Osseous grafting serves to restore form and function to craniofacial defects. These grafts have been used with the aim of enhancing osteoinductive, osteoconductive, and osteogenic properties to address vertical and horizontal defects so as to render the edentulous ridge more amenable to implant placement. As the biology of bone grafts continues to be unearthed, the use of adjuvants to augment grafts has proved effective. Three-dimensional printing, tissue engineering with the use of stem cells, immunotyping and hormonal therapy all hold promise for the future in the thrust to discover the ideal graft.
Key points
- •
Assessment of the socket and use of graft product along with adjuvants aid in socket preservation with potential for implant placement. No gold standard material has been identified.
- •
Augmentation provides adequate alveolar bone essential for long-term implant survival. Options are autogenous, onlay, guided bone regeneration, ridge splitting procedure, sandwich osteotomy, and alveolar distraction osteogenesis.
- •
Maxillary sinus height is improved with augmentation to allow for placement of implants as a single or staged procedure.
- •
Comprehensive rehabilitation for the edentulous ridge is becoming less invasive. Despite this, interpositional osteotomies, guided bone regeneration, and distraction osteogenesis continue to prove effective.
- •
Adjuvant therapy, 3-dimensional bioplotting, and tissue engineering have been effective in management of osseous defects, and ongoing developments hold promise for the creation of the ideal graft.
Introduction
There have been tremendous advances in public health that have greatly improved the retention rate of dentition. These advances in combination with the explosion of implant dentistry, in particular, implant prosthodontics, has revolutionized the oral rehabilitation of patients afflicted with edentulism. The ”less is more” philosophy for bone rehabilitation of the dental alveolus has seen a distinct rise, resulting in a decrease in extensive complicated reconstruction techniques performed and more novel solutions put forward. Despite this trend, there still is a significant sector of the population that requires enhancement of the alveolar ridge to accommodate implants. This article reviews the current trends in surgical management of the deficient alveolus and looks to future methods and alternatives that show promise of being incorporated in the surgical armamentarium.
History
Grafting of osseous material has been thought to have occurred since antiquity, as detailed in paintings depicting the replacement of a leg afflicted with cancer, by a limb, from a recently deceased Moor. Several artists in the Renaissance age depicted this as “the miracle of the black leg.” The first actually documented account of a bone graft was a xenograft performed by Dr Job van Meekeren in 1663 and published in 1668. In this procedure, a segment of bone from a dog was used to reconstruct a skull defect of a soldier. The first recorded autogenous bone graft was done by Dr Merrem in 1809. Dr William Macewan, a neurosurgeon in Scotland, performed the first allograft in 1878. Professor Vitorrio Putti of Italy helped lay the foundation of the biology of bone grafting, by establishing major principles detailed in an original article written in 1912. Putti’s article outlined basic bone grafting principles that continue to underpin modern-day techniques. In 1965, Dr Marshall Urist, an orthopedic surgeon in California, helped identify and document the importance of bone morphogenetic protein (BMP).
Biology of craniofacial bone grafts
Osseous defects frequently are due to congenital malformation, trauma, loss of dentition, infections, tumor ablation, and osteoradionecrosis. They vary in size and configuration. The aim of bone grafts in the craniofacial region is to restore and preserve form and function. As such, knowledge of the molecular and physiologic processes of grafting is integral in decision making to ensure success.
Four elements are needed for successful bone regeneration: osteoinduction, osteogenesis, intact vascular supply, and osteoconduction. Osteogenesis relies on transplanted osteogenic cells to retain viability and produce osteoid derived from periosteum, endosteum, marrow, and intracortical elements of the graft.
In creeping substitution, bone grafts undergo partial necrosis followed by an inflammatory stage, where the graft is replaced by new bone after vessel invasion. This is referred to as osteoconduction. For osteoinduction, factors are released from the graft and stimulate osteoprogenitor cells to differentiate to osteoblasts. The initial hematoma, inflammatory milieu, and bony remodeling provide the biologic foundation for bone grafting.
Classification of Bone Grafts
Grafts are classified based on their areas of origin and ultrastructure. They are autogenous or nonautogenous, and cortical, cancellous or corticocancellous respectively.
Autogenous is obtained from regional or distal sites. Common intraoral sites are the buttress, torus, symphysis, and the ramus, whereas the most common extraoral sites are the iliac crest, tibia, and the calvarium. Autogenous bone has osteogenic, osteoinductive, and osteoconductive properties, which make it perfect for alveolar augmentation.
Allograft
These grafts are immunogenic due to major histocompatibility complex antigens and carry the risk of viral transmission. Processing, which includes screening and irradiation, reduces infectious transmission to 1 in 1,600,000. Allografts have predominantly osteoconductive properties and the advantage of no patient donor site morbidity ( Fig. 1 ).

Synthetic Bone Graft Substitute
See Fig. 2 .

Adjuvants
Adjuvants are biologics that are used to enhance bone repair. These range from blood components, gene therapy, and recombinant proteins and are classified broadly as cell-based therapy, growth factors, and anabolic therapies.
Biologics provide alternatives with osteoinductive and osteogenic properties that spare the patient additional donor site surgery and foster graft incorporation.
The prototype biologic is BMP. BMPs initially were identified in matrix residue and collagen fibers by Urist in 1968. BMP is a member of the transforming growth factor β (TGF-β) family with more than 20 members identified. They act via serine threonine kinase receptors to up-regulate downstream pathways that affect osteogenic and nonosteogenic activity.
BMP2, BMP4, BMP6, BMP7, and BMP9 have osteogenic potential. BMP2 and BMP7 are the most widely studied subtypes. Ayoub in 2018 revealed dose-dependent BMP2 reconstructed 50% of surgical defects with the main adverse effect being localized swelling. BMP7 10-year follow-up showed similar results as autogenous bone, with good bone regeneration and shorter hospital stay. Like most growth factors, there are issues with short half-lives and rapid clearance.
Vascular endothelial growth factor (VEGF) potentiates angiogenesis. In rodent studies, it increases vascularity and bone quality. Khojasteh and Hossein demonstrated that BMP2 with VEGF had acceptable bone regeneration. Concerns are raised with possible hemangiomas and tumor recurrence with VEGF use.
Platelet-rich plasma (PRP) is an isolate and concentrate of platelets. Platelet concentration can be greater than 2 million cells/μL and confer mitogenic and chemotactic growth factors, such as platelet-derived growth factor, insulinlike growth factor, fibroblast growth factor, TGF-β, and VEGF, leading to much interest in its use. Roffi and colleagues in 2013 discussed PRP use for maxillary sinus augmentation. When used with autologous bone, PRP showed improved handling but demonstrated no significant difference in stability, graft resorption or healing. When used with freeze-dried bone allograft (FDBA) and other carriers, there was increased vital tissue.
PRP served as a predecessor to second-generation platelet concentrate, platelet-rich fibrin (PRF). PRF promotes healing with a better organized fibrin matrix that supports angiogenesis and migration of osteoprogenitor cells. PRF, unlike PRP, lacks laborious processing, requires no anticoagulant and is relatively inexpensive. After venous blood is drawn, centrifugation is done at 3000 revolutions per minute (rpm) for 10 minutes to 12 minutes to produce upper, middle, and lower fractions composed of acellular plasma, fibrin clot and red cells, respectively. Platelets trapped in fibrin in the middle layer (PRF) have been utilized with good effect to enhance bone regeneration during sinus and alveolar ridge augmentation, implant surgery ( Fig. 3 A), postextraction socket grafting, and root coverage. PRF has been used widely as membrane as well as mixed with allograft to form a matrix for grafting ( Fig. 3 B).

In addition to PRF, concentrated growth factors also are isolated from a patient’s venous blood using altered centrifugation with 2400 rpm to 2700 rpm for approximately 12 minutes. It is used to produce a dense fibrin matrix. Both PRF and concentrated growth factors are used as barrier membranes over the growth factor enriched bone graft matrix in the grafting procedure ( Fig. 3 C). Addition of autologous fibrin glue (AFG) to the PRF and particulate graft mixture forms a stable growth factor–enriched bone matrix, known as sticky bone. AFG is obtained through centrifugation of venous blood at 2400 rpm to 2700 rpm for 2 minutes. The top layer obtained would be AFG with red blood cells at the bottom. The AFG can be extracted with a syringe and mixed with the particulate graft and PRF membrane cut into small pieces and allowed to polymerize for 5 minutes to 10 minutes. This results in a stable moldable mass, which prevents movement of the grafted bone and the fibrin network prevents ingrowth of soft tissue into the area.
An injectable form of PRF(i-PRF) has been used to make stable moldable well agglutinated mixture for bone grafting. In this technique, intravenous blood was used with centrifugation for 2 minutes at 3300 rpm. This produced a 2-layered concentrate with an orange-colored fluid at the top consisting of fibrin, platelets, growth factors, and various cell types, including leukocytes and stem cells. Similar to AFG, i-PRF can be extracted with a syringe and mixed with particulate graft to form a moldable stable mass for bone grafting.
The growth factors, alluded to previously, require biomaterial carriers to gain access to the recipient bed. Carriers ideally are highly interconnected porous networks large enough to allow cell migration, fluid exchange, tissue ingrowth, and vascularization. Common carriers include polylactic-co-glycolic acid (PLGA), absorbable collagen sponge (ACS), hydroxyapatite, natural bone matrix (NBM), demineralized bone matrix, β-tricalcium phosphate, and autologous thrombin ( Table 1 ).
Biomaterial Carrier | Preparation Technique | Advantages | Disadvantages |
---|---|---|---|
PLGA | Particulate leaching | Control over porosity, pore sizes and crystalline nature; high porosity | Residual solvents; limited mechanical properties |
ACS | Freeze drying method | Facilitates surgical implantation, retention of growth factor and hemostasis | Low porosity and mechanical strength |
NBM | Production method of cadavers’ bone | High porosity and interconnectivity | Potential host reaction, limited supply, excessive resorption, potential disease transmission |
Postextraction socket grafting
Socket preservation procedures after dental extraction have gained popularity in recent years. Bone quantity, quality, and supporting soft tissue at the time of implant placement determine the longevity of implants. Buccal cortical bone, especially in the anterior and premolar areas, has been shown to exhibit more resorption in comparison with the lingual side. Since the increased awareness of alveolar ridge preservation, multiple studies have been conducted assessing various socket filling techniques and also the use of various biomaterials.
A recently published systematic review concluded that with the current techniques and available biomaterials, there is no gold standard material to preserve extraction sockets and none of the current techniques managed to completely stop alveolar resorption. It was observed, however, that socket preservation decreased vertical and horizontal alveolar resorption and showed better preservation of keratinized tissue. The role of autologous platelet concentrates in accelerating healing and soft tissue epithelialization, while also reducing postoperative pain, also was noted in this review.
Once clinical assessment of the tooth or teeth to be extracted is done, the surrounding bone status is determined by diagnostic imaging, cone beam computed tomography (CBCT) is the most ideal. Atraumatic extraction of the indicated tooth is performed preserving surrounding bone that is available thus ensuring the grafting procedure has an ideal housing for the graft material. The postextraction socket is assessed with regard to whether an immediate implant placement with grafting is possible or socket grafting alone is done. The type of defect then is categorized by the number of surrounding bony walls.
Once the decision to place a graft is made, the extraction socket must be débrided of granulation tissue and irrigated with sterile saline. Presence of purulent discharge in the area could prove to be detrimental to the graft. In such instances, consideration should be given to utilize hydrogen peroxide irrigation followed by copious amounts of a mixture of saline with chlorhexidine 0.12%.
A 5-wall defect with thin walls is treated with the placement of corticocancellous demineralized bone in combination with autogenous blood product (PRF and PRP). The wound is covered with PRF and a collagen plug. These together are held in place with a figure-of-8 suture using resorbable suture material. A healing time of 4 months is adequate for implant placement. Clinical measurements and CBCT scan is performed at the end of the healing period and the available bone height and width is assessed to evaluate the outcome of the grafting procedure ( Fig. 4 ).

Sockets with 3 to 4 wall defects, where loss of bone is seen over the buccal wall and with a lack of bone in the apical area, may require the use of an autogenous block along with particulate graft. The overall goal is to maintain the alveolar bone width and height and limit resorption while supporting the soft tissue structures.
Bone augmentation
Adequate alveolar bone is essential for long-term survival of dental implants. It generally is accepted that there should be 1.5 mm to 2 mm of bone around each implant. Implant positions now are commonly dictated by the restorative positions, so the best methods to augment the deficient alveolar bone should allow for implant placement to match the restorative demands. Augmentation options include autogenous onlay grafting, alloplastic materials, guided bone regeneration (GBR), ridge splitting procedures (RSPs), sandwich osteotomy, and alveolar distraction osteogenesis (DO). These augmentation procedures have different success rates when applied to horizontal versus vertical deficiencies. Autogenous bone continues to be viewed as the gold standard for alveolar augmentation and this has to be taken into account when planning the procedure. The main negative factor of autogenous grafting is donor site morbidity. With the harvesting of bone from intraoral sites, the patient morbidity is decreased compared with extraoral sites (iliac crest, calvarium, tibia, and fibula).
The size and location of the required augmentation dictate the appropriate modality. Alloplastic materials with GBR techniques are useful for small defects, especially in the horizontal plane but usually limited in the vertical plane. Moderate horizontal alveolar defects may be treated with RSPs with or without interpositional grafts, or onlay grafts. In the vertical dimension, onlay grafts often have significant resorption in the vertical dimension, so for moderate defects, a sandwich osteotomy technique is used with interpositional corticocancellous grafts to achieve more predictable height. For large defects in the horizontal direction, onlay grafting usually is the treatment of choice, whereas DO is beneficial for vertical defects.
Horizontal Defects
Horizontal defects are common after tooth loss, especially in the anterior maxilla and posterior mandible. It is important to assess the degree of horizontal bone loss because it dictates the augmentation technique ( Fig. 5 ).

Hourglass Deformity (Buccal Fenestration)
Clinically, there is coronal and apical stability for the implant but with a buccal fenestration. Alloplastic graft material with GBR is sufficient for augmentation ( Fig. 6 ).

Moderate Horizontal Deficiency
If the alveolar ridge width is 3 mm to 5 mm, an RSP should be considered, which provides 1.5 mm to 2 mm of bone on both the buccal and lingual/palatal cortices. The concept of the RSP is that over time, an area of alveolar bone, without a tooth, undergoes atrophy, which occurs more in the cancellous bone than the cortical. The RSP entails placing an osteotomy between the cortical plates in the cancellous bone and slowly expanding the cancellous space to allow for either implant placement or an interpositional graft.
Technique
The incision is placed lingually in order to help with primary closure after expansion. A mucoperiosteal flap is elevated gently, but over the buccal cortex of the expanded segment, the periosteum must be left attached in order to maintain vascularity. The 2-mm twist drill is used to make the initial osteotomy for the implants ( Fig. 7 A). A saw then is used to make the ridge split osteotomy along the alveolar crest, through the previous 2-mm osteotomies, to a depth where the greenstick fracture of the buccal cortex is anticipated to happen. Vertical osteotomies may be used at the anterior or posterior extent of the segment. This osteotomy is taken to the same depth of the ridge split osteotomy and usually is angled at 45°.
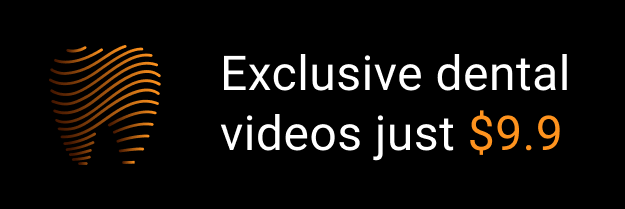