Introduction
This research aimed to measure the bone depth and thickness of different insertion paths for safe placement of infrazygomatic crest miniscrews between the first (U6) and second maxillary molars (U7) by 3-dimensional (3D) reconstruction and to explore their clinical significance.
Methods
Cone-beam computed tomography data from 36 adult orthodontic patients were obtained to generate 3D models (n = 72) of the infrazygomatic crest region. For each model, the bone depth and thickness of 27 different insertion paths were measured in the region between U6 and U7. The relationship between bone depth and thickness was statistically analyzed. The clinical risk for each insertion path was assessed according to the impacts of bone depth and thickness on insertion failure.
Results
Maximum bone depth (median, 7.41 mm; mean, 8.42 mm) was present at 13 mm insertion sites with a gingival tipping angle of 50° and a distal tipping angle of 30°. Maximum bone thickness (median, 3.73 mm; mean, 4.00 mm) was present at 17 mm insertion site with a gingival tipping angle of 70° and a distal tipping angle of 30°. There was a significant negative correlation between bone depth and bone thickness ( r s = −0.569, P <0.001). Failure rates were significantly different among different insertion paths ( P <0.001).
Conclusions
Because the bone depth and thickness may affect the safe insertion of infrazygomatic crest miniscrews in the region between U6 and U7 and they are negatively related, a safe insertion protocol design for distal tooth movement should take both into consideration.
Highlights
- •
We generated 3-dimensional models (n = 72) of the infrazygomatic crest region.
- •
Bone depths and thicknesses of 27 insertion paths of each model were measured.
- •
There was a significant negative correlation between bone depths and bone thicknesses.
- •
A safe insertion protocol should take the bone depth and thickness into consideration.
- •
The clinic risk and proper miniscrew size of each path were provided.
Miniscrews are commonly used for anchorage in distal movement of the maxillary molars for treatment of Class II malocclusion, because of their advantages of minimal anatomic limitation, simple placement, and limited complications. , Placement of miniscrews in the buccal interradicular region is one of the most common approaches used in distal tooth movement. , Although the interradicular miniscrews may reduce complications related to soft tissue irritation, the limited interradicular space can obstruct tooth movement. Therefore, the infrazygomatic crest region is frequently selected for miniscrew insertion, , because it not only has the thickest maxillary cortical bone but also is far from the dentoalveolar region. The anatomic advantages of the infrazygomatic crest region contribute to better primary stability of miniscrews and provide the possibility for unobstructed tooth movement. However, because no well-accepted insertion protocol or sophisticated assistive device has been available for operators, anatomic research is needed for safe miniscrew insertion in this region.
Miniscrew insertion in the infrazygomatic crest region is often restricted by surrounding anatomic structures, like molar roots and maxillary sinus. To provide a clinical guide for miniscrew insertion, Liou et al measured the bone depth from an insertion site to the sinus floor at different gingival tipping angles and insertion heights. They suggested that miniscrews should be inserted at special angles and heights to achieve a bone depth of 6 mm. However, their study only considered the importance of bone depth but ignored the effect of bone thickness at the buccal side of the roots on the safe insertion of miniscrews. The bone thickness related to the diameter of a miniscrew affects not only the mechanical strength of a miniscrew but also the risk for root damage. Reducing the diameter of a miniscrew might decrease the risk of root damage, but the failure rate might increase with decreased mechanical strength. , Therefore, it is necessary to take the bone depth and thickness of each insertion path into account in the evaluation of the clinical risk in infrazygomatic crest miniscrew use.
Moreover, studies have demonstrated that U6 does not seem to be an ideal insertion zone in the infrazygomatic crest region because of its thinner buccal alveolar bone and deeper sinus floor. , Instead, the region between the first (U6) and second maxillary molars (U7) has been suggested as an alternative zone for miniscrew placement.
Currently, cone-beam computed tomography (CBCT) has been commonly used to evaluate the 3-dimensional (3D) craniofacial anatomy and dental morphometrics, because it can provide more objective data about anatomic structures and thus result in more accurate measurements than two-dimensional radiography.
Therefore, this study aimed to explore how the bone depths and thicknesses of different insertion paths in the region between U6 and U7 may affect the infrazygomatic crest miniscrew use by 3D reconstruction models because of CBCT data.
Material and methods
CBCT data from 36 adult orthodontic patients (16 men and 20 women; mean age, 23.1 years; range, 20-28 years) were obtained from the Department of Orthodontics in the Third Affiliated Hospital of Xinxiang Medical University, Xinxiang, Henan, China. The CBCT data included for this study met the following criteria: (1) no missing (except the third molar), supernumerary or defective tooth in the maxillary posterior-tooth area; (2) no radiographic signs of periodontal disease or severe crowding of the dental arch; (3) no craniofacial anomaly or systemic disease; (4) no history of orthodontic treatment. Informed consent signed by the patients was waived because of the observational nature of the study and the anonymity and deidentification of personal information before data analysis. This study was approved by the medical ethical committee of the Third Affiliated Hospital of Xinxiang Medical University.
The CBCT images, taken with KaVo 3D eXam i (KaVo Dental, Biberach, Germany) at these settings: 5 mA, 120 kV, exposure time of 6 seconds, voxel size of 0.2 mm, axial slice thickness of 0.2 mm, and scanning area of 140 mm × 85 mm, were saved in Digital Imaging and Communications in Medicine format and reconstructed with continuous slices at 0.2-mm thickness using Mimics 14.01 software (Materialise, Leuven, Belgium). For each patient, two 3D models were reconstructed for bilateral infrazygomatic crest regions. Altogether, 72 models were produced for the 36 patients.
Geomagic Studio 12.0 (3D Systems, Morrisville, NC) was used to create insertion paths by constructing characteristic, and reference planes in the 3D reconstructed models. The 5 characteristic planes (buccal root plane, distal plane of U6, mesial plane of U7, alveolar bone plane, and bisection plane of U6 and U7) were constructed by self-contained functions of the software. In detail, using the Best Fit function, the part of the buccal surface above the root furcation plane (parallel to the posterior occlusal plane and through the root furcation point) of the distobuccal root of U6 (DR6) and the mesiobuccal root of U7 (MR7) was selected to fit the buccal root plane ( Fig 1 , A and D ). Using the same function, the part of the distal surface above the root furcation plane of DR6 and the part of the mesial surface above the root furcation plane of MR7 was used to fit the distal plane of U6 and the mesial plane of U7, respectively ( Fig 1 , B and D ). Similarly, the part of alveolar bone from 11 mm to 19 mm above the posterior occlusal plane (POP) between the distal plane of U6 and the mesial plane of U7 was selected to fit the alveolar bone plane ( Fig 1 , C and E ). Using the 2-Plane Average function, the bisection plane of U6 and U7 was constructed by averaging the distal plane of U6 and the mesial plane of U7 ( Fig 1 , D ). In this study, the POP was used to represent the horizontal reference plane and a plane that was at a right angle to the horizontal plane and the alveolar bone plane through the contact point between the crowns of U6 and U7 was used to represent the vertical reference plane ( Fig 1 , F ).

In each of the 3D models, 27 different insertion paths were created at 3 insertion heights, 3 gingival tipping angles, and 3 distal tipping angles. Because of the characteristic and reference planes, 3 insertion sites corresponding to the midpoints between the roots of U6 and U7 were designed at heights of 13, 15, and 17 mm above the horizontal reference plane on the buccal alveolar bone ( Fig 2 , A ). At each insertion site, 2 measuring planes were designed parallel to the horizontal and vertical reference planes, respectively ( Fig 2 , B ). Then, 2 perpendicular lines of the horizontal and vertical reference planes at each insertion site were constructed as a vertical axis and a horizontal axis, respectively ( Fig 2 , C ). The 3 distal tipping angles were obtained by rotating the vertical measuring plane by 0°, 15°, and 30° distally around the vertical axis, respectively ( Fig 2 , D ). Similarly, the 3 gingival tipping angles were obtained by rotating the horizontal measuring plane by 50°, 60°, and 70° gingivally around the horizontal axis, respectively ( Fig 2 , E ). Finally, 9 insertion paths at each insertion site were obtained. The paths connected 1 specific insertion site with 9 intersection points where the 3 rotated vertical measuring planes and the 3 rotated horizontal measuring planes met at the bottom of the maxillary sinus ( Fig 2 , F ). Hence, 3 insertion sites at different heights produced 27 insertion paths in one specific 3D model.

To our knowledge, 1.6 mm is the minimum diameter recommended to avoid miniscrew fracture in dense cortical bone, 3.8 mm the minimum effective length (length in bone) recommended for miniscrew stability, and 0.5 mm the minimal safety distance from a miniscrew to any adjacent anatomic structure. Therefore, insertion failure in this study was defined as an effective length of miniscrew (length in bone) less than 3.8 mm ( Fig 3 , A ) or a minimum distance less than 0.5 mm from a 1.6 mm miniscrew to roots ( Fig 3 , B ) during the distal tooth movement. Bone depth and thickness of each insertion path were measured respectively as 2 indexes closely related. The bone depth was defined as the distance from an insertion site to the intersection point at the bottom of the maxillary sinus ( Fig 4 , A ), whereas the bone thickness as the radius of a cylinder with an insertion path as its axis, tangent to the distal movement trajectory of roots ( Fig 4 , B ). If insertion path passed below the distal movement trajectory of roots, the value of bone thickness was 0. In this study, the maximum distal movement of molar roots was designed as 3.2 mm. We simulated the distal movement at a trajectory from 0.0 mm to 0.8 mm to 1.6 mm to 2.4 mm and 3.2 mm.


In short, an insertion failure might arise in a path when its bone depth is less than 3.8 mm or its bone thickness less than 1.3 mm (miniscrew with 0.8 mm radius added the 0.5 mm minimal safety distance). The insertion failure rate for each insertion path was calculated according to its bone depth and thickness.
To determine whether the sample size was sufficient, CBCT data from 20 adult orthodontic patients were collected in the pilot study, and the bone depths and thickness of forty 3D models (20 people, each with left and right sides) were measured using the same method described above. Finally, CBCT data from 36 adult orthodontic patients were obtained, and the bone depths and thickness of seventy-two 3D models (36 people, each with left and right sides) were measured in this study. Of all the 3D models measured by a rater, 16 were selected randomly by another rater for reevaluation in the same way.
Statistical analysis
Statistical analysis was conducted with SPSS software (version 20; IBM, Armonk, NY). A level of P <0.05 was considered statistically significant. We tested the normal distribution using the Shapiro-Wilk test and found that the measurements were not normally distributed.
Wilcoxon signed-rank test was used to analyze differences between measurements of the left and the right sides. Mann-Whitney U test was used to analyze differences between measurements of the males and females. For each measurement index, multiple comparisons were made only between variables of the same category, whereas those of the other 2 categories were controlled. Friedman test was used to evaluate the differences in bone depths and thicknesses of different paths, and Cochran’s Q test to analyze the insertion failure rates of different paths. The significant analysis was followed by the post-hoc test with the Bonferroni correction. Correlation between bone depths and bone thicknesses was analyzed by Spearman’s rank correlation coefficient. Interrater reliability for measurement was examined using the interclass correlation coefficient.
In our pilot study, bone depths and thicknesses of forty 3D models from 20 patients were measured 27 times at different insertion paths. For a 2-sided hypothesis test, 1080 measurements (multiplying 40 × 27) could yield a 100% power to detect a Spearman correlation of −0.51 between the depths and thicknesses with a significance level (α) of 0.05. Therefore, seventy-two 3D models of infrazygomatic crest region from 36 patients in our study were sufficient for statistical analysis.
Results
Because no statistically significant differences were found between different sides ( P >0.05) or genders ( P >0.05), the following evaluations were performed on the pooled data without any distinction in laterality or gender.
Statistical analysis showed that the interclass correlation coefficient was 0.942 ( P <0.001) and 0.936 ( P <0.001) respectively for bone depths and bone thicknesses, demonstrating a high reliability of measurements in this study.
This study found that the bone depths of different insertion paths were significantly different ( P <0.001). Significant differences in bone depth were observed between any 2 insertion heights at the same insertion angle ( P <0.001). From 13 mm to 15 mm and 17 mm, the bone depths decreased significantly. Regarding the gingival tipping angle, only at 13 mm insertion height and distal tipping angle of 30°, a significantly greater bone depth was observed at 50° than 60° ( P <0.001) and 70° ( P <0.001). In the other paths, significantly greater bone depths were observed at 70° than 60° ( P <0.05). Although the bone depth increased with the increased distal tipping angle at the same insertion height and gingival tipping angle, no significant difference in bone depth existed among the 3 distal tipping angles at 15 mm insertion height and gingival tipping angle of 70° ( P = 0.067). In the other paths, significantly greater bone depths were observed at 30° than 0° ( P <0.05) and 15° ( P <0.05). In Table I , bone depths of different paths were expressed as median (25th-75th percentile) and mean ± standard deviation. The maximum bone depth (median, 7.41 mm; mean, 8.42 mm) was present at a disposition of 13 mm insertion site, gingival tipping angle of 50°, and distal tipping angle of 30°.
Angles | Insertion sites at different heights | ||||||
---|---|---|---|---|---|---|---|
Occlusogingival direction | Mesiodistal direction | 13 mm (a1-i1) | 15 mm (a2-i2) | 17 mm (a3-i3) | |||
Median (25th-75th) | Mean ± SD | Median (25th-75th) | Mean ± SD | Median (25th-75th) | Mean ± SD | ||
50 ° | 0° (a) | 6.70 (5.16–8.47) a2,a3,d1,c1 | 8.00 ± 4.54 | 5.01 (3.60–6.57) a1,a3,c2,g2 | 5.72 ± 2.92 | 3.49 (2.41–4.94) a1,a2,c3,g3 | 4.04 ± 2.32 |
15° (b) | 6.87 (5.25–9.03) b2,b3,e1,c1 | 7.82 ± 3.85 | 5.15 (3.70–6.61) b1,b3,c2,h2 | 5.76 ± 2.96 | 3.64 (2.44–4.86) b1,b2,c3,h3 | 4.11 ± 2.31 | |
30° (c) | 7.41 (5.76–9.35) c2,c3,f1,i1,a1,b1 | 8.42 ± 4.10 | 5.52 (4.05–7.15) c1,c3,f2,b2,a2 | 6.34 ± 3.64 | 3.91 (2.57–5.36) c1,c2,a3,b3 | 4.38 ± 2.45 | |
60 ° | 0° (d) | 6.56 (5.29–8.24) d2,d3,f1,a1,g1 | 7.16 ± 2.61 | 5.02 (3.82–6.39) d1,d3,f2,g2 | 5.52 ± 2.38 | 3.53 (2.53–4.77) d1,d2,f3,g3 | 4.02 ± 2.12 |
15° (e) | 6.68 (5.29–8.32) e2,e3,f1,b1,h1 | 7.19 ± 2.56 | 5.13 (3.80–6.45) e1,e3,f2,h2 | 5.54 ± 2.34 | 3.62 (2.51–4.91) e1,e2,f3,h3 | 4.05 ± 2.07 | |
30° (f) | 6.94 (5.55–8.53) f2,f3,c1,d1,e1 | 7.45 ± 2.62 | 5.37 (3.85–6.69) f1,f3,d2,e2,c2,i2 | 5.75 ± 2.41 | 3.72 (2.54–4.92) f1,f2,i3,d3,e3 | 4.20 ± 2.16 | |
70 ° | 0° (g) | 6.81 (5.56–8.34) g2,g3,d1,i1 | 7.33 ± 2.44 | 5.23 (4.02–6.74) g1,g3,a2,d2 | 5.73 ± 2.28 | 3.75 (2.67–5.07) g1,g2,a3,d3,i3 | 4.24 ± 2.08 |
15° (h) | 6.97 (5.56–8.26) h2,h3,e1,i1 | 7.31 ± 2.39 | 5.22 (4.07–6.65) h1,h3,b2,e2 | 5.72 ± 2.24 | 3.76 (2.68–5.11) h1,h2,b3,e3,i3 | 4.26 ± 2.09 | |
30° (i) | 7.02 (5.63–8.30) i2,i3,c1,g1,h1 | 7.40 ± 2.40 | 5.35 (4.05–6.75) i1,i3,f2 | 5.80 ± 2.27 | 3.87 (2.69–5.21) i1,i2,f3,g3,h3 | 4.32 ± 2.10 |
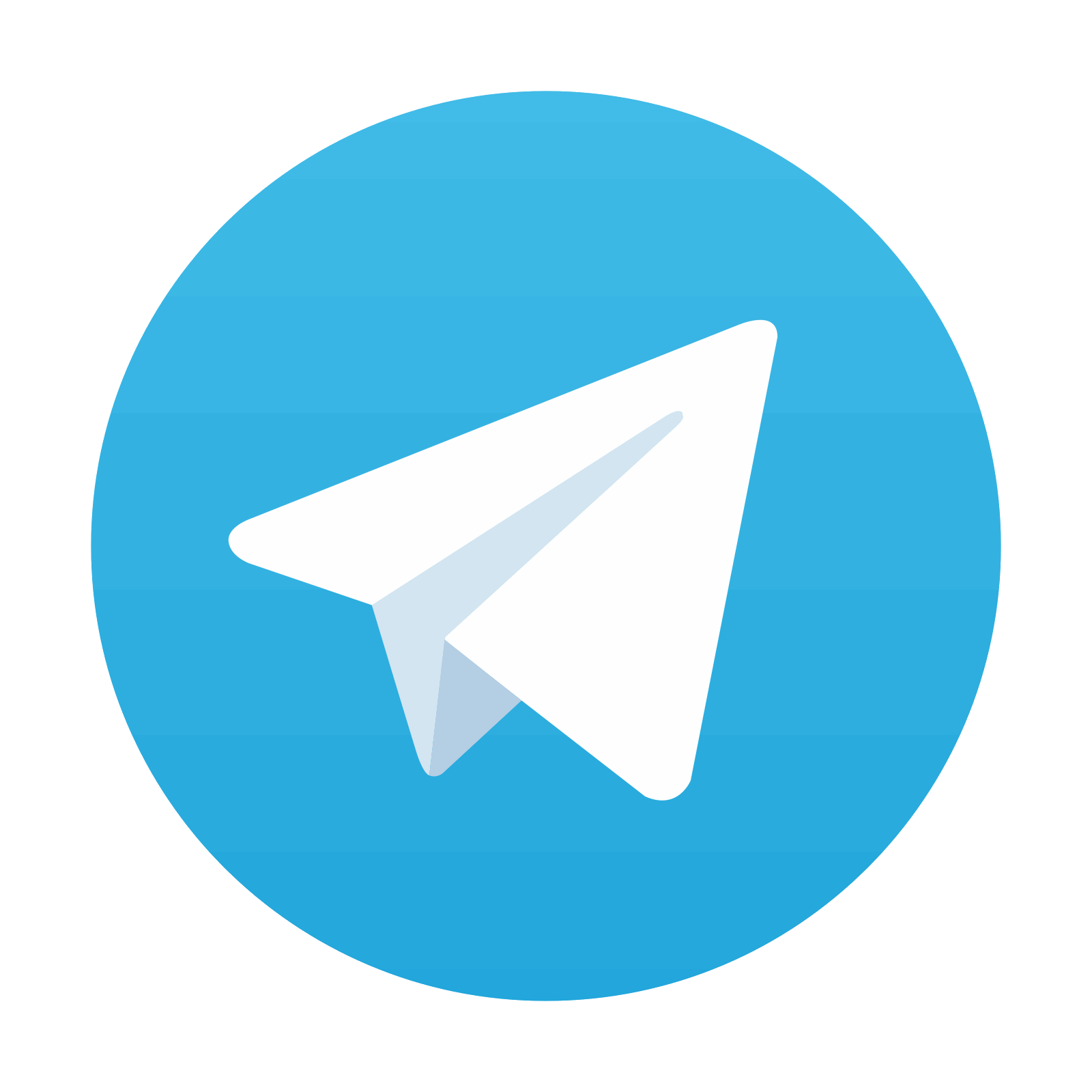
Stay updated, free dental videos. Join our Telegram channel

VIDEdental - Online dental courses
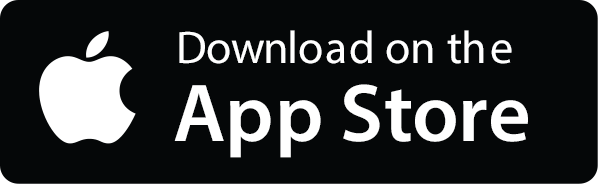
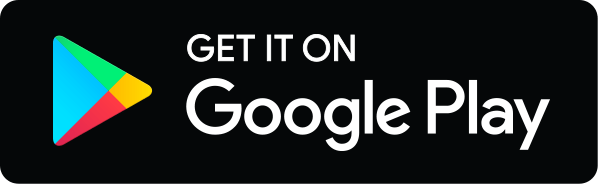
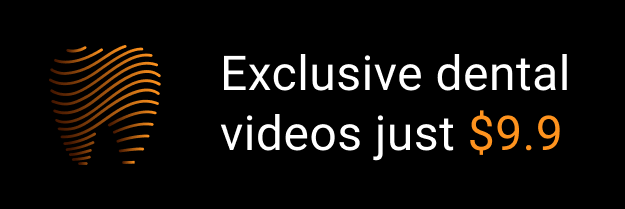