Abstract
Objectives
This study investigated the incorporation of calcium hydroxide (CH) and its effect on pH-neutralization and fundamental properties of model self-adhesive resin cements (SARCs).
Methods
Two-paste SARCs were obtained. Paste A: UDMA/Bis-GMA/TEGDMA/HEMA co-monomer, 25% phosphate monomer (GDMA-P), and 50% glass fillers; Paste B: UDMA/HEMA co-monomer, water, photoinitiators, and 60% glass fillers. CH was added at final concentrations of 0 (control), 0.25%, 0.5%, 1%, 2%, and 4%. Equal volumes of the pastes were mixed before testing. pH-neutralization of the eluate was followed during 24-h storage in distilled water. Other physico-chemical properties were evaluated only for the control and CH concentrations that neutralized the eluate. These evaluations included degree of C C conversion, film thickness, flexural strength/modulus, work-of-fracture, hardness, depth of cure, water sorption/solubility, and dentin bond strength. Statistical comparisons were conducted at a 5% significance level.
Results
All CH concentrations above 0.25% caused pH increase of the eluate at 24 h. The only CH concentrations leading to pH-neutralization were 2% and 4%. Compared with the control cement, the addition of 4% CH decreased the flexural strength, flexural modulus, work-of-fracture, C C conversion, and dentin bond strength. The cement with 2% CH had similar properties to the control cement, except for lower work-of-fracture and lower film thickness. Hardness, depth of cure, and water sorption were not affected by CH. The cement with 4% CH had lower solubility and film thickness than the other materials.
Significance
Incorporation of 2% CH to SARCs may lead to pH-neutralization without dramatically affecting other material properties.
1
Introduction
Self-adhesive resin cements (SARCs) were introduced to simplify the adhesive luting procedures by eliminating the etching, priming and bonding steps. SARCs are gaining increasing popularity because they do not require any pre-treatment of the dental structure, according to the manufacturers’ instructions. After the cement is mixed, its application is carried out with less clinical steps than regular resin-based cements, in a procedure similar to the application of conventional water-based cements. A variety of SARCs is currently available in the dental market.
The adhesive properties of SARCs are attributed to the presence of methacrylate monomers modified by acidic functionalities. These monomers allow demineralization and infiltration of the dental substrate , resulting in micromechanical retention. Because the cement is viscous and therefore its infiltration into the dental tissues is restricted , it has been suggested that the acidic groups could bind with hydroxyapatite, providing additional chemical attachment with the tooth structure .
Compared with regular resin cements, the main differences in the formulation of SARCs are related to the presence of acidic monomers, in addition to the need for water (as a constituent of the cement or available in the bonding substrate) to ionize the acidic monomers to allow their interaction with the dental structures. In some formulations, acid-soluble fluorosilicate glass fillers are also incorporated . Another potential component of SARCs is calcium hydroxide (CH), which is a component of particular value in dentistry in its pure form or as a constituent of a variety of materials. In SARCs, CH might be added with the aim of reacting with the remaining acidic monomers and improve neutralization of the cured cement. It is also hypothesized that the acid–base reactions could be important for the formation of water during the setting reaction; the water is subsequently reused, culminating in transformation to a hydrophobic cement matrix . A recent study has shown that the pH-neutralization potential has a significant impact on the mechanical stability of commercial SARCs .
Despite the possible effects of CH added as a constituent of SARCs, there is no evidence in the literature regarding the impact of this component on the formulation, pH-neutralization and properties of SARCs. Therefore, the aim of this study was to investigate the effect of adding different concentrations of CH on pH-neutralization and fundamental properties of model SARCs. The hypothesis tested was that incorporation of CH would aid in neutralizing the pH of the cured cement without interfering with other material properties.
2
Materials and methods
2.1
Reagents
The (di)methacrylate monomers urethane dimethacrylate (UDMA – Esstech Inc., Essington, PA, USA), 2,2-bis[4-(2-hydroxy-3-methacryloxyprop-1-oxy)phenyl]propane (Bis-GMA – Evonik, Essen, Germany), triethylene glycol dimethacrylate (TEGDMA, Esstech), and 2-hydroxyethyl methacrylate (HEMA – Sigma–Aldrich, St. Louis, MO, USA) were used as received. The phosphate monomer 1,3-glycerol dimethacrylate phosphate (GDMA-P) was synthesized as previously described . The photosensitizer camphorquinone (CQ – Esstech) and the co-initiator ethyl 4-(dimethylamino)benzoate (EDAB – Sigma–Aldrich) were used to render the materials photocurable. Silanated barium borosilicate glass particles (4-μm average size) were used as fillers (Schott, Landshut, Germany).
2.2
Formulation of the model SARCs
The model SARCs were obtained as two paste materials in order to avoid premature acid–base interactions or ionization of the acidic monomers. The pastes had the following constituents (mass fractions):
Paste A: UDMA (10%), Bis-GMA (5%), TEGDMA (5%), HEMA (5%), GDMA-P (25%), and glass fillers (50%);
Paste B: UDMA:HEMA blend at a 3:1 ratio (36%), distilled water (1.6%), CQ (0.8%), EDAB (1.6%), and glass fillers (60%). CH powder was added at a mass concentration of 0, 0.5, 1, 2, 4, or 8% relative to the resin (co-monomer) content of paste B.
For all analysis, equal volume of pastes A and B were mixed for 10 s before testing; thus, the final concentrations of CH tested were 0 (control), 0.25%, 0.5%, 1%, 2%, and 4%. Fourier transform mid-infrared (FTIR) spectra (Prestige-21; Shimadzu, Tokyo, Japan) of mixed materials are shown in Fig. 1 . Photoactivation procedures were carried out using a light-emitting diode unit (Radii; SDI, Bayswater, Victoria, Australia) with 600-mW/cm 2 irradiance.
2.3
pH of the eluate
Photopolymerized discs of each material (diameter 20 mm, thickness 2 mm) were individually immersed in 10 mL of distilled water (pH = 6) immediately after photopolymerization. Baseline pH of the water was measured using a digital pHmeter (An2000; Analion, Ribeirão Preto, SP, Brazil). The discs were immersed in water and pH readings of the eluate were carried out again 1, 5, 15, 30, 60, 120, 240, 360 and 1440 min after immersion ( n = 3). After analyzing the pH data ( Fig. 2 ), the CH concentrations further tested were restricted to 2% and 4% as the other concentrations were unable to neutralize the pH of the eluate.
2.4
Degree of C C conversion
The C C conversion of the resin cements ( n = 5) was evaluated using real-time FTIR spectroscopy with an attenuated total reflectance device (ZnSe crystal). The mixed cement was placed on the ZnSe crystal and covered with a 1-mm thick glass slide to obtain a thin cement film . A preliminary reading for the unpolymerized material (monomer) was taken using 24 scans co-addition and 4-cm −1 resolution. The resin cement was photoactivated for 40 s and another reading was carried out after 5 min (polymer). The C C conversion (%) was calculated as previously described .
2.5
Film thickness
The combined thickness of two optically flat, square glass plates (thickness 5 mm) stacked in contact was measured with a digital caliper accurate to 1 μm. Constant volume of the cements was placed centrally between the plates, and a constant load of 150 N was applied via the top plate for 180 s. The loading system was released and the combined thickness of the two glass plates and cement film was measured. Film thickness (μm) was recorded as the difference between the readings ( n = 5).
2.6
Flexural strength, elastic modulus, and work-of-fracture
Flexural strength tests were performed using bar-shaped specimens ( n = 5) with dimensions of 25 mm × 2 mm × 2 mm photopolymerized between glass slides in a metallic mold by light exposure for 2 min on each side. After 24 h storage in distilled water in the dark at 37 °C, the flexural strength, flexural modulus, and work-of-fracture were obtained in three-point bending on a mechanical testing machine (DL500; EMIC, São José dos Pinhais, PR, Brazil) using a span of 20 mm and a crosshead speed of 0.5 mm/min.
2.7
Knoop hardness
Specimens retrieved from the flexural tests ( n = 5) were embedded in epoxy resin and wet-ground with 600 and 1200-grit SiC abrasive papers. Five hardness readings were performed on each specimen using a microindenter (FM-700, Future-Tech, Kawasaki, Japan), under a load of 50 g and a dwell time of 10 s. The Knoop hardness number (kgf/mm 2 ) for each specimen was recorded as the average of the five indentations.
2.8
Depth of cure
Depth of cure was analyzed by the scraping method. The materials were filled into a cylindrical metallic mold (4 mm diameter, 6 mm height) and irradiated through a polyester strip for 40 s. The cement was extracted from the mold and the uncured material (if any) was removed. The maximum thickness of the cured material was measured with a digital caliper ( n = 3).
2.9
Water sorption and solubility
Cured cylindrical specimens (diameter 15 mm, thickness 1 mm) were dry-stored at 37 °C and repeatedly weighed after 24 h intervals using an analytical digital balance (AUW220D; Shimadzu) accurate to 0.01 mg, until a constant mass was obtained. The specimens were then individually immersed in distilled water and stored at 37 °C. After 7 days, the surface water of the specimens was removed and the mass of each specimen was recorded again. The specimens were dry-stored again at 37 °C and reweighed until a constant mass was obtained. Water sorption and solubility were calculated as the percentage in mass gain or loss during the sorption and desorption cycles.
2.10
Bond strength to dentin
Bovine incisors were embedded in epoxy resin and had their buccal faces wet-ground to expose medium dentin. The dentin surfaces were wet-polished with 600-grit SiC abrasive papers for 60 s. Dentin moisture was controlled with absorbent paper until no surface water was observed. Elastomer molds (thickness 0.5 mm) with a cylindrical orifice (diameter 1.5 mm) were placed onto the dentin surfaces; the orifices were filled with the SARCs and the molds covered with a polyester strip and a glass slide. The specimens were submitted to a 500-g cementation load for 3 min and photoactivated for 60 s. After storage in distilled water at 37 °C, for 24 h, a thin steel wire (diameter 0.2 mm) was looped around the cylinder and aligned with the bonding interface. The shear test was conducted at a crosshead speed of 0.5 mm/min until failure. Bond strength values were calculated in MPa ( n = 12). Modes of failure were classified under a 40× magnification.
2.11
Statistical analysis
For each cement property, including pH-value at 24 h, data were submitted to Analysis of Variance and Student–Newman–Keuls’ test. A 5% significance level was set for all analyses.
2
Materials and methods
2.1
Reagents
The (di)methacrylate monomers urethane dimethacrylate (UDMA – Esstech Inc., Essington, PA, USA), 2,2-bis[4-(2-hydroxy-3-methacryloxyprop-1-oxy)phenyl]propane (Bis-GMA – Evonik, Essen, Germany), triethylene glycol dimethacrylate (TEGDMA, Esstech), and 2-hydroxyethyl methacrylate (HEMA – Sigma–Aldrich, St. Louis, MO, USA) were used as received. The phosphate monomer 1,3-glycerol dimethacrylate phosphate (GDMA-P) was synthesized as previously described . The photosensitizer camphorquinone (CQ – Esstech) and the co-initiator ethyl 4-(dimethylamino)benzoate (EDAB – Sigma–Aldrich) were used to render the materials photocurable. Silanated barium borosilicate glass particles (4-μm average size) were used as fillers (Schott, Landshut, Germany).
2.2
Formulation of the model SARCs
The model SARCs were obtained as two paste materials in order to avoid premature acid–base interactions or ionization of the acidic monomers. The pastes had the following constituents (mass fractions):
Paste A: UDMA (10%), Bis-GMA (5%), TEGDMA (5%), HEMA (5%), GDMA-P (25%), and glass fillers (50%);
Paste B: UDMA:HEMA blend at a 3:1 ratio (36%), distilled water (1.6%), CQ (0.8%), EDAB (1.6%), and glass fillers (60%). CH powder was added at a mass concentration of 0, 0.5, 1, 2, 4, or 8% relative to the resin (co-monomer) content of paste B.
For all analysis, equal volume of pastes A and B were mixed for 10 s before testing; thus, the final concentrations of CH tested were 0 (control), 0.25%, 0.5%, 1%, 2%, and 4%. Fourier transform mid-infrared (FTIR) spectra (Prestige-21; Shimadzu, Tokyo, Japan) of mixed materials are shown in Fig. 1 . Photoactivation procedures were carried out using a light-emitting diode unit (Radii; SDI, Bayswater, Victoria, Australia) with 600-mW/cm 2 irradiance.
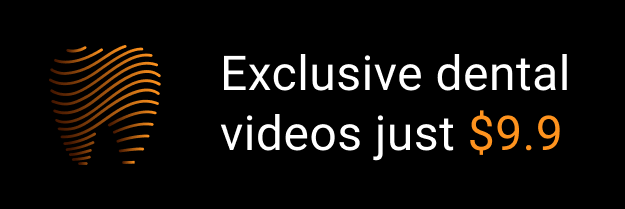