This article examines each class of bone grafting material based on some of the studies in each of the following categories: safety, animal research, periodontal and maxillofacial applications, skeletal grafting, and attempts to qualify the efficacy of each class of material. The article also examines some of the research being done in “tissue engineering” to get a sense of the future of bone grafting.
The replacement of bone is a complex and demanding undertaking. A brief description of bone’s biology and constitutional elements is helpful in understanding the challenges that must be met when its replacement by grafting is the goal. Bone formation occurs when osteoblasts secrete collagen molecules and ground substance. The collagen molecules polymerize to form collagen fibers. Calcium salts precipitate in the ground substance along the collagen fibers to form osteoid. Osteoblasts become trapped in the osteoid and then are called osteocytes.
Mature compact bone is composed of approximately 30% organic matrix and 70% calcium salts. Ninety percent to 95% of the organic matrix is collagen fibers, and the remainder is the gelatinous medium called ground substance, which is composed of chondroitin sulfate and hyaluronic acid. The collagen fibers are oriented along the lines of tensional force. The predominant crystalline salt, composed of calcium and phosphate, is hydroxyapatite—CA10 (P04)6 (OH) 2. Compact bone has hydroxyapatite crystals lying adjacent to and bound to the collagen fibers. The collagen fibers provide tensile strength, and the hydroxyapatite crystals provide compressional strength . Duplication of these constitutional elements comprises some of the grafting materials discussed later in this article.
Bone formation in grafting is characterized by three types of bone growth: osteogenesis, osteoinduction, and osteoconduction. Osteogenesis is the formation of new bone by osteoblasts derived from the graft material itself. Osteoinduction is the ability of a material to induce the formation of osteoblasts from the surrounding tissue at the graft host site, which results in bone growth. Osteoconduction is the ability of a material to support the growth of bone over a surface.
Although not directly responsible for bone formation, an additional characteristic, osteointegration, which is the ability to chemically bind to the surrounding bone, is desirable to aid in the incorporation of the graft at the host site.
Autogenous bone grafts
Autogenous bone grafts, also called autografts, are bone grafts transferred from one site to another site within the same individual. These grafts are the gold standard to which all other grafting materials are compared because they possess all of the previously mentioned characteristics. Because they are from the host itself, there is also an absence of antigenicity.
Autogenous grafts can be cortical or cancellous or a combination of both. Cancellous grafts have the ability to revascularize sooner because of their spongy architecture. This revascularization begins at around the fifth day . Before revascularization, cellular survival in the graft depends on nutrition and elimination of metabolic waste products through plasmatic diffusion. Osteocytes within their lacunae seem to survive if they are within 0.3 mm of a perfusion surface . Cortical grafts require considerable resorption by osteoclastic activity before osteoblastic bone formation. This process is called “creeping substitution” and can produce areas of necrotic bone that persist indefinitely .
As a result of the differing biology of cortical and cancellous bone, the characteristics of a graft composed of each type differ. A cortical graft is strong initially but weakens overtime before regaining strength. There also may be a loss of dimension as a result of a resorption process unless physiologic stress stimulation is producing bone reorganization. Dynamic loading has been shown to be critical for the preservation and increase of bone mass in vivo and, on a cellular level, for modulation of osteoblastic and osteoclastic activity . Cortical grafts have been shown to be 40% to 50% weaker than normal bone from 6 weeks to 6 months after transplantation . Cancellous grafts tend to be weak initially because of their open architecture but continually gain in strength. Physiologic stress stimulation is necessary for continued dimensional and strength stability.
The disadvantages of autogenous grafts are the amount of available graft material and the morbidity associated with their harvest. These disadvantages have led to the development of myriad grafting materials that can be classified into the following categories:
-
Allografts, also called allogenic, homologous, or homografts, are composed of materials taken from another individual of the same species.
-
Xenografts, also known as heterografts or xenogenic grafts, are materials taken from another species.
-
Alloplastic grafts, or synthetic grafts, are artificial or manufactured materials and can be subdivided based on their origin and chemical composition.
There are many and varied combinations of these materials (see list). This article examines each class of material based on some of the studies in each of the following categories: safety, animal research, periodontal and maxillofacial applications, skeletal grafting, and attempt to qualify the efficacy of each class of material. The article also examines some of the research being done in “tissue engineering” to get a sense of the future of bone grafting.
Allografts
Allografts are cadaveric in origin. This type of grafting material is attractive because it closely matches the recipient in constitutional elements and architecture and is theoretically available in unlimited quality. The fundamental problems of this grafting material are antigenicity and the potential for transmission of disease.
Although allografts are treated in various ways, the real and perceived risk of disease transmission still exists. It has been estimated that the risk of HIV transmission is 1 in 1.6 million . There has been one reported case of hepatitis B and three cases of hepatitis C transmission associated with the transplantation of allografts, with the latest case occurring in 1992 . There have been two separate cases of septic arthritis from bone-tendon-bone allografts from a common donor for reconstruction of anterior cruciate ligaments . The US Centers for Disease Control and Prevention conducted an investigation that revealed at least 25 other cases of allograft-related infection or illness .
As recently as March 9, 2006, there was a recall of allograft regenerative products produced by manufacturers, including Tutogen Inc., Regeneration Technologies Inc., Lifecell Corp., Lost Mountain Tissue Bank, and the Blood and Tissue Center of Central Texas. This recall resulted from an investigation of Biomedical Tissue Services, Ltd., a New Jersey company under scrutiny for allegedly procuring tissue from funeral homes without proper documentation . Recalled tissues were tested for HIV, hepatitis B virus, and hepatitis C virus, and as of March 2006, no contaminated allografts were identified . Despite these risks and in recognition of the advantages of bone grafting using allograft material, bone grafting procedures expanded from approximately 10,000 cases in 1985 to more than 1 million in 2004 .
Allografts for maxillofacial and periodontal use generally come as demineralized freeze-dried bone allografts (DFDBA) or mineralized freeze-dried bone allografts (FDBA) and in the form of particles, sheets, blocks, or entire preformed bones. Some researchers propose that removal of the mineral component allows greater expression of osteoinductive proteins ; however, allografts are predominately space-occupying osteoconductive lattices or frameworks. The osteoinductive capability of these products is minimal because of the low concentration of bone growth proteins as a result of the rigorous processes involved in the removal of potential antigenicity and pathogenicity . Piatell and colleagues found that only the DFDBA particles near the host bone were involved in the mineralization process, whereas in FDBA even particles that were farthest from the host bone were lined by osteoblasts actively secreting osteoid matrix and newly formed bone. No osteoinduction was observed with FDBA or DFDBA. There was an increased osteoconductive effect with FDBA.
Noumbissi and colleagues compared mineralized cancellous allograft material to a 1:1 combination of DFDBA and deproteinized mineralized bovine bone in bilateral sinus grafts and concluded that resorption and replacement by new bone occurred more rapidly in the mineralized cancellous allograft material but that both groups resulted in successful new bone formation. Two years after completion of the study there were no differences in osteointegration or stability of implants placed in either material. Schwartz and colleagues demonstrated that different bone bank preparation of DFDBA, even from the same bank, varied considerably in their ability to induce new bone formation and further concluded that the ability to induce bone formation seems to depend on the donor age. Fucini and colleagues studied allograft particle size and found no statistically significant difference in bone fill in periodontal osseous defects between different particle sizes of DFBA in humans. Glowacki stated that we cannot conclude what the performance of different lots of demineralized bone allografts will be in vivo or in vitro and that test systems should be used as a measure of clinical performance. The author also called for an osteoconductivity standard for products that are to be released to market followed by clinical monitoring.
Animal studies have demonstrated (1) improved skeletal healing in mice with the use of demineralized bone matrix + hyaluronan , (2) better bone fill in critical-sized defects in baboons using DFDBA combined with tendonous collagen , (3) stable augmentation of the sinus floor with the use of deproteinized bone particles in rabbits , (4) comparable mechanical loading of implants with the use of homogeneous demineralized freeze-dried bone in one-stage sinus lift procedures in sheep when compared with autogenous cancellous bone from the iliac crest , and (5) new bone formation induced by active DFDBA and a dose-dependent increase in new bone area that exceeded that induced by active DFDBA caused by the addition of rhBMP-2 to inactive DFDBA .
Human studies and case reports of the use of these materials in the maxillofacial region have yielded the following information:
-
Ridge augmentation and sinus grafting with freeze-dried bone allograft in combination with platelet-rich plasma provides a therapeutic alternative for implant placement .
-
Mineralized, solvent dehydrated cancellous bone allografts were replaced by newly formed bone significantly faster and in greater quantities in the maxillary sinus when compared with a composite of DFDBA plus deproteinized bovine bone xenografts .
-
Allogenic bone block material is an effective alternative to autogenous bone for implant site development .
-
Van Den Bergh and colleagues reported the placement of 69 implants in 30 sinuses grafted with DFDB without the loss of a single implant.
-
Minichetti and colleagues studied the grafting of extraction sockets with particulate mineralized bone allograft and concluded that it demonstrated the formation or remodeling of bone and was clinically useful in maintaining bone volume for implant placement after extraction.
-
Grogan and colleagues reported that “allograft bone produced reliable results with a satisfactory outcome” in posterior spinal fusion for correction of idiopathic scoliosis.
-
Cammisa and colleagues compared a demineralized bone gel to iliac crest autogenous grafts, with each patient acting as his or her own control. They found bone fusion in 52% of the allograft side and 54% of the autogenous side.
Summary
Although the results of these studies do not yield consistent results, they demonstrate that allografts are osteoconductive and some are possibly osteoinductive. Under the right circumstances and with proper patient and site selection, they provide an acceptable material for grafting.
Xenografts
The disadvantages of allografts, including disease transmission, antigenicity, supply, and psychological aversion, have led to the exploration of xenografts as an alternative grafting material. Xenografts are bovine in origin and carry the theoretical risk of transmission of bovine spongiform encephalopathy. Theoretical and experimental data, however, indicate that the use of these materials does not carry a risk for transmitting bovine spongiform encephalopathy to humans . Sogal and Tofe applied the risk assessment models of the German Federal Ministry of Health and the Pharmaceutical Research and Manufacturers Association of America to a bovine bone graft substitute and concluded that the risk of bovine spongiform encephalopathy transmission was negligible. This was attributed to the stringent protocols followed in sourcing and processing.
Animal studies have revealed the following information:
-
Bovine bone granules possess better osteoconductive potential than bioglass crystals and hydroxyapatite when tested in New Zealand rabbits .
-
Xenogenic demineralized bone matrix was osteoconductive when implanted in rats .
-
Bovine bone xenograft was to be more effective than particulate dentin combined with plaster of Paris in forming new bone in calvarial bone defects in rats .
-
Xenographic grafts undergo slower resorption than autogenous grafts when placed in mandibular lateral surface defects in dogs .
-
Xenografts were essentially osteoconductive when examined in monkeys .
Human use of xenografts has demonstrated the following findings:
-
Bovine bone mineral grafts, when used with barrier membranes, improved clinical and radiographic parameters of deep intrabony pockets .
-
Excellent integration of inorganic bovine material with newly formed bone suggests that the material can be used for onlay grafting procedures .
-
Biocompatibility and successful use occur in rebuilding atrophic alveolar ridges when supported by a configured titanium mesh .
-
Success in sinus elevation procedures with or without implant placement occurs when used alone or in combination with venous blood, platelet rich plasma, and autogenous bone .
A unique regenerative product combines an anorganic bovine bone matrix with Pepgen-15 (P-15), a synthetic peptide that mimics the cell-binding domain of type 1 collagen . Because collagen forms the scaffold for cell attachment—migration—and modulates cell differentiation and morphogenesis by mediating the flux of chemical and mechanical stimuli and because the P-15 peptide represents the cell-binding site of collagen, it was hypothesized that materials coated with P-15 should act as an effective substitute for autogenous bone grafts . Bhatnagar and colleagues have demonstrated that this material produced enhanced bone formation within a shorter time interval compared with a composite graft material composed of anorganic bovine bone and DFDBA. Thompson and colleagues compared a P-15 product to mineralized FDBA and coralline hydroxyapatite in 13 maxillary extraction sockets and found that the P-15–containing grafts produced the highest amount of vital bone. Human osteoblasts have been shown to demonstrate the greatest proliferation and differentiation in vitro when applied to a P-15–containing graft material as compared with coralline hydroxyapatite, low temperature bovine hydroxyapatite, alpha tricalcium phosphate, and high-temperature bovine hydroxyapatite . Similar results were demonstrated when P-15 was combined with hydroxyapatite calcified from red algae .
Alloplasts
Alloplastic materials that have been investigated and manufactured include hydroxyapatite, coral- and algae-derived hydroxyapatite, the calcium phosphates, calcium sulfate, collagen, and polymers. These synthetic materials are inert with no or little osteoinductive activity, with the exception of P-15, which is claimed to stimulate the differentiation of mesenchymal cells into osteoblasts .
The advantages of alloplastic grafts include an absence of antigenicity, no potential for disease transmission, and unlimited supply. These materials can be treated to be resorbable or nonresorbable, are provided in various particle or pore sizes, are combined with various carriers to improve handling characteristics, or are combined with bioactive proteins to provide osteoinduction. Animal studies of these materials have demonstrated the following findings:
-
Bone formation in monkey extraction sites and dog infrabony periodontal defects with a hydroxyapatite/agarose gel .
-
Bone fill in rat calvarial defects, when hydroxyapatite was combined with chitosan glutamate , cultured bone marrow osteoblasts , and reconstituted collagen microspheres .
-
Mineralization rates for nanoparticle hydroxyapatite that were comparable to autogenous bone in pig osseous defects .
-
Bone formation with porous hydroxyapatite in posterolateral lumbar fusion in sheep .
Human studies revealed these findings:
-
Hydroxyapatite bone cement seems to hold great promise as a grafting alloplastic material for sinus floor augmentations .
-
Hydroxyapatite can be used as a porous ceramic or as a paste/cement bone graft material in humans in the hand , cranium , and tibia .
Coral- and algae-derived hydroxyapatite
Because coral- and algae-derived hydroxyapatite has similar architecture and similar mechanical properties to cancellous bone, much research has gone into its use as a substitute graft material on its own or combined with other substances . Unlike bone, coral’s inorganic component is calcium carbonate, which can be exchanged for phosphate to produce coralline hydroxyapatite . Like other synthetic materials, coral- and algae-derived hydroxyapatite is not osteoinductive or osteogenic . Its structure and composition mimic natural bone, however . Pore size and interconnectivity and particle size have been shown to influence bone regeneration and growth . A minimum pore size of 100 nm is required for ingrowth of connective tissue or osteoid, with an ideal pore size of approximately 100 to 135 nm .
The rate of a material’s resorption is a critical element in a graft’s success as the material maintains a desired volume that should be replaced with bone. Premature resorption of graft material may result in inadequate volume of the replacement . This rate of resorption is influenced by porosity and the composition of the graft material .
Simunek and colleagues demonstrated that a material derived from sea algae was gradually resorbed and replaced by newly formed bone. Ewers and colleagues showed that marine-derived hydroxyapatite material combined with 10% autogenous bone and plasma-rich protein produced comparable—and in some cases better—results than autogenous grafts in sinus augmentation procedures . Similar results were obtained with the use of this material in the foot and ankle and iliac crest . Less promising results were produced when it was used for spinal fusion in rabbits and humans .
Bioactive glass
Bioactive glasses were introduced more than 30 years ago as bone substitutes. The designation “bioactive” relates to their ability to bond to bone and enhance bone-tissue formation. This is thought to be a result of the similarity of surface composition and structure of the bioactive materials to the mineral component of bone. This bioactivity depends on an intimate contact with bone and is limited in nature . Because of these characteristics, studies have used this material as stand-alone bone grafting materials and scaffolds for osteoinductive proteins and osteogenic cells. The rate and degree of resorption are a function of architecture, particle size, and manufacturing methods .
Research in animals has yielded conflicting results.
-
Moreira-Gonzalez and colleagues concluded that “the use of bioglass granules to repair large craniofacial defects cannot be advised.” This statement was based the study of the repair of critical sized calvarial defects in rabbits.
-
Griffin and colleagues looked at metaphyseal defects in sheep and found that defects filled with mixtures that contained 50% to 100% bioactive glass contained less bone and more fibrous tissue than defects filled with allograft, autograft, or allograft combined (<50%) with bioglass.
-
Hall and colleagues found no statistically significant difference between bioactive glass and no material in the repair of intrabony defects around implants in the canine mandible and found that DFDBA produced better bone to implant contact and better bone height fill than bioactive glass material.
Other studies have come to different conclusions:
-
Wheeler and colleagues studied critical sized distal femoral cancellous bone defects treated with bioactive glasses and found that all grafted defects had more bone than unfilled controls.
-
Cancian and colleagues found total repair of surgically created defects in monkey mandibles with intimate contact of the remaining particles of bioactive glass and newly formed bone at 180 days.
-
Research on the use of bioactive glass in spinal fusion in rabbits has led others to conclude that it may have potential as a bone graft material .
-
When this material was looked at for improved healing in extraction sockets or sinus floor augmentation, either alone or in combination with other grafting materials (DFDBA, autogenous bone), it was found to be effective for bone regeneration .
Calcium phosphates and calcium sulfate
Calcium phosphate is the name given to a group of minerals that contain calcium ions (Ca 2+) combined with orthophosphates (PO4 3−). Tricalcium phosphate Ca3 (PO4)2, which is also known as Whitlockite, occurs in alpha and beta phases . Hydroxyapatite, Ca10 (PO4) , (OH)2 is the principle mineral component of bone . Calcium sulfate (CaS04) is better known as plaster of Paris or gypsum and has been used as synthetic bone graft material for more than 100 years .
Calcium sulfate
Human studies most recently have concentrated on the use of this material in combination with other graft materials. Maragos and colleagues looked at its use combined with doxycycline and DFDBA in the treatment of class II mandibular furcation defects in humans and found that either of these additions significantly enhanced the clinical outcome than did calcium sulfate alone. Borrelli and colleagues concluded that medical grade calcium sulfate increases the volume of graft material, facilitates bone formation, and is safe in the treatment of nonunions and fractures with osseous defects. Other researchers also have demonstrated this material’s biocompatibility and osteoconduction . Herron and colleagues demonstrated resorption of calcium phosphate and its replacement with bone in rabbits.
Calcium phosphate
Blokhuis and colleagues compared calcium phosphate with autogenous bone grafts in 3-cm tibial segmental defects in sheep and concluded that calcium phosphate does not provide an alternative to autogenous grafts for this use. Linhart and colleagues concluded that calcium phosphate cements represent a good alternative to autogenous bone transplantation, especially in elderly patients.
Tricalcium phosphate has been shown to have no adverse effect on cell count, viability, and morphology and can provide a matrix that favors limited cell proliferation in vitro . Rabbit 1-cm diaphyseal segmental defects treated with calcium sulfate combined with mesenchymal stem cells gave evidence of the use of this material as an alternative to autografts . When tricalcium phosphate was compared with inorganic bovine bone in dog mandibular defects, tricalcium phosphate showed significantly greater bone formation at 12 and 24 months and better resorption than inorganic bovine bone . Several studies in human sinus augmentation, either alone or in combination with other substances, have demonstrated its use as an effective bone grafting material . Ultraporus beta tricalcium phosphate used in 24 patients with orthopedic bone cavity defects exhibited steady resorption and trabeculation with time, but incorporation was not complete at 1 year in large defects .
The future
Current avenues of research in molecular biology, progenitor cell use, and biomimetic scaffolds hold promise for the future of bone replacements by defining and employing the complex of stimuli and processes that can result in bone formation. Postnatal progenitor cells have demonstrated the capacity to differentiate into a multitude of cell types . Mesenchymal stem cells can be harvested from bone marrow and demonstrate extensive proliferative ability and the capacity to be guided into bone-forming cell types . Their availability is a limiting factor because their fraction in marrow has been estimated to be as low as 1 in 27,000 cells . Adipose tissue–derived progenitor cells also have been investigated . They possess the advantages of availability and accessibility and have demonstrated capabilities similar to bone marrow–derived cells. In vitro and in vivo studies have demonstrated their ability to form bone .
The molecular processes of the multitude of factors in platelet rich plasma, pro-osteogenic cytokines (BMP 2, 4, 7), and angiogenic factors leading to osteoblastic bone formation are being elucidated . The delivery or support of these biochemicals or cellular elements depends on a carrier or scaffolding system. Collagen, hyaluronic acid, calcium phosphate, chitosan, and hydroxyapatite have been studied in the past . Polymer chemistry has yielded polyglycolic acid, polylactic acid, polycaplactone, and combinations such as the copolymer polyglycolic acid–polylactic acid. Although these polymers are biocompatible, their breakdown products are potentially tissue damaging.
The goal is to configure these materials as competent carriers of the biomolecular pro-osteogenics or as supportive scaffolds for cellular proliferation and bone formation . One technique that is showing some promise is three-dimensional printing technology. Three-dimensional complex shapes or structures can be computer generated, constructed in a three-dimensional printer, and then used as protein or cellular carriers for custom implantable bone graft substitutes.
The future
Current avenues of research in molecular biology, progenitor cell use, and biomimetic scaffolds hold promise for the future of bone replacements by defining and employing the complex of stimuli and processes that can result in bone formation. Postnatal progenitor cells have demonstrated the capacity to differentiate into a multitude of cell types . Mesenchymal stem cells can be harvested from bone marrow and demonstrate extensive proliferative ability and the capacity to be guided into bone-forming cell types . Their availability is a limiting factor because their fraction in marrow has been estimated to be as low as 1 in 27,000 cells . Adipose tissue–derived progenitor cells also have been investigated . They possess the advantages of availability and accessibility and have demonstrated capabilities similar to bone marrow–derived cells. In vitro and in vivo studies have demonstrated their ability to form bone .
The molecular processes of the multitude of factors in platelet rich plasma, pro-osteogenic cytokines (BMP 2, 4, 7), and angiogenic factors leading to osteoblastic bone formation are being elucidated . The delivery or support of these biochemicals or cellular elements depends on a carrier or scaffolding system. Collagen, hyaluronic acid, calcium phosphate, chitosan, and hydroxyapatite have been studied in the past . Polymer chemistry has yielded polyglycolic acid, polylactic acid, polycaplactone, and combinations such as the copolymer polyglycolic acid–polylactic acid. Although these polymers are biocompatible, their breakdown products are potentially tissue damaging.
The goal is to configure these materials as competent carriers of the biomolecular pro-osteogenics or as supportive scaffolds for cellular proliferation and bone formation . One technique that is showing some promise is three-dimensional printing technology. Three-dimensional complex shapes or structures can be computer generated, constructed in a three-dimensional printer, and then used as protein or cellular carriers for custom implantable bone graft substitutes.
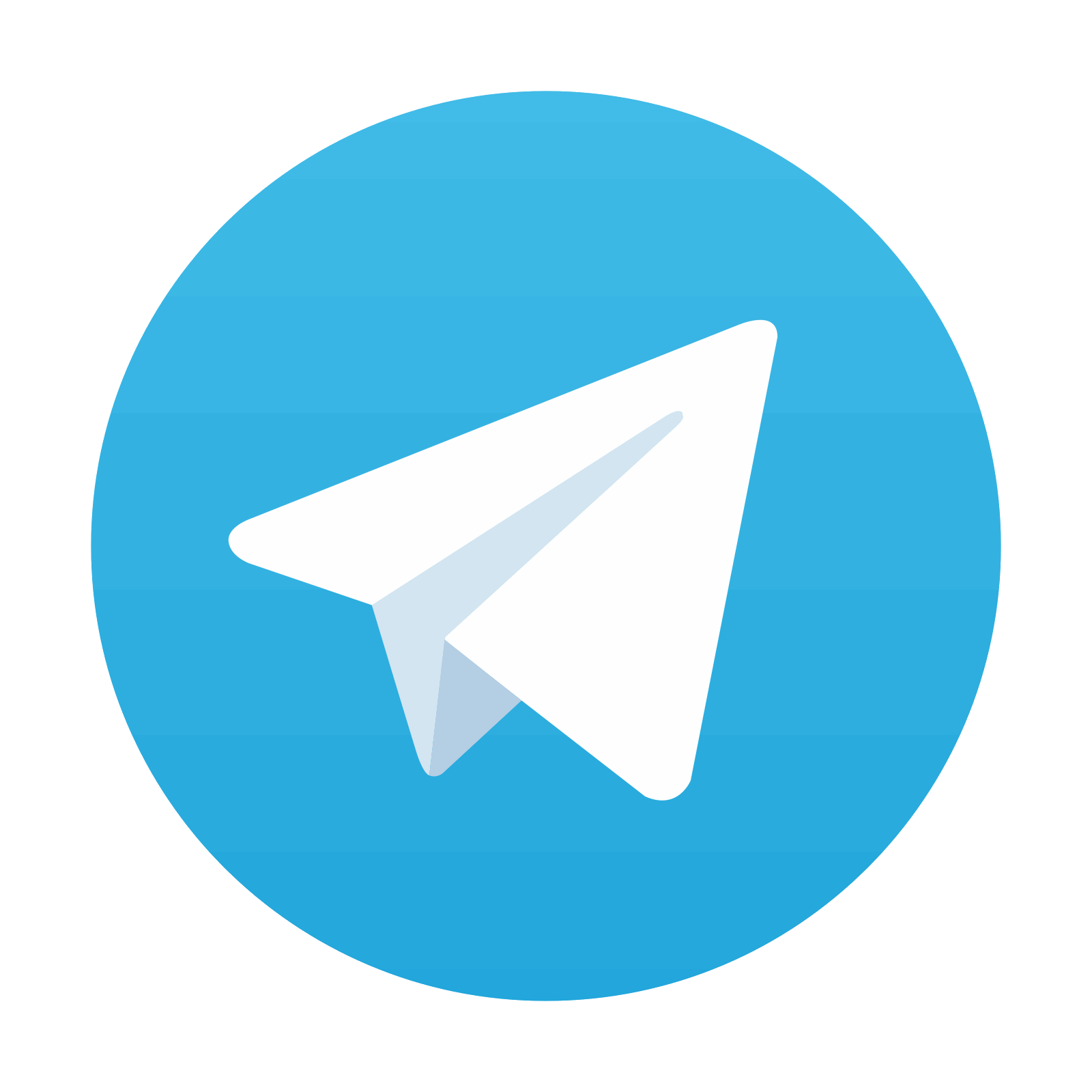
Stay updated, free dental videos. Join our Telegram channel

VIDEdental - Online dental courses
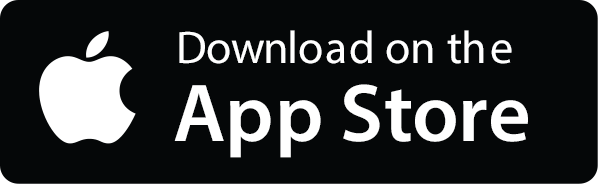
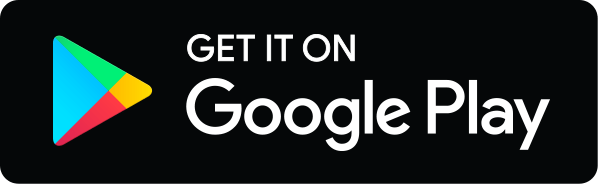