Graphical abstract

Abstract
Objectives
This study examined the bonding performance and dentin remineralization potential of an experimental adhesive containing calcium-phosphate (Ca/P) micro-fillers, and self-etching primers doped with phosphoprotein biomimetic analogs (polyacrylic acid-(PAA) and/or sodium trimetaphosphate-(TMP)).
Methods
Experimental self-etching primers doped with biomimetic analogs (PAA and/or TMP), and an adhesive containing Ca 2+ , PO 4 −3 -releasing micro-fillers (Ca/P) were formulated. Sound human dentin specimens were bonded and cut into sticks after aging (24 h or 6 months) under simulated pulpal pressure (20 cm H 2 O), and tested for microtensile bond strength (μTBS). Results were analyzed using two-way ANOVA and Tukey’s test (p < 0.05). Interfacial silver nanoleakage was assessed using SEM. Remineralization of EDTA-demineralized dentin was assessed through FTIR and TEM ultrastructural analysis.
Results
Application of the Ca/P-doped adhesive with or without dentin pre-treatments with the primer containing both biomimetic analogs (PAA and TMP) promoted stable μTBS over 6 months. Conversely, μTBS of the control primer and filler-free adhesive significantly decreased after 6 months. Nanoleakage decreased within the resin-dentin interfaces created using the Ca/P-doped adhesives. EDTA-demineralized dentin specimens treated the Ca/P-doped adhesive and the primer containing PAA and TMP showed phosphate uptake (FTIR analysis), as well as deposition of needle-like crystallites at intrafibrillar level (TEM analysis).
Significance
The use of Ca/P-doped self-etching adhesives applied in combination with analogs of phosphoproteins provides durable resin-dentin bonds. This approach may represent a suitable bonding strategy for remineralization of intrafibrillar dentin collagen within the resin-dentin interface.
1
Introduction
Dental adhesive systems (DBSs) are essentially a mixture of volatile solvents (e.g. H 2 O, alcohols, acetone), hydrophilic and hydrophobic monomers, which infiltrate demineralized dental substrates (e.g. dentin and enamel) and polymerize in situ to create a micromechanical bond for restorative dental resin composites to dentin . Depending on their composition, DBSs may be applied via an etch-and-rinse or a self-etch bonding approach . Although recent improvements have been made in the composition of new generation of etch-and-rinse adhesives (ERAs) and self-etch adhesives (SEAs), their long-term dentin bonding performance remain questionable . That is, the stability of the resin-dentin interface may be jeopardized by three types of degradation processes : i) Enzymatic-mediated (e.g. matrix-metalloproteinases-(MMPs) and cysteine cathepsins-(CTPs)) dentin collagen degradation ; ii) hydrolysis of the adhesive polymeric matrix . This latter type of degradation is much slower compared to the proteolysis of dentin collagen fibrils, although several esterases can accelerate the breakdown of ester bonds in (poly)methacrylates. iii) Hydrolysis of the silane-coupling molecule (Si-O-Si) may also occur and cause debonding of the fillers from the polymer matrix .
Most researchers agree that hybrid layers created by ERAs, in particular those with simplified bonding approaches may degrade quicker than those generated using SEAs. This seems mainly due to the use of (35–40%) phosphoric acid (PA), which demineralizes dentin deeper and more completely compared to SEAs. The low pH (∼0.4) of PA causes complete demineralization of collagen fibrils, which activates all of their endogenous proteases and makes them more susceptible to hydrolytic degradation . Conversely, with the use of milder SEAs, demineralization and resin-infiltration occurs simultaneously. Their milder acidic primer (pH: >1.6) does not totally expose the collagen fibrils, but leaves collagen fibrils protected by intrafibrillar apatite crystallites within the dentinal matrix and smear plugs inside dentinal tubules. The presence of smear plugs inside dentin tubules reduces the risk of dentinal fluid permeability from tubules into the demineralized matrix during resin infiltration . However, although resin-dentin interfaces created using SEAs might require “less attention” in terms of biomimetic remineralization compared to those created using ERAs, it is important to consider that dentin bonded using SEAs can be still affected by collagen enzyme-mediated degradation, since self-etching primers can also activate these enzymes . Indeed, significant enzyme-mediated degradation of resin-dentin hybrid layer has been demonstrated , suggesting that the activation of the dentinal enzymes may occur via a two-step process. This process starts with the initial demineralization and exposure of collagen fibrils, and then the acidity of bonding systems (self-etch primers) may cause secondary enzyme activation due to their lower degree of polymerization at the hybrid layer . There is also evidence that the proteolytic and gelatinolytic activity that destroys collagen fibrils, begins at the bottom of the hybrid layer, in particular if this latter is not well infiltrated by resin monomers and properly polymerized . Hence, as the bottom half of the hybrid layer degrades, the MMPs spread into the upper regions of the resin-bonded dentin, and increase the porosities within the hybrid layer .
It is critical that clinicians understand why resin dentin bonds created both with ERAs and SEAs have poor durability. The reasons for that poor durability are both biochemical and biomechanical . Indeed, after etching with phosphoric acid and rinsing with water, the water-saturated demineralized dentin have a residual water content of 76 vol% . The etching acid also activates all proforms of MMPs and cysteine cathepsins in the matrix. These enzymes are hydrolases. That is, they add free water across specific peptide bond to cleave the biopolymer into soluble fragment; that results in degradation of hybrid layers. The stiffness of water-saturated demineralized dentin is about 0.1 MPa, while that of resin-infiltrated demineralized dentin is about 3–4 GPa because the collagen fibrils are surrounded by resin monomers that polymerize in loco during light-curing procedures . Mineralized or remineralized dentin at the resin-dentin interface contains little free water. In the absence of free water, the intrinsic hydrolases of the dentin matrix are inactivated. Thus, mineralization is a form of dehydration as apatite nanocrystals physically displace free water as they slowly stiffen dentin.
Resin composites have stiffness 16–18 GPa, while the underlying mineralized dentin has a stiffness of 18–20 GPa . When the resin-bonded interface is loaded with compressive stress, the highest stress-concentrations develops at the site of the lowest stiffness. This causes the hybrid layer to undergo cyclic strain during mastication. That cyclic strain can cause fatigue, which ultimately destroys the hybrid layer.
In ideal therapeutic resin composites, the low stiffness of hybrid layers would slowly undergo remineralization by therapeutic resin composites. If all of the layers in the bonded interfaces had the same high degree of stiffness, there would be a reduced amount of stress-concentration within the interface and less fatigue processes . Additionally, such remineralized hybrid layers would not contain enough free water to allow endogenous proteases to hydrolyze collagen.
The bonding longevity of the resin-dentin interface may be enhanced by means of therapeutic remineralization of mineral-depleted dentin collagen within the hybrid layer . The use of biomimetic analogs of dentin phosphoproteins (e.g. polyacrylic acid-(PAA)) and/or sodium trimetaphosphate-(TMP)), in combination with resin-based materials that can release ionized calcium (Ca 2+ ) and phosphate (PO 4 −3 ) ions in a neutral to alkaline water-rich environment, may induce intrafibrillar collagen remineralization . It has been demonstrated that if demineralized dentin is covered with a flowable resin composite containing amorphous calcium phosphate (ACP), and immersed in biomimetic polyanions that can slowly diffuse through any water-filled porosities within adhesives and hybrid layers (i.e. residual water in un-infiltrated dentin), it would be possible to “back-fill” such defects with apatite crystals and fossilize all dentin proteases as the matrix collagen remineralize . As was described above, self-etching adhesives do not demineralize dentin as deeply as etch-and-rinse adhesives; greater mineral content and much lower residual water contents are present in such circumstances. Nevertheless, there is no published information about the potential biomimetic remineralization effect of phosphoproteins biomimetic analogs used in combination with self-etching adhesives containing specific calcium phosphates.
Thus, the objective of this in vitro study was to evaluate the bonding performance of an experimental remineralizing self-etching adhesive containing micro-fillers of reactive calcium phosphate (Ca/P), and a primer doped with phosphoproteins biomimetic analogs [polyacrylic acid-(PAA): and/or sodium trimetaphosphate-(TMP)].
The first hypothesis tested in this study was that the use of a self-etching primer doped with PAA and/or TMP could increase the durability of dentin-bonded specimens created with an ion-releasing adhesive containing Ca/P, and submitted to prolonged aging (6 months) under simulated pulpal pressure. The second hypothesis was that the use of a self-etching primer doped with PAA and/or TMP in combination with the ion-releasing adhesive containing Ca/P could induce reliable biomimetic remineralization of fully EDTA-demineralized dentin collagen fibrils.
2
Materials and methods
2.1
Experimental adhesives
An experimental resin-based solution was prepared and used as a control mildly acidic primer (P-CTR) by blending 20 wt% glycerol-dimethacrylate-phosphate (GDMA-P), 10 wt% hydroxyethyl-methacrylate (HEMA), 15 wt% urethane-dimethacrylate (UDMA), 5 wt% triethyleneglycol-dimethacrylate (TEGDMA), 20 wt% de-ionized water and 30 wt% absolute ethanol. Using this control primer, three additional experimental primers were formulated by adding the biomimetic analogs PAA and/or TMP: i) 10 wt% polyacrylic acid (P-PAA, Mw 1800); ii) 10 wt% sodium trimetaphosphate (P-TMP); iii) 5 wt% PAA + 5 wt% TMP (P-PAA/TMP) . The pHs of the primers were all adjusted to 2.1 using NaOH.
A further experimental co-monomer blend was prepared and used as a control adhesive (R-CTR) resin by mixing 30 wt% UDMA, 25 wt% TEGDMA, 20 wt% GDMA-P, 17 wt% ethoxylated bisphenol-A-diglycidyl-dimethacrylate (Bis-EMA), 5 wt% bisphenol-A-diglycidyl-dimethacrylate (Bis-GMA), 1 wt% ethyl 4-dimethylamine benzoate, 0.5 wt% canphoroquinone and 1.5 wt% diphenyliodonium hexafluorophosphate. The control R-CTR was then used to formulate the experimental ion releasing adhesive (R-IR). This latter was created by adding 20 wt% Ca/P micro-filler (10–50 μm particle size) constituted of a mixture of monocalcium phosphate monohydrate (MCPM) (45 mol%), beta-tricalcium phosphate (β-TCP) (45 mol%) and Ca(OH) 2 (10 mol%). All resin blends created in this study were stirred for 1 h under continuous sonication in order to obtain homogenous solutions.
2.2
FTIR-ATR analysis
Six sound human third molars were obtained under a protocol (038/2014) approved by the institutional Research Ethics Committee (REC) for medical investigations. The teeth were stored at 4 °C in 0.5% Chloramine-T up to 30 days before use. The enamel was removed to expose mid-coronal dentin and twenty-five dentin sticks (7 mm × 2 mm × 1 mm) were obtained from each tooth using a hard tissue microtome (Isomet, Buehler Ltd., Lake Bluff, USA) equipped with a slow-speed water-cooled diamond wafering saw. These sticks were completely demineralized in ethylene-diamine-tetraacetic acid (EDTA) 17% solution for 21 days and then ultrasonicated in distilled water for 5 min; complete dentin demineralization was checked by total reflectance Fourier-transform infrared spectroscopy (ATR-FTIR; Spectrum 2000, Pelkin Elmer, Shelton, USA).
The completely demineralized dentin sticks were immersed in each primer solution [P-CTR (number of specimens, no sp. = 10); P-PAA (no sp. = 5); P-TMP (no sp. = 5); P-PAA/TMP (no sp. = 5)] and maintained under continuous agitation for 30 s. The specimens were then removed from the primer, air-dried for 10 s to remove the excess of primer and immediately immersed in the R-CTR or R-IR [(P-CTR + R-CTR); (P-CTR + R-IR); (P-PAA + R-IR) (P-TMP + R-IR) (P-PAA/TMP + R-IR)] and maintained under continuous agitation for 3 h in the dark. Finally, the specimens were removed from the resin adhesives air-dried for 10 s to remove the excess of resin and light cured for 20 s each side of the stick using a light-emitting diode source (Bluephase G2, Ivoclar-Vivadent Inc., Schaan, Liechtenstein) with an irradiance value of 1200 mW/cm 2 at a distance of 1 mm.
All the individual specimens were stored a hermetic vials containing 1.5 mL of simulated body fluid (SBF: 205.2 mM NaCl, 6.3 mM NaHCO 3 , 4.5 mM KCl, 1.5 mM KHPO 4 ·3H 2 O, 2.25 mM MgCl 2 ·6H 2 O, 3.75 mM CaCl 2 and 0.75 mM Na 2 SO 4 ) pH = 7.3 at 37 °C for 6 months. The SBF was changed weekly. The remineralization process was evaluated from each side of the sticks after 24 h, 7 days, 2 and 6 months using ATR-FTIR with a spectral resolution was 4 cm −1 and 32 scans for each analysis to identify the appearance of phosphate peaks.
2.3
Transmission electron microscopy (TEM) analysis
After 6 months of SBF immersion, the dentin specimens used for the ATR-FTIR survey were processed for transmission electron microscopy analysis (TEM) . Specimens were fixed in Karnovsky’s solution, rinsed in cacodylate buffer and post-fixed with 1% osmium tetroxide solution. Each stick was rinsed with sodium cacodylate buffer. The sticks were dehydrated in ascending ethanol solutions (30–100%), immersed in propylene oxide and embedded into epoxy resin (Buehler, Lake Bluff, USA). Ninety nanometer thick sections were prepared using an ultra-microtome and examined without further staining using transmission electron microscope (JEM-1400, JEOL, Tokyo, Japan) at 110 kV.
2.4
Dentin bonding procedures
Fifty extracted non-carious human molars were used to prepare flat dentin surfaces perpendicular to the longitudinal axis of each tooth using a slow-speed diamond saw (Isomet, USA) under water-cooling. The occlusal dentin surface was polished with a 600-grit silicon carbide paper under continuous water irrigation for 60 s to produce a standardized smear layer. The specimens (n = 10) were divided into five groups as previously described: 1) P-CTR + R-CTR; 2) P-CTR + R-IR; 3) P-PAA + R-IR; 4) P-TMP + R-IR; 5) P-PAA/TMP + R-IR
On each dentin specimen, the self-etching primers were applied for 10s, gently air-dried for 3 s and the adhesive resin applied vigorously for 20 s with final light-curing for 20 s using a LED unit (Bluephase G2, Ivoclar-Vivadent Inc., Schaan, Liechtenstein), followed by incremental placement of two 2 mm-thick horizontal layers of a resin composite (Filtek Z350 XT, 3M-ESPE, St. Paul, USA), light-cured for 40 s each. After this procedure, half of the specimens (N = 5/group) was tested after 24 h in de-ionized water, whilst the other half was submitted to aging under a simulated pulpal pressure of 20 cm H 2 O for 6 months as described by Feitosa et al., .
2.5
Microtensile bond strength (μTBS)
Resin-bonded specimens were sectioned in resin-dentin sticks (0.9 mm × 0.9 mm) for microtensile bond strength testing; the sticks from the most peripheral area presenting residual enamel were excluded from the test. The sticks were attached to a jig with a cyanoacrylate cement (Super Bonder gel, Loctite, Henkel Corp., Rocky Hill, USA) and tested to tensile failure in an universal testing machine (EZ-test; Shimadzu, Kyoto, Japan) with a 50-N load cell (cross-head speed: 1.0 mm/min). The exact cross-sectional area of each tested stick was measured with a digital caliper after fracture. The μTBS results were calculated and expressed in MPa. The μTBS values obtained from the sticks of the same resin-bonded tooth were averaged and the mean bond strength of each individual tooth was used as one unit for statistical analysis. Five resin-bonded teeth (n = 5) were evaluated for each material sub-group. The μTBS data were statistically analyzed using two-way ANOVA (the independent variables were the bonding agents and the aging period) and Tukey’s multiple comparisons test at α = 5%.
Subsequent to the μTBS testing, the mode of failure of each fractured stick was determined using a stereomicroscope (Olympus SZ 40-50; Tokyo, Japan) at ×100 magnification. The fractures were classified as adhesive, mixed, cohesive in composite or cohesive in dentin.
2.6
Nanoleakage analysis
Two resin-dentin sticks were selected from each specimen of the tested groups and subgroups during the cutting procedure. These sticks were immersed in 50 wt% ammoniacal silver nitrate (AgNO 3 (aq)) solution in total darkness for 24 h . Subsequently, the specimens were rinsed with distilled water to remove the excess of silver nitrate and immersed in a photo-developing solution for 8 h under light in order to reduce silver ions into metallic silver grains. The silver-impregnated sticks were embedded in epoxy resin and polished using 600-, 1200-, 2000-grit SiC papers and diamond pastes (Buehler, Lake Bluff, IL, USA) with 6, 3, 1, and 0.25 μm particle sizes, with an ultrasonic cleaning bath of 20 min after each abrasive/polishing step. Specimens were finally air-dried, dehydrated overnight in silica gel, coated with carbon and analyzed using SEM (JSM-5600LV; JEOL, Tokyo, Japan) and observed in the backscattered electron mode at 15 kV.
2
Materials and methods
2.1
Experimental adhesives
An experimental resin-based solution was prepared and used as a control mildly acidic primer (P-CTR) by blending 20 wt% glycerol-dimethacrylate-phosphate (GDMA-P), 10 wt% hydroxyethyl-methacrylate (HEMA), 15 wt% urethane-dimethacrylate (UDMA), 5 wt% triethyleneglycol-dimethacrylate (TEGDMA), 20 wt% de-ionized water and 30 wt% absolute ethanol. Using this control primer, three additional experimental primers were formulated by adding the biomimetic analogs PAA and/or TMP: i) 10 wt% polyacrylic acid (P-PAA, Mw 1800); ii) 10 wt% sodium trimetaphosphate (P-TMP); iii) 5 wt% PAA + 5 wt% TMP (P-PAA/TMP) . The pHs of the primers were all adjusted to 2.1 using NaOH.
A further experimental co-monomer blend was prepared and used as a control adhesive (R-CTR) resin by mixing 30 wt% UDMA, 25 wt% TEGDMA, 20 wt% GDMA-P, 17 wt% ethoxylated bisphenol-A-diglycidyl-dimethacrylate (Bis-EMA), 5 wt% bisphenol-A-diglycidyl-dimethacrylate (Bis-GMA), 1 wt% ethyl 4-dimethylamine benzoate, 0.5 wt% canphoroquinone and 1.5 wt% diphenyliodonium hexafluorophosphate. The control R-CTR was then used to formulate the experimental ion releasing adhesive (R-IR). This latter was created by adding 20 wt% Ca/P micro-filler (10–50 μm particle size) constituted of a mixture of monocalcium phosphate monohydrate (MCPM) (45 mol%), beta-tricalcium phosphate (β-TCP) (45 mol%) and Ca(OH) 2 (10 mol%). All resin blends created in this study were stirred for 1 h under continuous sonication in order to obtain homogenous solutions.
2.2
FTIR-ATR analysis
Six sound human third molars were obtained under a protocol (038/2014) approved by the institutional Research Ethics Committee (REC) for medical investigations. The teeth were stored at 4 °C in 0.5% Chloramine-T up to 30 days before use. The enamel was removed to expose mid-coronal dentin and twenty-five dentin sticks (7 mm × 2 mm × 1 mm) were obtained from each tooth using a hard tissue microtome (Isomet, Buehler Ltd., Lake Bluff, USA) equipped with a slow-speed water-cooled diamond wafering saw. These sticks were completely demineralized in ethylene-diamine-tetraacetic acid (EDTA) 17% solution for 21 days and then ultrasonicated in distilled water for 5 min; complete dentin demineralization was checked by total reflectance Fourier-transform infrared spectroscopy (ATR-FTIR; Spectrum 2000, Pelkin Elmer, Shelton, USA).
The completely demineralized dentin sticks were immersed in each primer solution [P-CTR (number of specimens, no sp. = 10); P-PAA (no sp. = 5); P-TMP (no sp. = 5); P-PAA/TMP (no sp. = 5)] and maintained under continuous agitation for 30 s. The specimens were then removed from the primer, air-dried for 10 s to remove the excess of primer and immediately immersed in the R-CTR or R-IR [(P-CTR + R-CTR); (P-CTR + R-IR); (P-PAA + R-IR) (P-TMP + R-IR) (P-PAA/TMP + R-IR)] and maintained under continuous agitation for 3 h in the dark. Finally, the specimens were removed from the resin adhesives air-dried for 10 s to remove the excess of resin and light cured for 20 s each side of the stick using a light-emitting diode source (Bluephase G2, Ivoclar-Vivadent Inc., Schaan, Liechtenstein) with an irradiance value of 1200 mW/cm 2 at a distance of 1 mm.
All the individual specimens were stored a hermetic vials containing 1.5 mL of simulated body fluid (SBF: 205.2 mM NaCl, 6.3 mM NaHCO 3 , 4.5 mM KCl, 1.5 mM KHPO 4 ·3H 2 O, 2.25 mM MgCl 2 ·6H 2 O, 3.75 mM CaCl 2 and 0.75 mM Na 2 SO 4 ) pH = 7.3 at 37 °C for 6 months. The SBF was changed weekly. The remineralization process was evaluated from each side of the sticks after 24 h, 7 days, 2 and 6 months using ATR-FTIR with a spectral resolution was 4 cm −1 and 32 scans for each analysis to identify the appearance of phosphate peaks.
2.3
Transmission electron microscopy (TEM) analysis
After 6 months of SBF immersion, the dentin specimens used for the ATR-FTIR survey were processed for transmission electron microscopy analysis (TEM) . Specimens were fixed in Karnovsky’s solution, rinsed in cacodylate buffer and post-fixed with 1% osmium tetroxide solution. Each stick was rinsed with sodium cacodylate buffer. The sticks were dehydrated in ascending ethanol solutions (30–100%), immersed in propylene oxide and embedded into epoxy resin (Buehler, Lake Bluff, USA). Ninety nanometer thick sections were prepared using an ultra-microtome and examined without further staining using transmission electron microscope (JEM-1400, JEOL, Tokyo, Japan) at 110 kV.
2.4
Dentin bonding procedures
Fifty extracted non-carious human molars were used to prepare flat dentin surfaces perpendicular to the longitudinal axis of each tooth using a slow-speed diamond saw (Isomet, USA) under water-cooling. The occlusal dentin surface was polished with a 600-grit silicon carbide paper under continuous water irrigation for 60 s to produce a standardized smear layer. The specimens (n = 10) were divided into five groups as previously described: 1) P-CTR + R-CTR; 2) P-CTR + R-IR; 3) P-PAA + R-IR; 4) P-TMP + R-IR; 5) P-PAA/TMP + R-IR
On each dentin specimen, the self-etching primers were applied for 10s, gently air-dried for 3 s and the adhesive resin applied vigorously for 20 s with final light-curing for 20 s using a LED unit (Bluephase G2, Ivoclar-Vivadent Inc., Schaan, Liechtenstein), followed by incremental placement of two 2 mm-thick horizontal layers of a resin composite (Filtek Z350 XT, 3M-ESPE, St. Paul, USA), light-cured for 40 s each. After this procedure, half of the specimens (N = 5/group) was tested after 24 h in de-ionized water, whilst the other half was submitted to aging under a simulated pulpal pressure of 20 cm H 2 O for 6 months as described by Feitosa et al., .
2.5
Microtensile bond strength (μTBS)
Resin-bonded specimens were sectioned in resin-dentin sticks (0.9 mm × 0.9 mm) for microtensile bond strength testing; the sticks from the most peripheral area presenting residual enamel were excluded from the test. The sticks were attached to a jig with a cyanoacrylate cement (Super Bonder gel, Loctite, Henkel Corp., Rocky Hill, USA) and tested to tensile failure in an universal testing machine (EZ-test; Shimadzu, Kyoto, Japan) with a 50-N load cell (cross-head speed: 1.0 mm/min). The exact cross-sectional area of each tested stick was measured with a digital caliper after fracture. The μTBS results were calculated and expressed in MPa. The μTBS values obtained from the sticks of the same resin-bonded tooth were averaged and the mean bond strength of each individual tooth was used as one unit for statistical analysis. Five resin-bonded teeth (n = 5) were evaluated for each material sub-group. The μTBS data were statistically analyzed using two-way ANOVA (the independent variables were the bonding agents and the aging period) and Tukey’s multiple comparisons test at α = 5%.
Subsequent to the μTBS testing, the mode of failure of each fractured stick was determined using a stereomicroscope (Olympus SZ 40-50; Tokyo, Japan) at ×100 magnification. The fractures were classified as adhesive, mixed, cohesive in composite or cohesive in dentin.
2.6
Nanoleakage analysis
Two resin-dentin sticks were selected from each specimen of the tested groups and subgroups during the cutting procedure. These sticks were immersed in 50 wt% ammoniacal silver nitrate (AgNO 3 (aq)) solution in total darkness for 24 h . Subsequently, the specimens were rinsed with distilled water to remove the excess of silver nitrate and immersed in a photo-developing solution for 8 h under light in order to reduce silver ions into metallic silver grains. The silver-impregnated sticks were embedded in epoxy resin and polished using 600-, 1200-, 2000-grit SiC papers and diamond pastes (Buehler, Lake Bluff, IL, USA) with 6, 3, 1, and 0.25 μm particle sizes, with an ultrasonic cleaning bath of 20 min after each abrasive/polishing step. Specimens were finally air-dried, dehydrated overnight in silica gel, coated with carbon and analyzed using SEM (JSM-5600LV; JEOL, Tokyo, Japan) and observed in the backscattered electron mode at 15 kV.
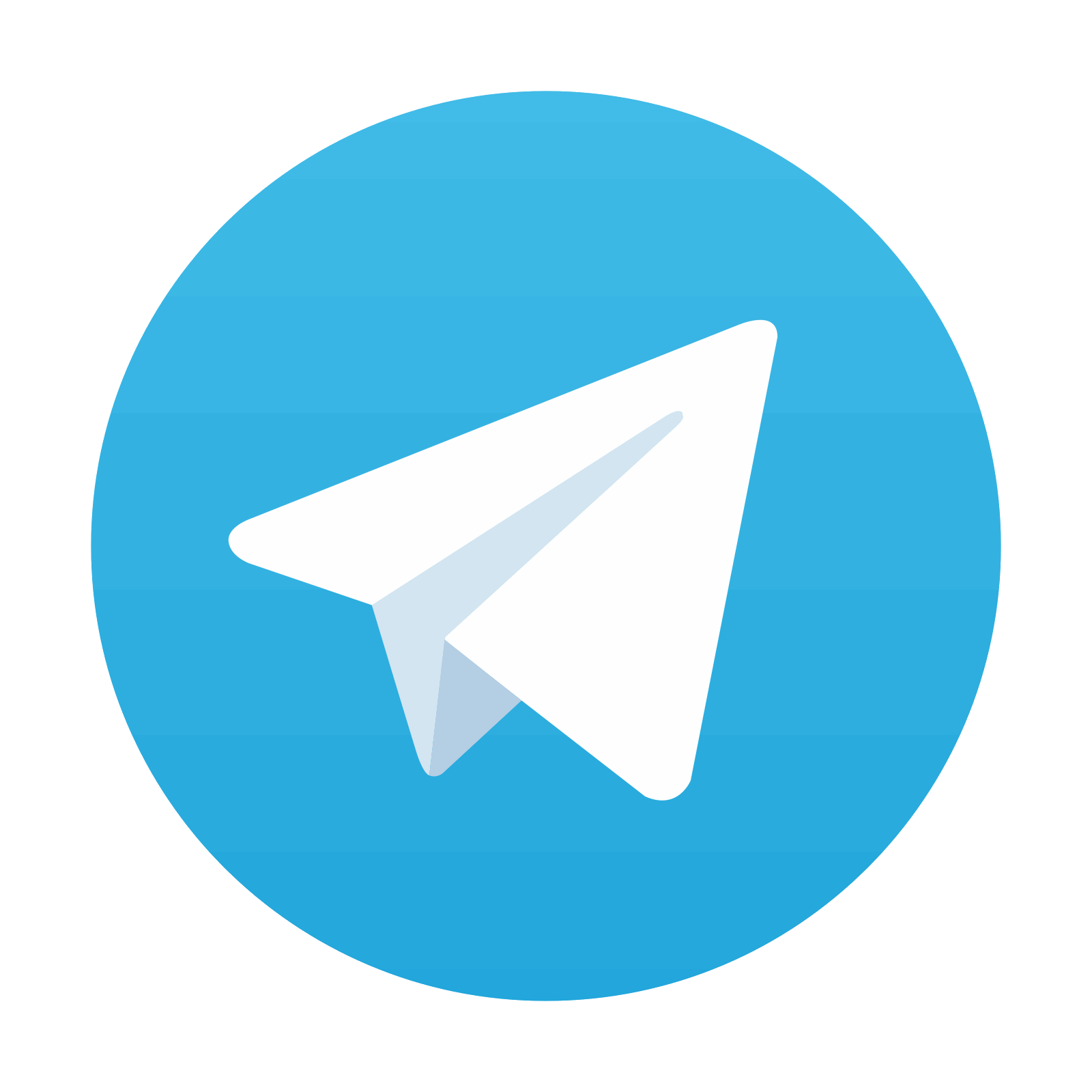
Stay updated, free dental videos. Join our Telegram channel

VIDEdental - Online dental courses
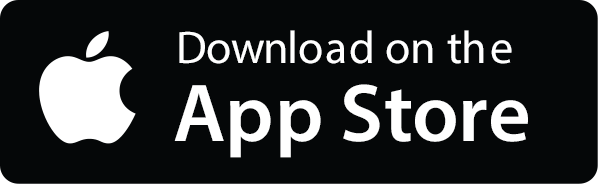
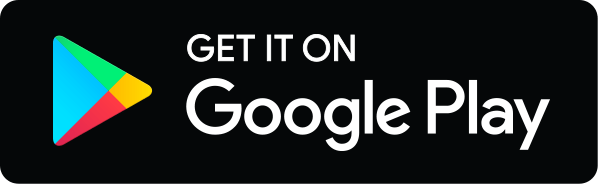