Abstract
Objectives
The aim was to evaluate the polymerization properties of bulk-fill resin composites using two different light-curing protocols, in terms of degree of conversion (%DC), Vickers hardness (HV), polymerization volume shrinkage (PVS) and polymerization shrinkage stress (PSS) and compare them to conventional condensable and flowable resin composites.
Materials and methods
Filtek BulkFill (FBF, 3MESPE, Germany), SDR (Dentsply, Germany), TetricEvoCeram BulkFill (TBF, Ivoclar-Vivadent, Liechtenstein), Venus BulkFill (VBF, Heraeus, Germany), X-traBase (XTB, Voco, Germany), FiltekZ250 (3MESPE) and Filtek Supreme XTE Flowable (FSF, 3MESPE) were investigated. Light-curing was performed for 30 s or according to manufacturers’ instructions (1200 mW/cm 2 , Bluephase20i, Ivoclar-Vivadent). For %DC and HV, discs ( n = 5) of 2 or 4 mm in thickness were prepared and stored for 24 h in distilled water at 37 °C. %DC was determined by FTIR-ATR-spectroscopy. %DC and HV were measured at the top and bottom of the specimens. PVS was measured using Archimedes method ( n = 6). PSS measurements ( n = 10) were carried out using 5 mm diameter PMMA rods as bonding substrates with a specimen height of 1 mm in a universal testing machine. Data were analyzed using one- and two-way ANOVA ( α = 0.05).
Results
Except Z250 in the manufacturers’ light-curing mode, all materials showed no significant inferior %DC at 4 mm thickness. When light cured for 30 s Z250 had no significant differences in %DC at 2 or 4 mm when compared to top. FBF, TBF, FSF and Z250 displayed significant reduced HV at 4 mm in both curing modes. Z250 and TBF showed the lowest PVS and FSF the highest PSS in both curing modes.
Significance
All investigated bulk-fill composites obtained sufficient polymerization properties at 4 mm depth. Enhanced curing time improved the investigated polymerization properties of bulk-fills and Z250.
1
Introduction
One major goal in research and development of resin-based composites (RBCs) is to enhance their clinical longevity and ease of use. Since their introduction in dentistry RBCs have undergone many improvements in filler , matrix and initiator technology . This evolution led to contemporary RBCs showing clinical success rates comparable or higher than those of amalgam . Still one of the main reasons for restoration loss, along with fracture due to mechanical fatigue degradation , is secondary caries .
One of the shortcomings of RBCs is polymerization volume shrinkage due to radical polymerization. The resulting polymerization shrinkage stress is transmitted to the bonding interface and to the remaining dental tissue causing cuspal deflection , enamel cracks, marginal breakdown, gap formation and microleakage . This may ultimately lead to secondary caries and restoration loss. Although clinical evidence of the association between shrinkage stress and in situ failure might be difficult to collect , in vitro results show the need of developing strategies reducing shrinkage stress of RBCs .
Some practical strategies include alternative light curing protocols , the use of flowable cavity liners and incremental filling techniques . As RBCs shrink toward the bonded surfaces during light curing , stress relief can be achieved by flow of material in the pre-gel state from free surfaces into the bulk. The ratio of bonded to free surfaces (c-factor) is minimized by reducing the contact area between resin and cavity walls, as advocated in the incremental filling technique . For the clinical procedure, multiple small increments generally mean increased operational time and are difficult to place in small cavities.
Beside the shortcomings related to polymerization volume shrinkage and stress build up in RBCs, the degree of conversion is another limiting factor when placing restorations. It has been shown that an increment thickness of 2 mm should not be exceeded for RBCs with conventional chemistry . When placing restorations with RBCs in cavities deeper than 2 mm, proper cure may only be assured for incremental build-up techniques . Sufficient curing of RBCs is crucial to achieve appropriate physical and mechanical properties , wear resistance and biocompatibility .
In order to overcome the time consuming incremental cavity filling technique with conventional RBCs, bulk-fill RBCs have been developed. This newly developed kind of RBCs claim to allow the use of material increments up to 4 mm in thickness with low volumetric polymerization shrinkage and resulting low polymerization shrinkage stress . Therefore matrix and initiator chemistry as well as filler technology were optimized. The aims of this study were to evaluate degree of conversion (%DC), Vickers hardness (HV), polymerization volume shrinkage (PVS) and polymerization shrinkage stress (PSS) of bulk-fill RBCs and compare these parameters to those of conventional RBCs.
The null hypotheses were: (i) increment thickness has no significant influence on degree of conversion (%DC) or Vickers hardness (HV) of bulk-fill resin composites and conventional resin composites; (ii) bulk-fill resin composites show no significant lower polymerization shrinkage stress (PSS) and volumetric polymerization shrinkage (PVS) compared to conventional composite resins; and (iii) increased light curing time, compared to manufacturers’ instructions, has no significant influence on the evaluated polymerization parameters.
2
Materials and methods
2.1
Materials used
Five commercially available bulk-fill composites Filtek Bulk Fill Restorative (A2, 3M ESPE, Seefeld, Germany), SDR Surefil (Uni, Dentsply, Konstanz, Germany), Tetric EvoCeram Bulk Fill (IVA, Ivoclar Vivadent, Schaan, Liechtenstein), Venus Bulk Fill (Uni, Heraeus, Hanau, Germny) and X-tra Base (A2, VOCO, Cuxhaven, Germany), a conventional flowable composite Filtek Supreme XTE Flow (A2, 3M ESPE, Seefeld, Germany) and a condensable composite Z250 (A2, 3M ESPE, Seefeld, Germany) were the materials under investigation ( Table 1 ).
Code | Material | Manufacturer batch no. | Shade | Photoinitiators/coinitiators | Monomers | Fillers | Manufacturers’ curing time |
---|---|---|---|---|---|---|---|
FBF | Filtek Bulk Fill Flowable Restorative | 3M ESPE N428456 |
A2 | n.a. | UDMA 10–20 wt% Substituted dimethacrylate 10–20 wt% BisEMA-6 1–10 wt% BisGMA 1–10 wt% TEGDMA <1 wt% |
Silane treated ceramic 50–60 wt% YbF 3 1–10 wt% 42.5 vol% |
20 s |
SDR | SDR Surefil | Dentsply 6251 |
Uni | n.a. | Modified UDMA Dimethacrylate Difunctional diluents |
Barium and strontium alumino-fluoro- silicate glasses 68 wt% 44 vol% |
20 s |
TBF | Tetric Evo Ceram Bulk Fill | Ivoclar Vivadent R72542 |
IVA | Acyl phosphine oxide Camphorquinone Dibenzoyl germanium derivative |
Bis-GMA Bis-EMA UDMA 19.7 wt% organic matrix in total |
Prepolymer fillers 17 wt% Barium glass filler, ytterbium fluoride and spherical mixed oxide fillers 62.5 wt% 60 vol% |
10 s |
VBF | Venus Bulk Fill | Heraeus 10031 |
Uni | n.a. | UDMA EBADMA |
Barium glass filler, ytterbium fluoride and silica 65 wt% 38 vol% |
20 s |
XTB | X-tra Base | Voco 1250061 |
A2 | n.a. | n.a. | Anorganic fillers 75 wt% 58 vol% |
20 s |
FSF | Filtek Supreme XTE Flowable | 3M ESPE N359823 |
A2 | EDMAB <0.5 wt% | Substituted dimethacrylate 15–25 wt% BisGMA 5–10 wt% TEGDMA 5–10 wt% |
Silane treated ceramic 50–60 wt% Silane treated silica 5–10 wt% YbF 3 <5 wt% Functionalized dimethacrylate polymer <5 wt% Titanium dioxide <0.5 wt% 65 wt%/46 vol% |
20 s |
Z250 | Filtek Z250 Universal Restorative | 3M ESPE N217339 |
A2 | n.a. | UDMA 1–10 wt% BisEMA-6 1–10 wt% BisGMA 1–10 wt% TEGDMA <5 wt% |
Silane treated ceramic 75–85 wt% 60 vol% |
20 s |
2.2
Specimen preparation
From each composite, uncured material was inserted into cylindrical stainless steel molds of 8 mm in diameter and 2 mm ( n = 10) or 4 mm ( n = 10) in thickness. To prevent oxygen inhibition, a transparent mylar strip was placed on top and bottom of the mold. Per specimen thickness, half of the specimens ( n = 5) were light-cured from the top as specified by the manufacturer ( Table 1 ), whereas the other half ( n = 5) for an extended light-curing time of 30 s, both with a LED curing unit (1200 mW/cm 2 , Bluephase 20i, Ivoclar Vivadent, Schaan, Liechtenstein). All specimens were polished under profuse water cooling with silicon carbide abrasive paper (4000 Grit) for mirror gloss on top and bottom surfaces. This was performed to create a smooth surface for accurate Fourier transform infrared spectroscopy (FTIR) measurement. All specimens were stored for 24 h in distilled water at 37 °C prior to %DC and HV measurements.
2.3
Degree of conversion (%DC)
For each material FTIR spectroscopy (Nicolet 420 FT-IR Spectrometer, Thermo Fischer, Waltham) with attenuated total reflectance method (ATR, DuraSamplIR II, SensiIR Technologies, Danbury, USA) was performed to assess %DC. Absorption spectra on the top (0 mm) and bottom (2 mm or 4 mm) of each specimen ( n = 5), as well as for the uncured material, were measured with 64 scans at a resolution of 4 cm −1 within a wavelength spectrum of 4000–650 cm −1 . Peak heights at 1637 cm −1 (aliphatic carbon double bonds) and 1608 cm −1 (aromatic carbon double bonds) were measured using the baseline method with the software Origin 8.6 (Origin Lab Corporation, Northampton, USA). The %DC was calculated by the reduction of absorbance at 1637 cm −1 between uncured and cured material using the equation:
% DC = 1 − 1637 cm − 1 1608 cm − 1 Peak height cured 1637 cm − 1 1608 cm − 1 Peak height uncured × 100
The absorption band at 1608 cm −1 served as internal reference for normalization.
2.4
Vickers hardness (HV)
For each material HV was measured on top (0 mm) and bottom (2 mm or 4 mm) of each specimen ( n = 5) using a Vickers impression tester (15 s indentation time, 1 kg load, ZHV 10, Zwick Roell, Ulm, Germany). The length of the diagonals ( d 1 and d 2) left by the indenter was digitally measured under a light microscope (Stemi SV6 with Axiovision LE, Carl Zeiss Microscopy, Jena, Germany). Subsequently HV values, bottom-top-ratios (HV Bottom-Top-Ratio ) and % of maximal hardness (HV Max ) were calculated.
2.5
Volumetric polymerization shrinkage (PVS)
PVS was assessed by Archimedes’ principle of buoyancy using a density determination kit, installed on a high accuracy balance (YDK01 and CP124S, Sartorius, Goettingen, Germany). Densities of the uncured materials ( ρ u , 1 g, n = 6) were determined measuring weight in air and in buoyancy medium (23 °C, 0.1% sodium laurylsulfate aqueous solution). Materials were light cured as indicated by the manufacturer ( Table 1 , ρ c , 0.5 g, n = 12) or for 30 s ( ρ c , 0.5 g, n = 12) with a LED curing unit (1200 mW/cm 2 , Bluephase 20i, Ivoclar Vivadent, Schaan, Liechtenstein). The densities of the light cured materials were weighted after 24 h of storage in distilled water at 37 °C. The volumetric polymerization shrinkage was calculated using the equation:
PS vol . % = ρ c − ρ u ρ c × 100
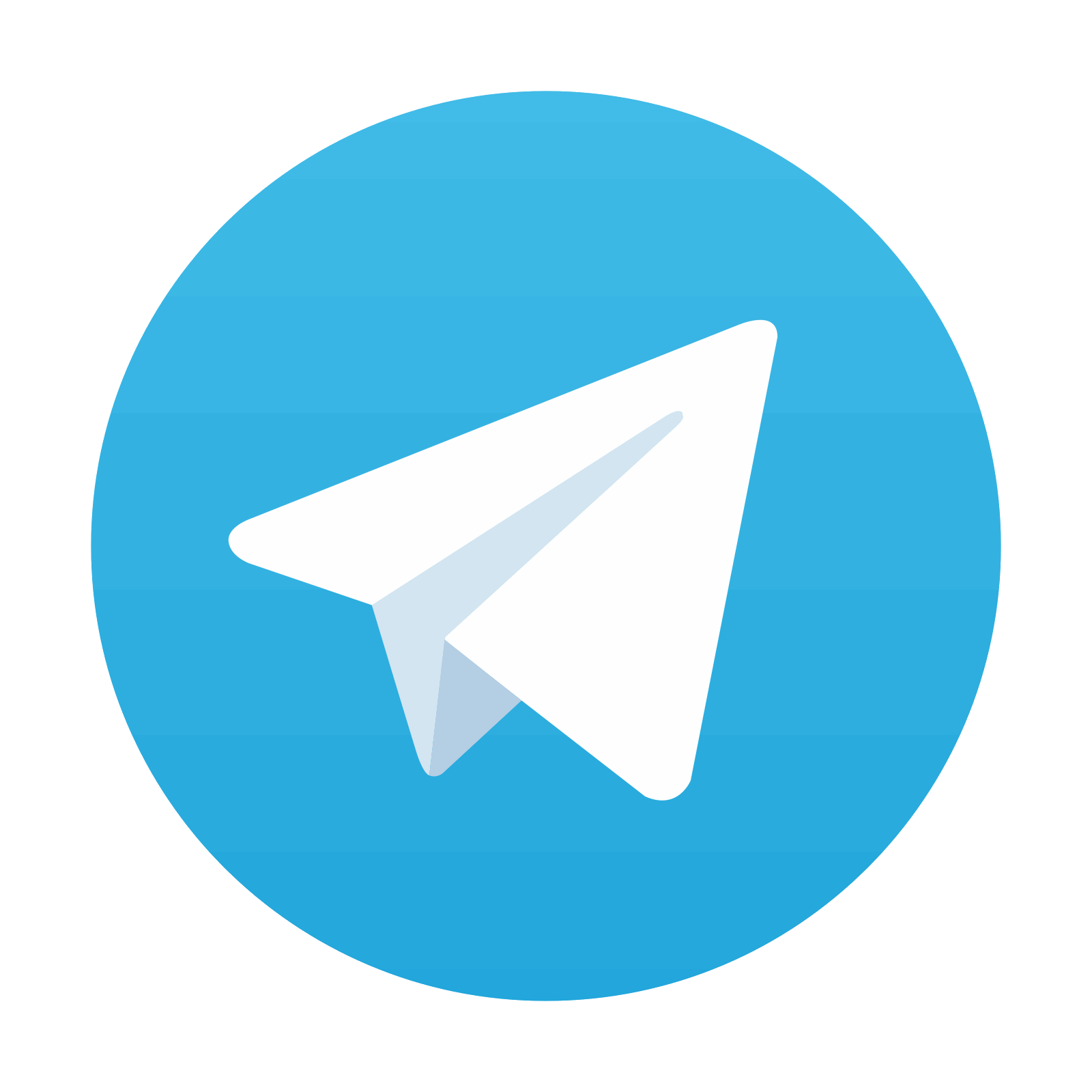
Stay updated, free dental videos. Join our Telegram channel

VIDEdental - Online dental courses
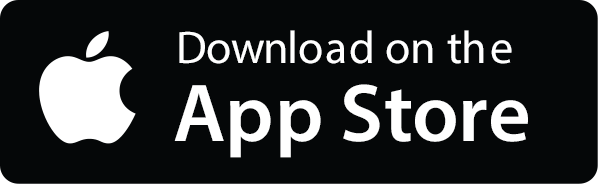
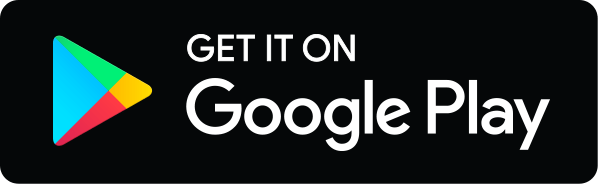