Abstract
The aim of this study was to determine the material composition and cell-mediated remodelling of different calcium phosphate-based bone substitutes. Osteoclasts were cultivated on bone substitutes (Cerabone, Maxresorb, and NanoBone) for up to 5 days. Bafilomycin A1 addition served as the control. To determine cellular activity, the supernatant content of calcium and phosphate was measured by inductively coupled plasma optical emission spectrometry. Cells were visualized on the materials by scanning electron microscopy. Material composition and surface characteristics were assessed by energy-dispersive X-ray spectroscopy. Osteoclast-induced calcium and phosphate release was material-specific. Maxresorb exhibited the highest ion release to the medium ( P = 0.034; calcium 40.25 mg/l day 5, phosphate 102.08 mg/l day 5) and NanoBone the lowest ( P = 0.021; calcium 8.43 mg/l day 5, phosphate 15.15 mg/l day 5); Cerabone was intermediate ( P = 0.034; calcium 16.34 mg/l day 5, phosphate 30.6 mg/l day 5). All investigated materials showed unique resorption behaviours. The presented methodology provides a new perspective on the investigation of bone substitute biodegradation, maintaining the material-specific micro- and macrostructure.
Bone is a dynamic tissue remodelled by osteoblast–osteoclast interactions under the regulation of the RANK/RANKL/OPG system. In bony defect healing, bone grafting materials are the most important acquirement in osseous tissue regeneration. The osteoconductive and osteoinductive features of these materials are controlled by different inherent material characteristics as much as by environmental criteria. The most important requirements for an ideal bone substitute are biocompatibility, osteoinduction, and osteoconduction, and for dental implants, stable long-term osseointegration with the re-establishment of structural tissue to attain a ‘restitutio ad integrum’.
Previous in vitro research has mainly focussed on osteoblast–material interactions, and investigations of bone substitute biodegradation have principally been restricted to animal experiments. Osteoclastic bone resorption occurs following strong attachment of the osteoclast to the hard tissue surface and subsequent generation of an acidic pH of 4.5 within the ‘ruffled border’, a special cellular compartment, to solubilize the alkaline salts of the bone mineral. Prior investigations of bone graft–osteoclast interactions have tended to study the cellular characteristics of bone remodelling processes, but the physico-chemical dissolution behaviour of bone substitutes has yet to be fully determined.
Bone substitute materials include both synthetic and biological substitutes, and are characterized by their chemical composition, crystallinity, and morphology ; these have to meet specific criteria, as defined by their surface structure and configuration, in order to mimic in vivo effects. Calcium (Ca)–phosphate (P) bioceramics of synthetic or natural origin have unique compositions analogous to bone mineral and its specific turnover, which involves, among other processes, physiological Ca and P metabolism. However, the specific mechanisms that underlie these remodelling processes have yet to be fully elucidated.
The aim of this study was to quantify the cell-mediated dissolution behaviour of three different bone substitutes in vitro by osteoclasts, with regard to Ca and P release from the bone substitutes. Furthermore, the cell adhesion qualities and the material composition of the bone substitutes were investigated.
Materials and methods
Bone substitutes
Three different bone substitutes were included in this study, one of bovine origin and two synthetic composite materials: Cerabone ® (Botiss Dental GmbH, Berlin, Germany), a high-temperature sintered material of bovine origin enclosing highly crystalline hydroxyapatite (HA) as the sintered inorganic part of bovine bone, with 50% porosity and particle sizes of up to 1 mm ; Maxresorb ® (Botiss Dental GmbH, Berlin, Germany), a synthetic material composed of 60% HA/40% β-tricalcium phosphate (β-TCP) with macropores of 200–800 μm and micropores of 1–10 μm ; NanoBone ® (Artoss GmbH, Rostock, Germany), a bone substitute based on nanocrystalline HA embedded in a nanostructured silica, which has been described to undergo complete biodegradation by osteoclasts in vivo .
Cell culture
A RAW 264.7 murine monocytic/macrophagic-type cell line (CLS Cell Line Services GmbH, Eppelheim, Germany) was seeded at a density of 3 × 10 7 cells/ml and cultured with 20 ng/ml macrophage colony-stimulating factor (M-CSF; BioCat GmbH, Heidelberg, Germany) and 30 ng/ml RANKL (Axxora GmbH, Lörrach, Germany) in Dulbecco’s modified Eagle’s medium (DMEM; Invitrogen, Carlsbad, CA, USA) supplemented with 10% heat-inactivated foetal calf serum (FCS; Invitrogen) and 1 μg/ml penicillin/streptomycin (Invitrogen) at 37 °C in a humidified 5% CO 2 atmosphere for osteoclast differentiation, as described previously. After 12 days, tartrate-resistant acid phosphatase (TRAP) staining, as well as measurement of osteoclastic marker expression (TRAP, cathepsin K, vitronectin receptor) was performed to determine the osteoclastic phenotype (data not shown). The cells were then passaged into 24-well tissue culture plates (Greiner Bio-One International AG, Kremsmünster, Austria), covered with or without different Ca–P-based bone substitutes in 2 ml medium/well. All materials were obtained directly from the manufacturers in sealed vials and were used without further modification.
Control assays were performed with the addition of 100 nM bafilomycin A1 (Sigma–Aldrich, St. Louis, MO, USA) to wells containing osteoclasts, in order to mediate inhibition of active endocytosis by RAW cells (data not shown). Samples with medium alone served as reference controls.
Sample preparation
After 1 and 5 days, cell culture supernatants were harvested and transferred into glass vials for further treatment. Samples were dried using a red light (150 W) under a laboratory fume hood; 0.2 ml of aqua regia was then added (mixed from 3 parts 65% hydrochloric acid and 1 part nitric acid; Merck KGaA, Darmstadt, Germany) and the samples left for 24 h. This was followed by a final dilution in 3 ml Ampuwa water (Fresenius Kabi Germany GmbH, Bad Homburg, Germany) to filter out any residue with a particle diameter over 1.2 μm remaining after sample drying (FP 30 1.2CA; Schleicher & Schuell BioScience GmbH, Dassel, Germany) in glass containers (bottles with a rolled edge, 3 ml; VWR International GmbH, Darmstadt, Germany).
Inductively coupled plasma optical emission spectrometry analyses
Ca and P ion concentrations in the samples (dilution 1:10) were measured with an inductively coupled plasma optical emission spectrometer (Ametek, Spectro A. I. Inc., Mahwah, NJ, USA) and an SC-4 DXS autosampler (Elemental Scientific, Omaha, NE, USA) at wavelengths of 317.933 nm (Ca) and 177.495 nm (P). The control program of the mass spectrometer performed three consecutive measurements of each sample, delivering the mean and standard deviation of the three results. Ion concentrations were calculated by inductively coupled plasma optical emission spectrometry (ICP-OS), which was calibrated by a standard control dilution series of defined ion concentrations subjected to matrix adjustment, delivering calibration curves as a reference. The detection limit and high resolution were guaranteed by continuous unit calibration before measurement of the first sample and after every 10 samples, and by repeated measurements of random samples as quality control.
Scanning electron microscopy analyses
After removal of the supernatants for ICP-OS analyses, the cells cultivated on bone substitutes for 5 days, as well as the surfaces of the three bone substitutes, were visualized with a scanning electron microscope (SEM, XL30; Philips, Eindhoven, the Netherlands) operating at 10 kV. For this purpose, the cells were fixed with paraformaldehyde (2.5%, pH 7) at 4 °C for 24 h and dehydrated in a graded alcohol series for 1 h each (30%, 50%, 70%, 80%, 90%, and 99.6%). The samples were then immersed in 1,1,1,3,3,3-hexamethyldisilizane (HDMS; Carl Roth GmbH, Karlsruhe, Germany) for 5 min, air dried at room temperature, and mounted on stainless steel stubs with double sticky tabs. The conduction was increased with Leit-C (Conductive Carbon Cement; Plano, Wetzlar, Germany) and sputter coating with platinum (Sputter Coater S150B; Edwards Ltd, Crawley, UK).
Energy-dispersive X-ray spectroscopy analyses
To assess the material composition of the three different bone substitutes, energy-dispersive X-ray spectroscopy (EDX) analyses were performed using a GENESIS 4000 EDX system (EDAX, Mahwah, NJ, USA) attached to the scanning electron microscope described above. For the EDX scans, an acceleration voltage of 20 kV and a magnification of 1000× were used in the SEM. The scan was performed over the whole visible region of the sample, thus determining the average element distribution in the region studied.
Statistical analysis
Statistical tests were performed using GraphPad Prism software version 4 (MacKiev Software). Data are expressed as the mean ± standard deviation. Statistical significance was assessed by Student’s t -test for unpaired observations after verifying normal distribution of the values. P < 0.05 was considered statistically significant.
Results
ICP-OS analyses
The absolute values of the ICP-OS ion concentration measurements are listed in Table 1 . Measurement of Ca and P ion concentrations in samples containing bone substitutes in medium alone showed a slight initial decrease in the basal ion concentrations induced by Cerabone, NanoBone, and Maxresorb after 1 day of incubation. Ca concentrations declined from 27 mg/l as the standard medium control value to 29% thereof for NanoBone, 64% for Maxresorb, and 75% for Cerabone. Similarly, P concentrations diminished from 43 mg/l as the pure medium control value to 23% thereof for NanoBone, 79% for Cerabone, and 88% for Maxresorb. At day 5, these levels remained relatively stable for both Ca and P. Taken together, Maxresorb showed the highest Ca and P medium content, followed by Cerabone and NanoBone. Analyses of the influence of osteoclasts on the dissolution behaviour of bone substitutes showed an initial Ca and P decrease in the medium on day 1, as seen for bone substitutes in medium alone. In combination with Cerabone, the presence of osteoclasts led to lower ion levels compared to the bone substitute alone, whereas incubation of osteoclasts with NanoBone resulted in higher Ca and P levels. For Maxresorb, ion concentrations were lower in the presence of cells on day 1, but had become higher on day 5 compared to the bone substitute incubated alone. In detail, Cerabone and NanoBone exhibited similar concentrations developing for both Ca and P and a subsequent decline to the initial levels on day 5. Maxresorb manifested a different dissolution pattern, as Ca increased to 149% and P to 238% of the medium control value on day 5.
Ion | Condition | Material | Concentration (mg/l) | |
---|---|---|---|---|
Day 1 | Day 5 | |||
Ca | BS | Cerabone | 20.12 | 16.60 |
Maxresorb | 17.28 | 17.03 | ||
NanoBone | 7.71 | 6.46 | ||
BS + OC | Cerabone | 18.13 | 16.34 | |
Maxresorb | 18.90 | 40.25 | ||
NanoBone | 7.81 | 8.43 | ||
BS + OC + bafilomycin | Cerabone | 16.42 | 13.63 | |
Maxresorb | 15.03 | 29.46 | ||
NanoBone | 9.08 | 12.06 | ||
P | BS | Cerabone | 34.11 | 31.10 |
Maxresorb | 37.98 | 55.18 | ||
NanoBone | 9.82 | 12.77 | ||
BS + OC | Cerabone | 29.26 | 30.60 | |
Maxresorb | 39.78 | 102.08 | ||
NanoBone | 11.02 | 15.15 | ||
BS + OC + bafilomycin | Cerabone | 26.74 | 26.09 | |
Maxresorb | 26.10 | 66.42 | ||
NanoBone | 11.12 | 17.21 |
a Absolute values of the ICP-OS ion concentration measurements are presented in mg/l. Data represent the mean values of three independent measurements for each sample.
Statistical analysis revealed significant differences in both Ca and P content in the supernatants between the materials investigated and at both observation time-points, except Cerabone in comparison to Maxresorb for Ca on day 1. Furthermore, Maxresorb showed statistically significant differences in the measured values of both ions when compared between day 1 and day 5. Calculated P -values are listed in Tables 2 and 3 .
Material | Ca | P | |||
---|---|---|---|---|---|
Day 1 | Day 5 | Day 1 | Day 5 | ||
Cerabone | Maxresorb | 0.773 | 0.034 * | 0.021 * | 0.034 * |
Cerabone | NanoBone | 0.021 * | 0.021 * | 0.021 * | 0.021 * |
Maxresorb | NanoBone | 0.021 * | 0.034 * | 0.021 * | 0.034 * |
Material | Ca (day 1–day 5) | P (day 1–day 5) |
---|---|---|
Cerabone | 0.248 | 0.386 |
Maxresorb | 0.034 * | 0.034 * |
NanoBone | 0.773 | 0.149 |
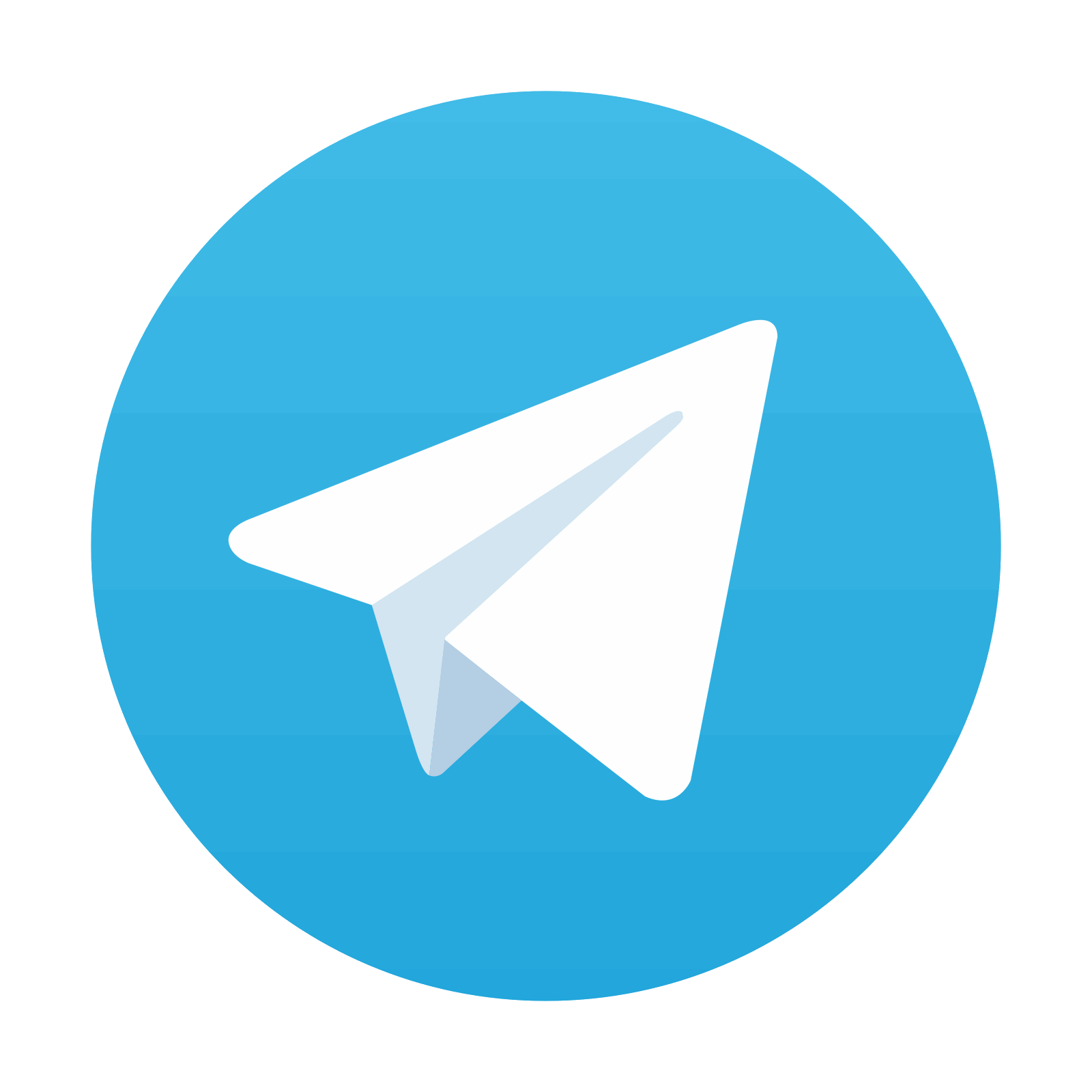
Stay updated, free dental videos. Join our Telegram channel

VIDEdental - Online dental courses
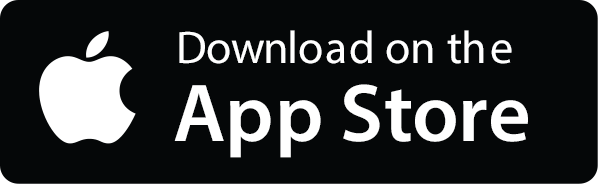
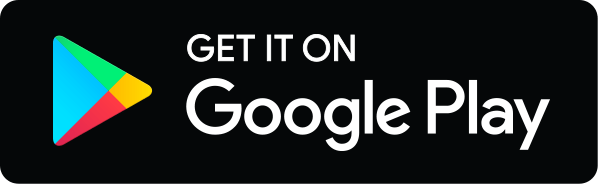
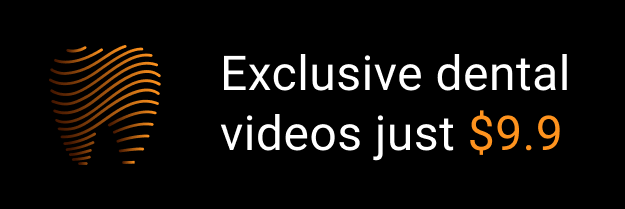