljahrsschrift für Zahnheilkunde (German Quarterly for Dentistry) in 1880 [9].
Schlenker reported the use of Portland cement on 25 teeth with a one‐year follow‐up and a 100% success rate. It is worth noting that in the study, success was defined as the absence of symptoms and/or infection. The Portland cement material tended to stain anterior teeth, which was the only negative outcome reported among the 25 treated cases (Figure 2.3). As a result, it is safe to assume that this was the first non‐randomized, non‐controlled study ever conducted on a calcium silicate‐based material [9].
It is worth noting, however, that carbolic acid and creosote was commonly used in root canal treatment back in the nineteenth century [10]. However, when the first reports of their toxicity began to emerge, these materials were identified as poisonous and their use began to decline significantly [11]. This could be why any further development on Portland cement was halted, because the materials suggested by Witte to serve as a vehicle were prohibited from being used in dental applications, effectively putting Portland cement development on hold until the first reports of development began to emerge in the 1990s at Loma Linda University [12].
Carbolic acid and creosote solutions in concentrations ranging from 1 to 5% were thought to have stimulating and antiseptic effects when applied to an exposed pulp, facilitating the stoppage of inflammation and preventing necrosis of the remaining vital part of the pulp [13].
2.5 Chemical Properties
Mineral trioxide aggregate (MTA) refers to the first experimental calcium silicate‐based dental bioceramic developed in modern times [14]. Torabinejad at Loma Linda University introduced a modified Portland cement as an endodontic material and patented it as mineral trioxide aggregate (MTA) in the 1990s. In 1998, it was approved by the US Food and Drug Administration and became commercially available as ProRoot MTA (Figure 2.4). When compared to the one used by Witte in 1878, the original material is essentially a refined version of ordinary gray Portland cement, and it contains an additional component known as bismuth oxide (a metal oxide that gives MTA its radiopacity). Portland cement is primarily derived from calcite, a naturally occurring calcium carbonate that accounts for more than three‐quarters of the raw materials mix used in the production of Portland cement clinker. While the primary calcium‐rich raw material may contain significant impurities, a secondary raw material is usually required to provide enough bulk. Typically, this secondary material is clay (argil) or shale [15]. Five years after FDA approval, a study compared Portland cement and MTA and found no significant differences in the elemental constituents of either, with the exception of the addition of bismuth oxide [16, 17].

Figure 2.3 A snippet from Schlenker’s non‐randomized non‐controlled study results conducted in 1880 using Portland cement as a root canal filling material [9].

Figure 2.4 The first commercial form of gray mineral trioxide aggregate (brown pack) and white mineral trioxide aggregate (white pack).
The preferred formula for MTA, according to its US patents, was based on the commercially available “Colton Fast‐Set” brand of cement manufactured by the California Portland Cement Company (Torabinejad and White, 1995).
The presence of calcium phosphate was reported in the first research paper on the constituents of MTA (Loma Linda University) in 1995, authored by the new material’s creators [12]. However, using energy dispersive analysis with X‐ray, Asgary et al. were unable to detect the presence of phosphorus [18]. Camilleri et al. also demonstrated that MTA was devoid of phosphorus [19]. Both of these reports raised concerns among scientists about the accuracy of the 1995 paper. However, subsequent investigations discovered phosphorus levels in MTA products to be very low, near the detection limit of electron probe microanalysis [20, 21], which still did not correlate with the manufacturer’s material safety data sheet [22]. Because it is unlikely that there has been a significant compositional change in MTA materials since the first report, and because Portland cement is primarily composed of silicate and aluminate materials [15, 20, 21], earlier reports [12] of MTA phosphorus content are incorrect [18].
It was later found that the MTA samples reported by Torabinjead in his 1995 paper [12] had been contaminated by prior immersion in phosphate solution [23]. Later, confirming Asgary’s and Camilleri’s research teams’ concerns, it was confirmed that the MTA powder is primarily composed of tricalcium and dicalcium silicates, as well as bismuth oxide. Analysis by X‐ray diffraction revealed that the cement was completely crystalline, with distinct peaks attributable to distinct phases [19].
As was mentioned earlier, Portland cement is primarily derived from limestone (calcite), with clay and shale serving as secondary raw materials. The raw materials are first crushed, ground, and combined in the desired proportions before being heated in a rotary kiln at temperatures ranging from 1400 to 1600 °C. The high levels of heat cause the limestone and clay/shale to break down into the fundamental raw materials, which can then be combined to produce cement clinker. Calcination is the process of transforming raw materials into clinker for use in the cement manufacturing process. The clinker that is produced as a by‐product is allowed to cool, then ground into a powder. After that, gypsum is added to slow down the setting time of the Portland cement that was produced as a result [15]. After calcination, the main constituents of cement clinker are tri‐ and dicalcium silicate (alite and belite), tetra‐calcium aluminoferrite (ferrite), tricalcium aluminate (aluminate or celite), and calcium sulphate dihydrate (gypsum) [24].
MTA in its gray form was responsible for the discoloration of tissues and teeth, which led to the development of the white variant of MTA [25]. As is the case with gray MTA, the elemental components of white MTA do not display any significant differences from those of white Portland cement, with the exception of the presence of bismuth oxide [16]. Despite this, both forms of MTA have been linked to discoloration, which has been attributed to the materials’ characteristic slow hydrating process. This process allows for the absorption and subsequent hemolysis of erythrocytes from the adjacent tissues, which ultimately leads to discoloration of the material and the tooth [25, 26]. The percentage of aluminum oxide, magnesium oxide, and in particular ferrous oxide in gray MTA is significantly higher than it is in the white variant. Both gray variants of ordinary Portland cement and MTA take on a gray hue because of the presence of tricalcium aluminoferrite, and these characteristics sum up the primary distinctions between the two varieties of cement [23].
2.5.1 Heavy Metal Contamination
It was found that gray Portland cement had higher levels of heavy metals than white Portland cement and both variants of MTA, with levels of arsenic and lead being of particular concern [27]. Although favorable constituent analysis and biocompatibility results have been demonstrated using commercial forms of both variants of Portland cement, some authors have even commented that it would be unwise to use commercial Portland cements because of potentially unsafe levels of arsenic [28]. However, in another study, it was found that arsenic levels were comparable between several types of Portland cement and MTA [29]. It was reported that the levels of arsenic in both varieties of MTA were lower than the 2‐ppm safety level required by the ISO standard or dental water‐based cements [30]. Interestingly, Camilleri found that arsenic concentrations were higher than those allowed by the ISO standard, but that effective release in a simulated tissue fluid was found to be negligible [31]. However, it is important to note that none of the formulations that were reported to contain high levels of arsenic were MTA’s precursor, which was the “Colton Fast‐Set” brand of cement that was produced by the California Portland Cement Company.
On a separate but related front, concerns about the toxicity of aluminum and its association with autism and Alzheimer’s disease have led to the production of calcium silicate cements that do not contain the aluminate phase that is present in MTA‐based cements [32]. Biodentine, BioAggregate, EndoSequence, NeoMTA Plus, iRoot BP Plus, and BioRoot RCS are relatively new calcium silicate cements that were manufactured in a laboratory without the presence of an aluminate phase [33]. Biodentine and BioAggregate cements have shown arsenic levels that are comparable to those of Portland cement and MTA [31]. Furthermore, when the acid‐soluble trace element content of each was investigated, Biodentine contained a high concentration of lead, whereas BioAggregate contained a high concentration of chromium [34].
Since the introduction of the sol–gel production method, there has been an increase in the quantity of calcium silicates that are chemically pure. This approach enables the synthesis of cements that have a higher purity at lower processing temperatures, as well as improved control over the structural homogeneity of the final product. For instance, the MTA‐based materials no longer require the utilization of raw materials such as limestone and clay as part of their development. This manufacturing process has the potential to be completely implemented in the near future for the purpose of the production of superior calcium silicate cements [35].
The fineness of Portland cement is measured by surface area and is indicated by the Blaine number (measurement of surface area per gram of material) of the cement. A typical Portland cement has a Blaine number in the 320–550 m2/kg range, with some manufacturers producing finer particle sizes and others producing larger particle sizes [15]. According to the material’s US patents, the preferred Blaine number for MTA is between 450 and 460 m2/kg, indicating that some ordinary Portland cement formulations used in the construction industry have finer particle size than MTA [36]. This also correlates to the fact that the source from which Portland cement‐based material is produced is critical in determining its suitability for use in dental applications.
Particle size comparisons of commercial MTA variants reveal differences between color variants and manufacturers. When it comes to color differences, white MTA has smaller particles and a narrower particle size range than gray MTA. In terms of manufacturer differences, ProRoot MTA has coarser particle size overall, but with more circularity and fewer extra‐large particles than MTA Angelus. ProRoot MTA also has a very similar particle size distribution compared to ordinary Portland cement [37]. The clinical significance of this is that particle size and shape may affect hydration and mineral release from calcium silicate‐based cements.
2.5.2 Setting Reaction
The process of setting calcium silicate‐based cements is a dynamic cascade‐like process. The rate at which the process of hydration occurs varies depending on the ratios of the elementary components. Experimental work in the field of dentistry has focused mainly on analyzing the end products rather than addressing specific dynamics of the setting reaction, therefore, the dental literature does not contain a description that can be taken as definitive of the setting process. Comparisons to the setting of Portland cement have been used to explain the various phases of hydration; however, specific interactions with phosphate‐containing tissue fluids produce specific differences between the setting of Portland cement and calcium silicate‐based dental cements via hydration with water alone, and a biological system in the presence of phosphate‐containing tissue fluids. When calcium silicate cements are wet, a dynamic hydration reaction takes place, and the various material phases hydrate at different rates at the same time. In the initial reaction, calcium oxide hydrates rapidly forming calcium hydroxide. Tricalcium aluminate hydrates shortly after the hydration of calcium oxide [38].
As a result, the setting reaction of Portland cement‐based calcium silicate cements can be simply summed up as the production of a mixed hydrated calcium aluminate, calcium sulphate aluminate, and amorphous hydrated calcium silicate matrix. This matrix is the final product of the setting reaction. The matrix also contains calcium hydroxide in excess, which can be found in crystalline form dispersed throughout the material. The findings of studies using scanning electron microscopy also confirm this complicated mixture of mineral phases. It is possible to observe bismuth oxide randomly dispersed throughout the hydrated matrix when it is utilized as a radioopacifier [39].
The ability of Portland cement‐based materials to form calcium hydroxide as a hydration product and hydroxyapatite in the presence of phosphates makes these materials bioactive [40]. Oral physiological fluids contain a high concentration of phosphate ions, which facilitate the hydration reaction that leads to the formation of carbonate calcium phosphate. The same physicochemical reactions can occur when the materials are immersed in culture medium containing a phosphate source [41]. When Portland cement reacts with phosphate ions, an amorphous calcium phosphate phase forms, which eventually hydrolyzes to form an apatite phase [42]. Amorphous calcium phosphate is a well‐known intermediate compound that precedes the appearance of biological apatites in skeletal calcification [43]. Because the maximum pH in Portland cement can reach greater than 9 when in contact with physiological fluids, this amorphous transformation occurs by the preferential formation of apatite rather than octacalcium phosphate, as is the case in a phosphate‐free medium [42, 44]. Because the hydroxyl ions are incorporated in the hydroxyl sites of the apatites, the continuous pH drop after reaching maximum pH in the Portland cement/physiological fluid reflects this transformation [45]. The specific reaction responsible for this precipitation may be the following, which is a well‐known reaction in any biologic calcification process [34, 46]:

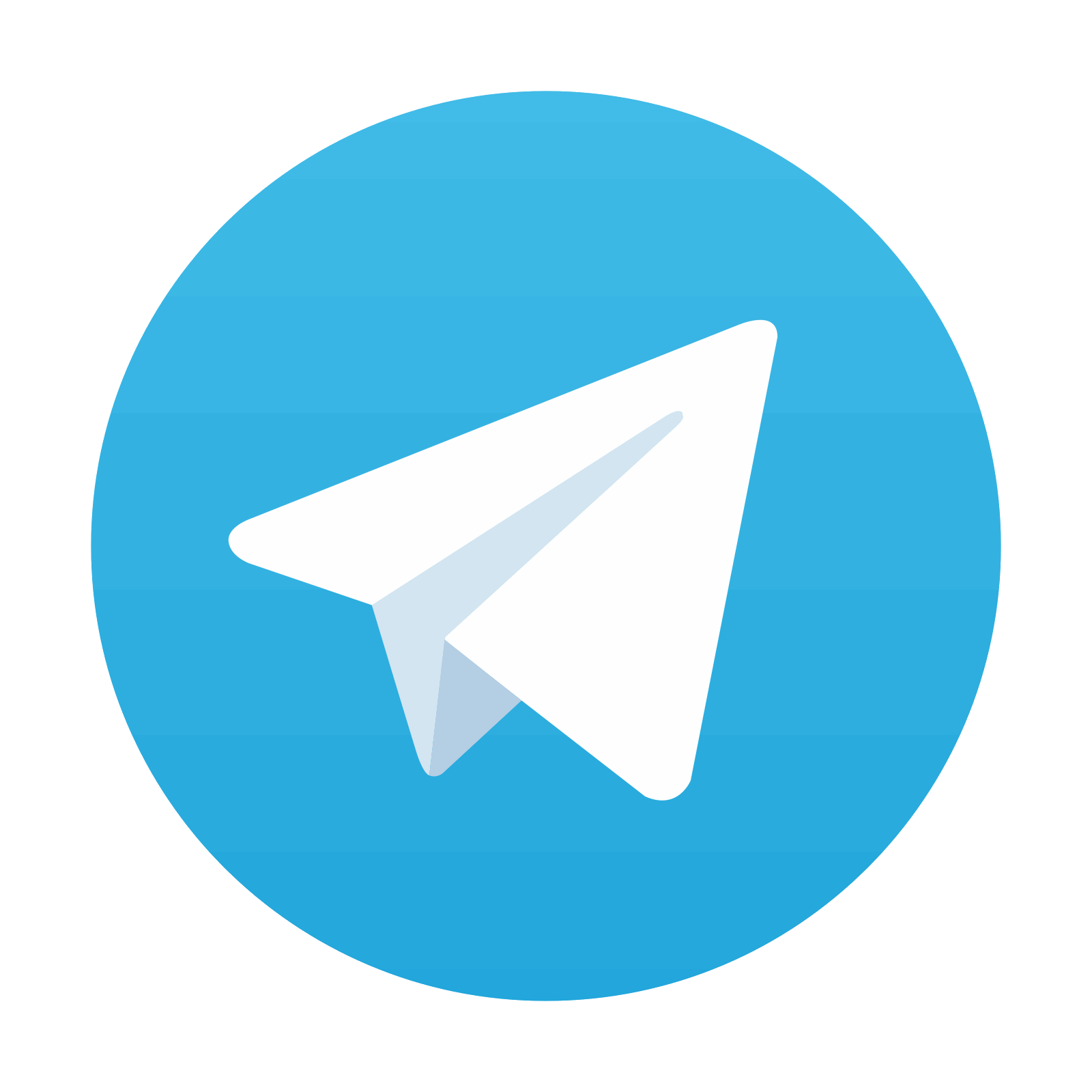
Stay updated, free dental videos. Join our Telegram channel

VIDEdental - Online dental courses
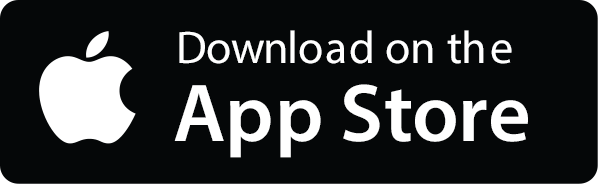
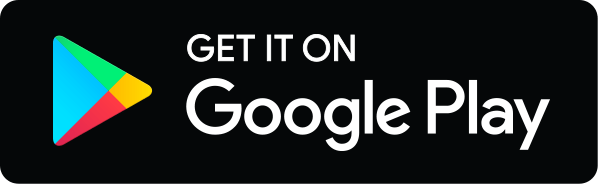