Abstract
Laminin-1 has been reported as one of the factors responsible for the nucleation of calcium phosphates and, in vitro , has been reported to selectively recruit osteoprogenitors. This article focused on its in vivo effects, and evaluated the effect of laminin-1 local application on osseointegration. Polished cylindrical hydroxyapatite implants were coated with laminin-1 (test) and the bone responses in the rabbit tibiae after 2 and 4 weeks were evaluated and compared to the non-coated implants (control). Before the samples were processed for histological sectioning, they were three-dimensionally analysed with micro computed tomography (μCT). Both evaluation methods were analysed with regards to bone area around the implant and bone to implant contact. From the histologic observation, new bone formation around the laminin-1 coated implant at 2 weeks seemed to have increased the amount of supporting bone around the implant, however, at 4 weeks, the two groups presented no notable differences. The two-dimensional and three-dimensional morphometric evaluation revealed that both histologic and three-dimensional analysis showed some tendency in favour of the test group implants, however there was no statistical significance between the test and control group results.
Osseointegration is defined as the intimate contact between biomaterials to bone, nowadays, most commonly between titanium, zirconia or other biocompatible materials. The concept has been applied to dental implants, hip prostheses, external hearing aids, and many others where a stable and functional restoration is required. In recent years, attempts to enhance the bioactivity of the materials have opened the door to further improvements of the osseointegration cascade. The term ‘bioactive’ can be defined as a material that interacts and stimulates protein and growth factors involved in the tissue healing process. This is especially true during the initial stages of osseointegration, where the constant interaction and attachment of different extracellular matrix proteins to the surface is a necessity for the subsequent biological events. These include the formation of fibrin networks, platelet and growth factor trapping, chemotaxis of osteoprogenitors, and the proliferation and differentiation of specific cells. This has been demonstrated with implant surfaces coated with fibronectin, one of the most common extra cellular matrix proteins, the osteogenic cells responded quickly to its presence and inducted their migration. In addition, the migrated cells seemed to have high proliferation and differentiation kinetics. It is strongly suggested that attracting or applying these bioactive proteins to the implant surface may be an effective alternative in achieving enhanced osseointegration.
Among the numerous varieties of extracellular matrix proteins, laminin is a unique heterotrimeric glycoprotein found in the basal lamina that contains the arginine–glycine–aspartic acid (RGD) sequence. One of the domain types, the laminin-1 (α1β1γ1), is the predominant glycoprotein in the basement membrane, which in the authors’ previous investigation presented increased calcium phosphate (CaP) precipitation on alkaline heat treated titanium discs in simulated body fluid (SBF). The authors hypothesized that the application of laminin-1 to implant surfaces could have significant effects on the initial stages of osseointegration, creating a foundation for further osteogenesis around the implant relative to uncoated control groups.
Materials and methods
Hydroxyapatite implant preparation and laminin coating
In order to minimize the effect of surface roughness in early osseointegration stages and to minimize the amount of scattering halation from the μCT analysis, non-threaded, polished hydroxyapatite implants (diameter 4.2 mm, length 9.0 mm) were prepared for this study ( Fig. 1 a). The hydroxyapatite powder (Plasma Biotal, UK) was processed by ball milling. The suspension was freeze granulated and the granules were cold isostatically pressed at 200 MPa to prepare powder compacts. A CAD/CAM procedure was used to transform the powder compacts to the desired shape of the implants. When sintered at 1250 °C for 2 h a density above 98% was obtained, and no other phases than hydroxyapatite were detected in the X-ray diffraction pattern. The surface of the sintered implants was polished using diamond paste. The surface roughness was characterized by interferometer (MicroXam; ADE Phase Shift, Inc., Tucson, AZ) and the mean S a value was 0.08 μm.

Based on the authors’ previous studies, laminin-1 (Sigma–Aldrich, L2020, Stockholm, Sweden) was diluted to a concentration of 100 μg/ml in Dulbecco’s phosphate buffered saline (DPBS) without CaCl 2 or MgCl 2 (GIBCO, Invitrogen Corporation, 14190-094, Grand Island, NY, USA). One hour prior to animal surgery, half of the ceramic implants were incubated at room temperature in 48 well plates (Nunclon Surface, Nunc, Roskilde, Denmark) containing 250 μl per well of the laminin-1 solution.
Ellipsometry was used to estimate the amount of adsorbed laminin-1 on optically smooth titanium surfaces similar to that of the polished experimental implants. The descriptive methodology can be found in a study by Linderback et al. In brief, cleaned SiO 2 surfaces were placed in an evaporation chamber with final pressure below 1 × 10 −8 Torr. Approximately 200 nm of titanium was physical vapour deposited (PVD), and spontaneously oxidized at room conditions.
Thereafter, the prepared surfaces were fixed in the ellipsometric cuvette filled with PBS at room temperature. Angles Δ 0 and Ψ 0 were measured with a Rudolph Research AutoEL III ellipsometer operating in a wavelength of 632.8 nm at a 70° angle of incidence. The cuvette was emptied and filled with laminin-1 solution and new angles Δ and Ψ were calculated. The thickness of the adsorbed protein was estimated to be 26 Å using the MacCrackin algorithm.
Surgical procedures
14 male lop-eared rabbits (mean body weight 4.0 kg, n = 7 for each time point) were used for the study. One test (laminin-1 coated) implant and one control (non-coated) implant was inserted into the left and right tibial metaphysis, respectively, into a drilled hole with the final drill diameter of 4.2 mm. In order to stabilize the non-threaded, smooth implants, the implants were inserted bicortically and were covered with a titanium membrane and were pinned down using bone tacks (Friadent GmbH, Mannheim, Germany) ( Fig. 1 b). The planned animal study was approved by the Malmö/Lund regional animal ethics committee (approval number: M282-09).
Before surgery, the legs were shaved and disinfected with 70% ethanol and 70% chlorhexidine. The animals were anesthetized with intramuscular injections of a mixture of 0.15 ml/kg medetomidine (1 mg/ml Dormitor; Orion Pharma, Sollentuna, Sweden) and 0.35 ml/kg ketamine hydrochloride (50 mg/ml Ketalar; Pfizer AB, Sollentuna, Sweden). Lidocaine hydrochloride (Xylocaine; AstraZeneca AB, Södertälje, Sweden) was administrated as the local anaesthetic at each insertion site at a dose of 1 ml. Postoperatively, buprenorphine hydrochloride (0.5 ml Temgesic; Reckitt Benckiser, Slough, UK) was administered as an analgesic for 3 days.
After 2 and 4 weeks, the rabbits were killed with an overdose (60 mg/ml) of pentobarbitalnatrium (Apoteksbolaget AB, Stockholm, Sweden). The implants were removed en bloc and immersed in 4% neutral buffered formaldehyde. After fixation, the samples remained in 70% ethanol for the micro computed tomography analysis.
Micro computed tomography
The 3 dimensional (3D) bone formation around the implant was examined using micro computed tomography (μCT 40, Scanco Medical, Basserdorf, Germany) with a slice resolution of 20 μm. 500 μCT slices were imaged at an X-ray energy level of 55 kVp, and a current of 145 μA. Integration time was 200 ms with a total scanning time of 45.4 min (160 mA s). All data were exported in DICOM format and imported into Amira software (Visage Imaging GmbH, Berlin, Germany) for evaluation. The data were cropped along the implant axis to where the cortical bone started to exclude unnecessary information above the cortical bone.
Before segmentation, threshold levels for bone and implant were determined, based on the complete slices. This was done by determining the upper and lower threshold levels for bone and implant. Threshold determination was repeated for intra- and inter-examiner repeatability evaluation.
First, a region of interest (ROI) was defined, where the bone area (BA) was calculated. Thus, implants were selected based on their threshold level and this region was circumferentially expanded, creating a 0.20 mm zone around the implant. Second, the implant and bone in the ROI were differentiated based on their threshold levels, creating three volumes: implant, bone and soft tissue. These were converted into a tetrahedral grid from triangular surfaces, to make area and volume measurements possible. Outcome variables were BA, which is the percentage of bone that is present in the region around the implant, and bone implant contact (BIC), the area-percentage of the total implant surface that is covered by bone.
Ground section preparation and histology
After the μCT analysis, 4 paired samples from each group, and each time point, were randomly selected for undecalcified ground sectioning. In brief, after a series of dehydration and infiltration in resin, the samples were embedded in light-curing resin (Technovit 7200 VLC; Heraeus Kulzer Wehrheim, Germany). Thereafter, one central undecalcified cut and ground section was prepared from each implant with the Exakt sawing and grinding equipment. The sections were ground to a final thickness of about 15 μm and stained with toluidine blue and pyronin.
Histological evaluations were performed using a light microscope (Eclipse ME600, Nikon, Japan) and histomorphometrical data were analyzed using image analyzing software (Image J, National Institute for Health, USA). BIC percentage was calculated along the entire implant and calculated with 10× objective magnification. BA percentage was calculated inside a defined ROI rectangular area drawn with the implant surface as base and with a height of 200 μm (BA (%): (area of bone)/(ROI) × 100).
Statistical analysis
The statistical significance for the differences was evaluated with the Mann–Whitney U -test with the significance level set at p ≤ 0.05. All statistical calculations were performed using SPSS v19 (SPSS Inc., Chicago, IL, USA) software.
Results
Histological analysis
After 2 weeks, the histological specimens showed that new bone formation was more evident for the laminin-1 coated implants compared to the non-coated implants ( Fig. 2 a). The mean percentage (SD) calculated for the BIC at 2 weeks was 36.6% (10.5%) for the test implants compared to 20.4% (20.2%) for the control implants. There was no statistical significance between both groups ( p = 0.248). At 4 weeks, the BIC was 45.9% (15.2%) for the test implants compared to 39.2% (12.9%) for the control implants. No statistically significant differences could be seen ( p = 0.386).

The mean BA % (SD) at 2 weeks was 33.6% (9.0%) for the test implants compared to 27.6% (11.9%) for the control implants ( Fig. 3 b), a difference which was not statistically significant ( p = 0.309). At 4 weeks, the BA was 49.6% (15.9%) for the test implants compared to 44.7% (15.6%) for the control implants ( Fig. 2 b). No statistically significant differences could be seen ( p = 0.564).

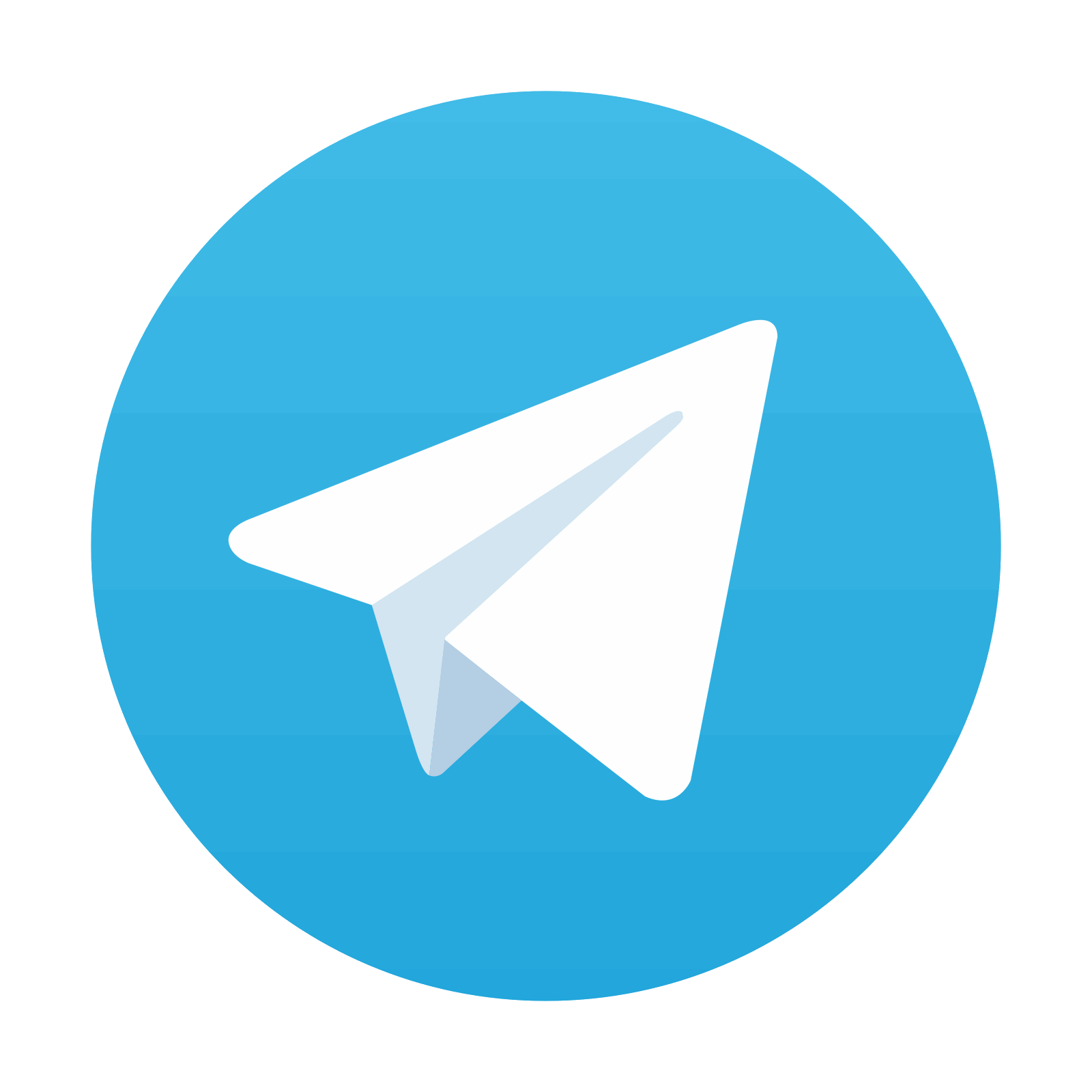
Stay updated, free dental videos. Join our Telegram channel

VIDEdental - Online dental courses
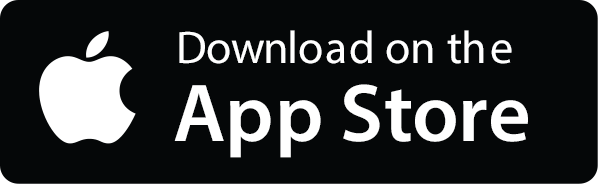
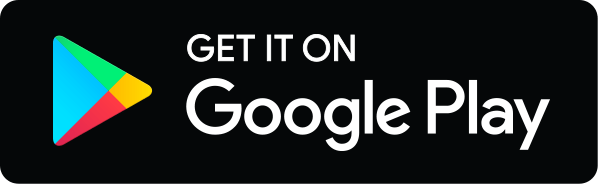
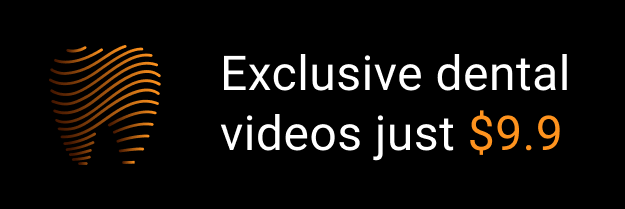