Abstract
Objectives
To evaluate the effect of bulk-filling high C-factor posterior cavities on adhesion to cavity-bottom dentin.
Methods
A universal flowable composite (G-ænial Universal Flo, GC), a bulk-fill flowable base composite (SDR Posterior Bulk Fill Flowable Base, Dentsply) and a conventional paste-like composite (Z100, 3M ESPE) were bonded (G-ænial Bond, GC) into standardized cavities with different cavity configurations (C-factors), namely C = 3.86 (Class-I cavity of 2.5 mm deep, bulk-filled), C = 5.57 (Class-I cavity of 4 mm deep, bulk-filled), C = 1.95 (Class-I cavity of 2.5 mm deep, filled in three equal layers) and C = 0.26 (flat surface). After one-week water storage, the restorations were sectioned in 4 rectangular micro-specimens and subjected to a micro-tensile bond strength (μTBS) test.
Results
Highly significant differences were found between pairs of means of the experimental groups (Kruskal–Wallis, p < 0.0001). Using the bulk-fill flowable base composite SDR (Dentsply), no significant differences in μTBS were measured among all cavity configurations ( p > 0.05). Using the universal flowable composite G-ænial Universal Flo (GC) and the conventional paste-like composite Z100 (3M ESPE), the μTBS to cavity-bottom dentin was not significantly different from that of SDR (Dentsply) when the cavities were layer-filled or the flat surface was build up in layers; it was however significantly lower when the Class-I cavities were filled in bulk, irrespective of cavity depth.
Significance
The filling technique and composite type may have a great impact on the adhesion of the composite, in particular in high C-factor cavities. While the bulk-fill flowable base composite provided satisfactory bond strengths regardless of filling technique and cavity depth, adhesion failed when conventional composites were used in bulk.
1
Introduction
‘Flowables’ are low-viscosity composites obtained from formulations with a filler loading that is 20–25% lower than that of conventional composites . They possess a good wetting ability, which favors their adaptation to the cavity walls, and are therefore expected to decrease the risk for air entrapment and void inclusion . However, the first-generation flowable composites were not suitable for full-depth posterior fillings because of their inferior mechanical properties and increased volumetric shrinkage compared to conventional paste-like composites, primarily due to the lower filler content . Basically, they could only be applied as liner or sealer, or to restore very small cavities . As dental industry constantly searches for materials with improved properties, the latest generations of flowable composites have higher filler content and are claimed to have increased mechanical properties; they thus are now also recommended for larger posterior restorations . To further simplify the filling procedure (and to save precious chair time), the latest trend in composite technology is the development of flowable restorative composites that can be placed in bulk up to 4 mm thickness . Posterior bulk-filling was introduced with the flowable composite ‘SDR Posterior Bulk Fill Flowable Base’ (Dentsply, Konstanz, Germany; in US: SureFil SDR Flow); it still requires a conventional paste-like composite to be placed/cured on top of the 4-mm thick composite base. Due to an enhanced translucency and by incorporating a photoactive group in the methacrylate resin (according to technical information from Dentsply), polymerization kinetics are claimed to be better controlled, enabling the composite base to be injected and cured in bulk up to a depth of 4 mm In the few studies that investigated this flowable composite base, polymerization stress was indeed reported to be considerably lower than that of a conventional flowable composite, being comparable to that of marketed low-shrinking composites , and marginal integrity appeared as good as that obtained with a conventionally layered composite .
Up to now, an incremental layering technique has been the standard to prevent gap formation due to polymerization stress and to achieve an adequate bonding of composite to tooth tissue . In order to test whether or not we can discard the incremental steps and fill the cavity at once, the performance of contemporary flowable composites for posterior restorations needs to be evaluated in deep and narrow cavities. These cavities have a high C-factor , where stress relief due to flow is severely limited and the contraction stress might exceed the bond strength . Moreover, mechanical properties of the composite most close to the interface might be compromised if the curing light loses too much intensity due to attenuation before reaching the bottom of the cavity .
Therefore, the objective of this study was to compare the performance of a universal flowable composite, a bulk-fill flowable base and a conventional paste-like composite in cavities with different C-factors. The null-hypothesis was that the micro-tensile bond strength (μTBS) to dentin does neither depend on the C-factor of the cavity, nor on the used composite.
2
Materials and methods
The study set-up is schematically illustrated in Fig. 1 and the materials that have been used are listed in Table 1 . Ninety-six non-carious human third molars were stored in 0.5% chloramine solution at 4 °C and used within 2 months after extraction. All teeth were mounted in gypsum blocks in order to ease manipulation. All groups were randomly subdivided in four subgroups. In three subgroups (‘Cav’), standard box-type Class-I cavities (3.5 mm × 3.5 mm) were prepared. The depth of these cavities was 2.5 mm in two subgroups (‘Cav2.5’) and 4 mm in one subgroup (‘Cav4’). The latter 4-mm deep cavities were obtained by first building up the molar teeth using the nano-hybrid composite Filtek Supreme XTE (3M ESPE, Seefeld, Germany) in a contrasting shade (A4), after which the cavities were prepared as detailed underneath. In this way, the cavity-bottom dentin in all cavity types (including the flat surface) was mid-coronal, ensuring that effects of regional variability on μTBS were negligible. The C-factor was calculated as the ratio between bonded and unbonded surfaces, as shown in Fig. 1 . The resulting C-factors of the 2.5- and 4-mm cavities were 3.86 and 5.57, respectively. The 2.5-mm depth was chosen because this is the maximum curing depth recommended by the manufacturer of the conventional composite that served as control (Z100, 3M ESPE). Next, the 2.5-mm deep cavities were filled either in bulk (‘Cav2.5-Bulk’) or increments (in three equal horizontal layers ‘Cav2.5-Increment’). Incremental filling decreases the C-factor per layer until approximately 1.95 and the volume is divided by three. The 4-mm deep cavities were filled in bulk (‘Cav4-Bulk’). In the fourth subgroup, flat surfaces were prepared at a depth of 2.5 mm (‘Flat2.5-Bulk’) and a 3.5 mm × 3.5 mm build-up was made in bulk (2.5 mm high) using a silicone mold (C-factor = 0.26, but with the same volume and dimensions as ‘Cav2.5-Bulk’). All preparations were made with a computer-controlled, custom-adapted automatic device (OES Allegra Controller, Trol-Mation Inc., Syracuse, NY, USA), equipped with a cylindrical medium-grit diamond bur (835 314 010, Komet, Lemgo, Germany) mounted in a high-speed air turbine (650, KaVo, Biberach, Germany). A one-step self-etch adhesive was used in all experimental groups (G-ænial Bond, GC, Tokyo, Japan) according to the manufacturer’s instructions. The teeth were randomly divided in three subgroups according to the composite used, resulting in 8 teeth per subgroup. Two flowable composites for posterior filling (‘GUF’, G-ænial Universal Flo, GC; ‘SDR’, SureFil SDR Flow, Dentsply) and a conventional micro-hybrid composite (‘Z100’, Filtek Z100, 3M ESPE) were used (shade A3, except for SDR, which is only available in one shade). The restoration was light-cured with a high-power LED curing device (Bluephase, Ivoclar Vivadent, Schaan, Liechtenstein) with an output of at least 900 mW/cm 2 (40 s in the bulk groups ‘Bulk’; 20 s per layer in the incremental group ‘Increment’). The light intensity was checked before and after each use with the accompanying Bluephase meter (Ivoclar Vivadent).

Material | Composition | Filler load | Maximum layer thickness | Batch no. |
---|---|---|---|---|
Composite | ||||
G-ænial Universal Flo (GC) | Matrix: urethane dimethacrylate, Bis-MEPP, TEG-DMA, pigment; photo-initiator Filler: SiO 2 (16 nm), Sr glass (200 nm) |
69 wt% 50 vol% |
1.5 mm | LOT 1011091 |
SureFil SDR Flow (Dentsply) | Matrix: SDR patented urethane dimethacrylate, dimethacrylate, ethoxylated bisphenol A dimethacrylate, pigment, photoinitiator Filler: barium and strontium alumino-fluoro-silicate glasses |
68 wt% 45 vol% |
4 mm | LOT 1104001464 |
Filtek Z100 (3M ESPE) | Matrix: Bis-GMA, TEG-DMA, pigment, photo-initiator Filler: silica/zirconia (600 nm) |
84.5 wt% 71 vol% |
2.5 mm | LOT 20091001 |
Adhesive | ||||
G-ænial Bond (GC) | Phosphoric ester monomer, 4-MET, hydrophilic methacrylate monomer, water, acetone, photo-initiator, nano-silica | LOT 1012181 |
After one week of water storage at 37 °C, the teeth were sectioned perpendicular to the adhesive-tooth interface using an automated water-cooled diamond saw (Accutom-50, Struers, Ballerup, Denmark) to obtain rectangular 1 mm × 1 mm, non-trimmed micro-specimens for μTBS testing. The specimens were examined light-microscopically (MSA 166305 stereomicroscope, Wild Heerbrugg, Switzerland) at a magnification of 50× to check for the presence of air bubbles or cavity border at the specimen’s interface; these samples were excluded from further testing. The specimens were kept moisturized until tested. They were attached to a notched BIOMAT-jig with cyanoacrylate glue (Model Repair II Blue, Sankin Kogyo, Tochigi, Japan) and stressed at a crosshead speed of 1 mm/min until failure in a LRX testing device (LRX, Lloyd, Hampshire, UK) using a load cell of 100 N. The μTBS was expressed in MPa, as derived from dividing the imposed force (N) at the time of fracture by the bond area (mm 2 ). When specimens failed before actual testing (pre-testing failure: ‘ptf’), they were recorded as zero for further statistical analysis.
The mode of failure was assessed light-microscopically (MSA 166305 stereomicroscope) at a magnification of 50×. Per micro-specimen, occurrence of ‘cohesive failure in dentin’, ‘adhesive failure at the interface’, ‘cohesive failure in composite’ or ‘mixed failure’ was recorded. A failure was classified as ‘mixed’ when a larger region from the surface (>10%) also included cohesive failure in dentin and/or resin. From all groups, some representative fracture surfaces, originating from at least two teeth, were processed for a more in-depth analysis using scanning electron microscopy (JSM-6610LV SEM, JEOL, Tokyo, Japan) after common SEM preparation techniques, including fixation in 2.5% glutaraldehyde in cacodylate buffer solution, dehydration in ascending concentrations of ethanol, chemical drying using hexamethyldisilazane, and gold-sputter coating .
Micro-tensile bond strength data (μTBS per micro-specimen in MPa) were analyzed by Kruskal–Wallis, preceding post hoc multiple comparisons at p < 0.05 (Statistica 10, StatSoft, Tulsa, USA). To estimate bonding performance, the data were also analyzed using Weibull statistics and pivotal confidence bounds were calculated using Monte Carlo simulation . Different groups were compared at the 10% unreliability levels (B10) and at the characteristic strength ( η , scale, also 63.2% unreliability). The slope of the Weibull distribution curve is referred to as β , which is related to reliability. Pre-testing failures were excluded from the Weibull analysis, as Weibull cannot operate with zero values. When fracture occurred ‘cohesively’ in dentin or in composite, yet close to the interface so that it could not be re-glued but not involving the interface, the obtained value was considered as right-censored. All tests were performed at a significance level of α = 0.05 using a software package (R2.12 and Weibull Toolkit 2.1, R Foundation for Statistical Computing, Vienna, Austria and weibulltoolkit 2.1, http://sourceforge.net/projects/weibulltoolkit ).
2
Materials and methods
The study set-up is schematically illustrated in Fig. 1 and the materials that have been used are listed in Table 1 . Ninety-six non-carious human third molars were stored in 0.5% chloramine solution at 4 °C and used within 2 months after extraction. All teeth were mounted in gypsum blocks in order to ease manipulation. All groups were randomly subdivided in four subgroups. In three subgroups (‘Cav’), standard box-type Class-I cavities (3.5 mm × 3.5 mm) were prepared. The depth of these cavities was 2.5 mm in two subgroups (‘Cav2.5’) and 4 mm in one subgroup (‘Cav4’). The latter 4-mm deep cavities were obtained by first building up the molar teeth using the nano-hybrid composite Filtek Supreme XTE (3M ESPE, Seefeld, Germany) in a contrasting shade (A4), after which the cavities were prepared as detailed underneath. In this way, the cavity-bottom dentin in all cavity types (including the flat surface) was mid-coronal, ensuring that effects of regional variability on μTBS were negligible. The C-factor was calculated as the ratio between bonded and unbonded surfaces, as shown in Fig. 1 . The resulting C-factors of the 2.5- and 4-mm cavities were 3.86 and 5.57, respectively. The 2.5-mm depth was chosen because this is the maximum curing depth recommended by the manufacturer of the conventional composite that served as control (Z100, 3M ESPE). Next, the 2.5-mm deep cavities were filled either in bulk (‘Cav2.5-Bulk’) or increments (in three equal horizontal layers ‘Cav2.5-Increment’). Incremental filling decreases the C-factor per layer until approximately 1.95 and the volume is divided by three. The 4-mm deep cavities were filled in bulk (‘Cav4-Bulk’). In the fourth subgroup, flat surfaces were prepared at a depth of 2.5 mm (‘Flat2.5-Bulk’) and a 3.5 mm × 3.5 mm build-up was made in bulk (2.5 mm high) using a silicone mold (C-factor = 0.26, but with the same volume and dimensions as ‘Cav2.5-Bulk’). All preparations were made with a computer-controlled, custom-adapted automatic device (OES Allegra Controller, Trol-Mation Inc., Syracuse, NY, USA), equipped with a cylindrical medium-grit diamond bur (835 314 010, Komet, Lemgo, Germany) mounted in a high-speed air turbine (650, KaVo, Biberach, Germany). A one-step self-etch adhesive was used in all experimental groups (G-ænial Bond, GC, Tokyo, Japan) according to the manufacturer’s instructions. The teeth were randomly divided in three subgroups according to the composite used, resulting in 8 teeth per subgroup. Two flowable composites for posterior filling (‘GUF’, G-ænial Universal Flo, GC; ‘SDR’, SureFil SDR Flow, Dentsply) and a conventional micro-hybrid composite (‘Z100’, Filtek Z100, 3M ESPE) were used (shade A3, except for SDR, which is only available in one shade). The restoration was light-cured with a high-power LED curing device (Bluephase, Ivoclar Vivadent, Schaan, Liechtenstein) with an output of at least 900 mW/cm 2 (40 s in the bulk groups ‘Bulk’; 20 s per layer in the incremental group ‘Increment’). The light intensity was checked before and after each use with the accompanying Bluephase meter (Ivoclar Vivadent).
Material | Composition | Filler load | Maximum layer thickness | Batch no. |
---|---|---|---|---|
Composite | ||||
G-ænial Universal Flo (GC) | Matrix: urethane dimethacrylate, Bis-MEPP, TEG-DMA, pigment; photo-initiator Filler: SiO 2 (16 nm), Sr glass (200 nm) |
69 wt% 50 vol% |
1.5 mm | LOT 1011091 |
SureFil SDR Flow (Dentsply) | Matrix: SDR patented urethane dimethacrylate, dimethacrylate, ethoxylated bisphenol A dimethacrylate, pigment, photoinitiator Filler: barium and strontium alumino-fluoro-silicate glasses |
68 wt% 45 vol% |
4 mm | LOT 1104001464 |
Filtek Z100 (3M ESPE) | Matrix: Bis-GMA, TEG-DMA, pigment, photo-initiator Filler: silica/zirconia (600 nm) |
84.5 wt% 71 vol% |
2.5 mm | LOT 20091001 |
Adhesive | ||||
G-ænial Bond (GC) | Phosphoric ester monomer, 4-MET, hydrophilic methacrylate monomer, water, acetone, photo-initiator, nano-silica | LOT 1012181 |
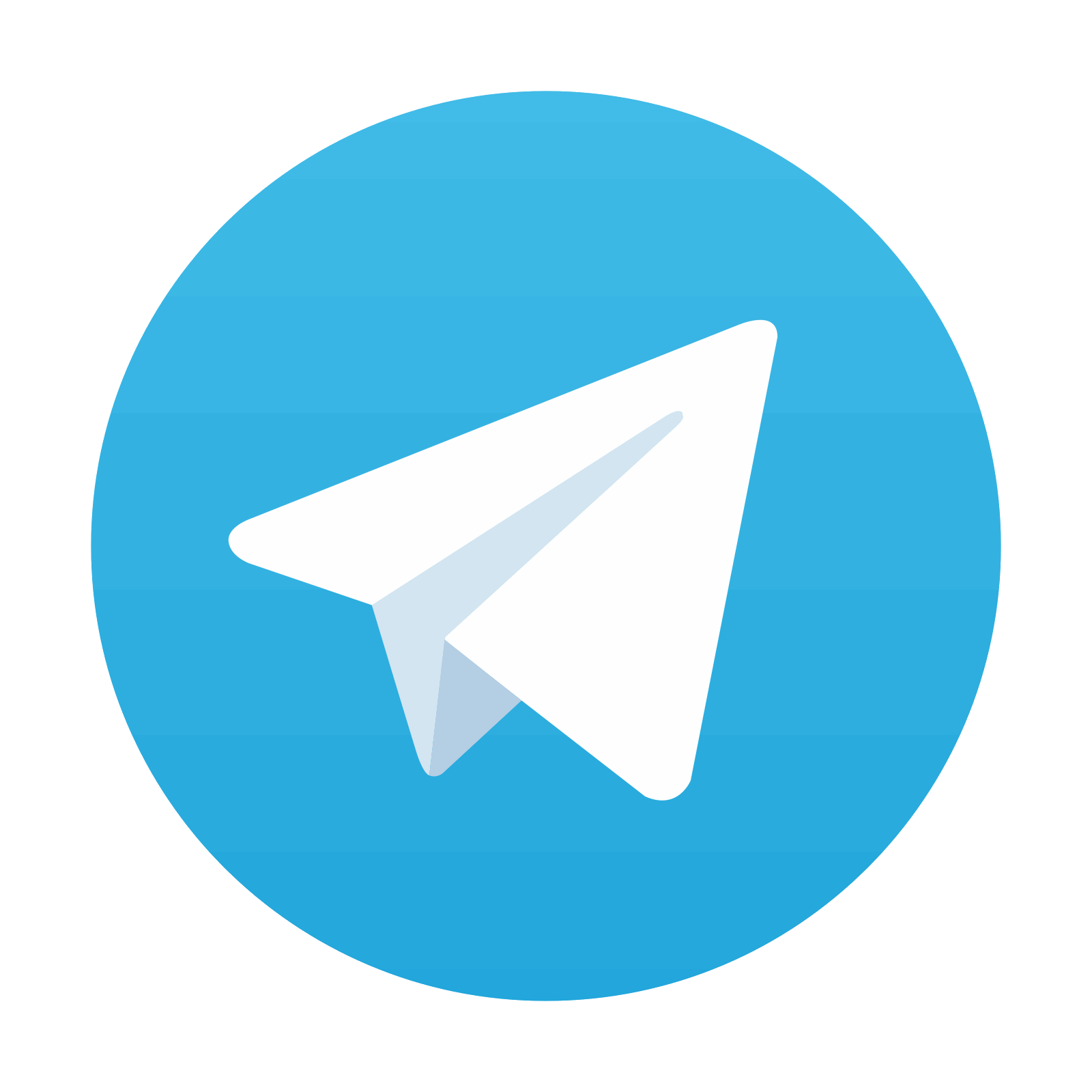
Stay updated, free dental videos. Join our Telegram channel

VIDEdental - Online dental courses
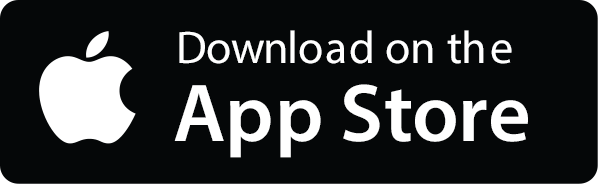
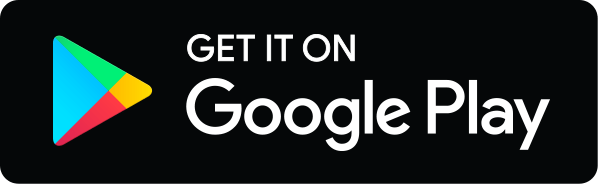
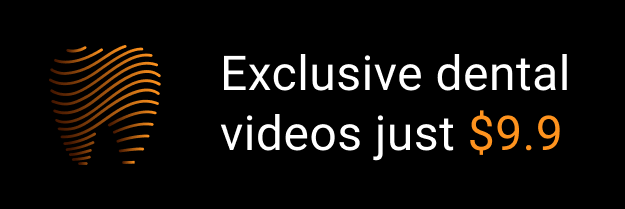