18
Bleeding and Clotting Disorders
Joel J. Napeñas, DDS, FDS RCSEd
Lauren L. Patton, DDS, FDS RCSEd
Oral healthcare professionals are increasingly called upon to provide care to individuals whose bleeding and clotting mechanisms have been altered by acquired or inherited mechanisms. This engagement in care of the coagulopathy patient provides an opportunity for the dentist trained in the recognition of oral and systemic signs of altered hemostasis to assist in the screening and monitoring of the underlying condition.
Inherited coagulopathies, such as the hemophilias and von Willebrand disease (VWD), may be mild to severe in clinical presentation, are present life‐long, are often diagnosed in infancy, and are predictable based on the hereditary pedigree. If it is not diagnosed in childhood, exposure to the first surgical procedure, which is often the removal of third molars, may reveal the underlying inherited bleeding disorder. Acquired coagulation disorders can result from drug actions or side effects of underlying systemic disease or their treatment. Over the past few decades, there have been expanding options for therapeutic anticoagulation for the prevention and management of thromboembolic disease. In addition to heparins and vitamin K antagonists (VKAs; e.g., warfarin), anticoagulants targeting enzymatic activity of thrombin and factor Xa (F Xa), often referred to as novel oral anticoagulants (NOACs) or direct oral anticoagulants (DOACs), have been developed and have become widely used. In addition to anticoagulants, antiplatelet therapy has become ubiquitous for the prevention of stroke or thromboembolic cardiac events (e.g., post coronary artery stenting, post myocardial infarction).
Moreover, systemic disease involving the liver and kidney, inherited or acquired platelet disorders, and myelosuppressive chemotherapy or treatment of hematologic malignancy add to the burden of altered hemostasis. Patients with liver disease may have impaired hemostasis due to thrombocytopenia and/or lack of coagulation factors, whereas renal failure may result in qualitative disorders in platelet function. Patients with hematologic malignancies may have thrombocytopenia as a result of overgrowth of malignant cells in the bone marrow that leaves no room for platelet precursors (megakaryocytes). In addition, cancer patients may have thrombocytopenia as a result of the cytotoxic effects of chemotherapeutic agents to treat their disease.
Invasive dental procedures resulting in bleeding can have serious consequences for the patient with a bleeding disorder, including severe hemorrhage or even death. Safe dental care may require consultation with the patient’s medical provider, institution of systemic management, and dental treatment modifications.
EPIDEMIOLOGY
The World Health Organization’s Global Burden of Disease (WHO‐GBD) study indicates that diseases requiring medication to manage premature clotting are on the rise. By 2017, among all diseases, ischemic heart disease and stroke had risen to number 1 and 3 for leading causes of early death and number of years lived with disability at all ages.1 In many countries, today the use of DOACs has surpassed the use of traditional VKAs.2
VWD is reported as the most common inherited coagulopathy, with a prevalence, including the multiple types and subtypes, of up to 1% of the population.3 Hemophilia A (F VIII deficiency) is the most common congenitally missing coagulation factor deficiency, with a prevalence in the United States of 1:6500 live male births.4 Hemophilia B or Christmas disease (F IX deficiency) occurs about a fifth as frequently as hemophilia A at 1:30,000 live male births.5
Systemic diseases that alter hemostasis are also increasing in prevalence. The American Cancer Society estimates that 1,806,590 individuals will be newly diagnosed with cancer in 2020, many being placed on myelosuppressive chemotherapy that creates thrombocytopenia; of these new cancer cases, 60,530 will be patients with leukemia who in addition may have malignancy‐related thrombocytopenia.6 Using WHO‐GBD data, epidemiologists note that the rise of hepatitis C infection and other causes have increased cases of liver cirrhosis and increased years living with this disease. This places patients at risk for thrombocytopenia and coagulopathies from the inability of the liver to properly form clotting factors; liver cirrhosis accounted for 1.45 million deaths globally in 2013, a 63% increase from 0.89 million in 1990.7 Systemic diseases with alteration of both coagulation and platelet number and/or function increase the complexity of hemostasis management during dental surgical procedures.
MECHANISMS OF HEMOSTASIS
Hemostasis is the process of blood clot formation at the site of vessel injury. Multiple processes occur in a rapid sequence that is localized and regulated. There is a careful balance between thrombin‐stimulated clot formation and plasmin‐induced clot lysis. Abnormal bleeding occurs when there is insufficient clot formation due to decreased thrombin (e.g., from F VIII deficiency) or increased clot lysis. Thrombosis that is nonphysiologic or functional clotting occurs when there is an excessive production of thrombin.
Hemostasis can be divided into four general phases:
- Endothelial injury and platelet plug formation—underway within 10–20 seconds of injury.
- Coagulation cascade and propagation of clotting—an initial hemostatic plug is formed in 1–3 minutes, and fibrin has been generated and added to stabilize the clot by 5–10 minutes.
- Termination of clotting by antithrombotic control mechanisms.
- Fibrinolysis and removal of the clot.
Endothelial Injury and Platelet Plug Formation
When vessel integrity is disrupted, reactants such as serotonin, histamine, prostaglandins (PGs), and other products that are vasoactive cause vasoconstriction of the microvascular bed in the area of the injury and the vessels contract. The immediate reflex vasoconstriction may alone be hemostatic in small vessels. Platelets are activated as well and adhere to the site of injury, forming a platelet plug that reduces or temporarily arrests blood loss.8
Endothelial injury activates endothelial cells to promote the recruitment of platelets, other cells, and procoagulant factors. This occurs in four steps (Figure 18‐1).

Figure 18‐1 Platelet phase. Platelet adhesion occurs immediately (within 1–2 seconds) via the binding of platelet surface receptor glycoprotein (GP) Ib/IX/V complex to von Willebrand factor. Adenosine diphosphate (ADP) is a potent nucleotide that binds to receptors P2Y1 and P2Y12, activating and recruiting other platelets in the area and adding to the size of the plug. Once platelets are stimulated by thrombin, collagen, or ADP the integrin GPIIb/IIIa on platelet surfaces are activated and bind to fibrinogen, in which aggregation occurs (within 10–20 seconds). Platelets then secrete a number of other factors, including thromboxane A2, which promotes vasoconstriction and further platelet aggregation.
Step One: Adhesion
Once the endothelium is injured, subendothelial collagen, laminin, and microfibrils are exposed. Platelet stimuli include adenosine diphosphate (ADP), epinephrine, thrombin, and collagen. Platelet adhesion occurs via the binding of platelet surface receptor GPIb/IX/V complex to von Willebrand factor (VWF) in the subendothelial matrix.9 GPIa/IIa and GPVI are important collagen receptors on the surface of platelets, involved in adhesion and activation, respectively.10 ADP is a potent nucleotide that binds to receptors P2Y1 (which leads to calcium mobilization, platelet shape change, and aggregation) and P2Y12 (responsible for platelet secretion and stable aggregation), in effect activating and recruiting other platelets in the area and adding to the size of the plug. Platelets have two receptors for thrombin, protease‐activated‐receptors 1 and 4 (PAR‐1 and PAR‐4).11 Platelet factor 3 is the intracellular phospholipid that activates F X and subsequently results in the conversion of prothrombin to thrombin.
Step Two: Aggregation
Once platelets are stimulated (e.g., by thrombin, collagen, or ADP), the integrin GPIIb/IIIa on platelet surfaces is activated and becomes a high‐affinity fibrinogen receptor. The GPIIb/IIIa complex also binds to immobilized VWF, resulting in platelet spreading and clot retraction.
Step Three: Activation and Secretion
Platelets secrete a number of substances upon cell stimulation, including:
- ADP and serotonin—activates and recruits additional platelets.
- Fibronectin and thrombospondin—adhesive proteins that stabilize platelet aggregates.
- Fibrinogen.
- Thromboxane A2 (TXA A2)—promotes vasoconstriction and further platelet aggregation.
- Growth factors—mediate tissue repair at the site of vascular injury.
Step Four: Procoagulant Activity
This involves the exposure of procoagulant phospholipids (e.g., phosphatidylserine) and the assembly of the enzyme complexes in the coagulation cascade on the platelet surface.12 This leads to fibrin formation and the generation of an insoluble fibrin clot that strengthens the platelet plug.8
The Coagulation Cascade and Propagation of Clotting
The generation of thrombin and fibrin is the end product of the coagulation phase. This process involves multiple proteins, many of which are synthesized by the liver (fibrinogen; prothrombin; F V, F VII, F IX, F X, F XI, F XII, and F XIII) and are vitamin K dependent (F II, F VII, F IX, and F X); see Table 18‐1. Vitamin K is an essential cofactor for a carboxylase that catalyzes the carboxylation of glutamic acid residues on vitamin K–dependent proteins, thereby making them biologically active.
A sequence of interactions between the various clotting factors occurs following tissue injury. The scheme of reaction is a bioamplification, in which a precursor is altered to an active form, which, in turn, activates the next precursor in the sequence. The coagulation of blood requires the presence of both calcium ions and exposed phospholipid on platelet surfaces. Beginning with an undetectable biochemical reaction, the coagulation mechanism results in a final explosive change of a liquid to a gel.
Classical Coagulation Cascade Model
The traditional model of the cascade (Figure 18‐2) is useful for interpreting in vitro tests of coagulation (e.g., prothrombin time [PT], international normalized ratio [INR]); however, it does not fully represent what occurs in vivo (i.e., in the body). It involves two separate pathways (intrinsic and extrinsic) that converge by activating a third (common) pathway:
Table 18‐1 Coagulation factors.
Coagulation Factor Affected | |||
---|---|---|---|
Factor (Name) | Intrinsic | Extrinsic | t1/2 (h) |
XIII (fibrin‐stabilizing factor) | * | * | 336 |
XII (Hageman factor) | * | 60 | |
XI (plasma thromboplastin antecedent) | * | 60 | |
X (Stuart factor) | * | * | 48 |
IX (Christmas factor) | * | 18–24 | |
VIII (antihemophilic factor) | * | 8–12 | |
VII (proconvertin) | * | 4–6 | |
V (proaccelerin) | * | * | 32 |
IV (calcium) | * | * | — |
III (tissue thromboplastin) | * | — | |
II (prothrombin) | * | * | 72 |
I (fibrinogen) | * | * | 96 |
t1/2, half‐life.
- The intrinsic pathway is initiated by the exposure of blood to a negatively charged surface. F XII is activated by surface contact (e.g., with collagen or subendothelium), and it involves the interaction of F XII and F XI to an active form (F Xia). The next step, the activation of F IX to F IXa, requires a divalent cation such as calcium.13 Once activated, F IXa forms a complex with F VIII, in a reaction that requires the presence of both calcium ions and phospholipid, which, in turn, converts F X to an activated form—F Xa.
- The extrinsic pathway is initiated by the release of tissue factor (TF), also called tissue thromboplastin, and does not require contact activation. TF binds to F VII in the presence of calcium, and this complex is capable of activating F IX and X, linking the intrinsic and extrinsic pathways.
- The common pathway begins through the activation of F X. Once activated, F Xa converts prothrombin to thrombin in a reaction similar to the activation of F X by F IXa. The activation of prothrombin by F Xa requires the presence of calcium ions and phospholipid as well as F V, a plasma protein cofactor.14 Once formed, thrombin converts fibrinogen, a soluble plasma protein, to insoluble fibrin. Fibrin polymerizes to form a gel, stabilizing the platelet plug. Finally, F XIII, which has been converted to an activated form by thrombin,15 produces covalent cross‐links between the fibrin molecules that strengthen the clot and make it more resistant to lysis by plasmin.
In Vivo Coagulation Cascade
The current established in vivo physiologic model of clotting has three overlapping phases: initiation, amplification, and propagation (Figure 18‐3). The activations and reactions of clotting factors occur on cell surfaces (e.g., activated platelets, endothelial cells) and a number of different cells (e.g., monocytes, fibroblasts). Standard laboratory clotting tests, which detect initial fibrin clot formation, primarily measure the initiation and not the propagation phase of clotting.

Figure 18‐2 Classical in vitro coagulation cascade.
- The primary initiation event in clotting is the generation or exposure of TF at the wound site, on TF‐bearing cells, and its interaction with and activation of F VII with the aid of F IXa.16,17 This TF–F VIIa complex activates F X, which gives rise to a small amount of thrombin (minor).
- The amplification phase occurs on the surface of platelets. In addition to activating platelets, the small amount of thrombin also activates F V, F VIII, and F XI, which participate in generating large amounts of thrombin (major).18
- In the propagation phase, procoagulant complexes are assembled on platelet membrane surfaces in the presence of calcium. An extrinsic tenase (X‐ase) complex consists of F Va and TF, and activates F X and F IX. An intrinsic X‐ase complex consists of F IXa and F VIIIa, and activates F X. The F Xa generated from either X‐ases binds with F Va to form the prothrombase on the surface of the platelet, which then generates a large amount of thrombin through the conversion from prothrombin (F II) to thrombin (F IIa). This ultimately leads to the conversion of fibrinogen to fibrin.18 In addition, thrombin activates F XIII (fibrin‐stabilizing factor), which cross‐links the monomeric fibrin to stabilize the clot.
Termination
Coagulation is modulated by several mechanisms: dilution of procoagulants in flowing blood, removal of activated factors through the reticuloendothelial system by the liver, and control of the activated procoagulants and platelets by natural antithrombotic pathways, mostly on vascular endothelial cells.
The inhibitors of the coagulation pathways are:
- Tissue factor pathway—tissue factor pathway inhibitor (TFPI) inhibits factor X activation in two ways: by directly inhibiting F Xa and by inhibiting the extrinsic X‐ase complex by complexing with factor Xa.
- Contact activation pathway—C1 esterase inhibitor (C1‐inh).
The regulation of the terminating phase of coagulation involves:
- Antithrombin (AT)—a circulating plasma protease inhibitor, which neutralizes most of the enzymes (i.e., thrombin, F Xa, F IXa, F XIIa).
- Protein C pathway (Figure 18‐4)—protein C is activated by thrombin after it binds to thrombomodulin during the process of clot formation, to form the protein C anticoagulant complex. Activated protein C, in association with protein S on phospholipid surfaces, inactivates F Va and F VIIIa, effectively inactivating the prothrombinase and intrinsic X‐ase complex, respectively.19

Figure 18‐3 In vivo coagulation cascade. TF complexes with factor VIIa (F VIIa) at the site of tissue injury. Activation of F X produces small amounts of thrombin, which in turn activate platelets and F V and VII (F VIII), which assemble on the surface of platelets to form prothrombinase and tenase complexes, respectively. The tenase complex activates F X, which forms on the prothrombinase complex that converts prothrombin to thrombin, which then converts fibrinogen to fibrin for the stable clot.

Figure 18‐4 Termination of clotting.
Fibrinolysis
Fibrinolysis is considered the major means of disposing of fibrin after its hemostatic function has been fulfilled (Figure 18‐5). This is critical to the process of wound healing, in order to restore vessel patency, and for tissue remodeling. Tissue plasminogen activator (tPA) is released from the endothelial cells and converts plasminogen to plasmin, which degrades fibrinogen and fibrin into fibrin degradation products (FDPs). tPA is a proteolytic enzyme that is nonspecific and also degrades F VIII and F V. Urokinase is also a plasminogen activator that is responsible for extravascular fibrinolysis. Kallikrein, which is an intrinsic activator of plasminogen, is generated when prekallikrein is bound to kininogen, thereby becoming a substrate for F XIIa. Activation of the fibrinolytic system can be turned off by inhibition of plasmin activity by α2‐antiplasmin, or inhibition of plasminogen activators by plasminogen activator inhibitors 1 and 2 (PAI‐1 and PAI‐2).20,21
GENERAL WORKUP OF PATIENTS WITH SUSPECTED BLEEDING OR CLOTTING DISORDER
Patient History and Clinical Features
Patient evaluation starts with a routine medical history, via a thorough questionnaire and interview. As part of the overall query, patients should be asked about any history of previous unusual bleeding episodes after surgery or injury, easy or frequent bruising, or spontaneous bleeding (Figure 18‐6). Specifically, patients should be asked about prolonged bleeding after invasive dental procedures, spontaneous bleeding from the gingivae, and history of nasal bleeding. They should also be asked about a family history of bleeding disorders. For the purpose of history‐taking, a clinically significant bleeding episode has been defined as follows:22

Figure 18‐5 The fibrinolytic system.
- Continues beyond 12 hours.
- Requires patient to call or return to treating dentist, or seek emergency care with a medical provider.
- Results in development of hematoma or ecchymosis in soft tissues.
- Requires blood product support.
A complete list of medications is essential, to include over‐the‐counter products, prescription drugs, and supplements, as they can have implications for hemostasis. A social history that determines alcohol consumption and illicit drug use is also essential.
A number of clinical features can be seen in patients with bleeding disorders:
- Petechiae. Petechiae are small, flat, red, discrete areas of skin bleeding that are typically <2 mm in diameter, nonblanching, and nonpalpable. Although typically occurring in lower extremities, sacral area, and areas of skin fragility, they can also occur in the oral cavity, especially with severe thrombocytopenia.
- Bruise/ecchymosis. A bruise (also called ecchymosis) is caused by subcutaneous/submucosal accumulation of extravasated blood (Figure 18‐7). The color can range from purplish blue to reddish brown to greenish yellow, indicative of breakdown of hemoglobin to biliverdin and bilirubin.
Figure 18‐6 Spontaneous gingival bleeding between the upper left lateral incisor and canine and labial petechiae in a 38‐year‐old white male with idiopathic thrombocytopenic purpura.
- Hematoma. A hematoma is a collection of blood in the extravascular space. A subcutaneous hematoma may raise the skin profile. Hematomas in deep tissues (e.g., muscle, retroperitoneal) may be suspected due to pain, a drop in hemoglobin level, or a fluid collection on imaging studies. Hematomas and hemarthroses are typical of coagulation factor deficiencies.
Features to look for in the general examination of a patient include multiple ecchymoses of the skin, bleeding wounds, hematomas, and swollen joints. Clinical manifestations of bleeding disorders can involve various systems, depending on the extent and type of disorder (Table 18‐2). Individuals with mild disease may present with no clinical signs, whereas individuals with severe coagulopathies may have definite stigmata. When skin and mucosa are involved, individuals may present with petechiae, ecchymoses, spider angiomas, hematomas, or jaundice. Deep dissecting hematomas and hemarthroses of major joints may affect severe hemophiliacs and result in disability or death. Disorders of platelet quantity may result in hepatosplenomegaly, spontaneous gingival bleeding, and risk of hemorrhagic stroke. Specific clinical manifestations will be discussed in detail for the various conditions. Intraorally, petechiae, ecchymoses, hematomas, or excessive gingival bleeding should be of concern to the dentist.

Figure 18‐7 A 68‐year‐old woman with acute myelogenous leukemia and a platelet count of 9000/mm3. Platelet transfusions and e‐aminocaproic acid oral rinses were used to control bleeding. (A) Buccal mucosa and palatal ecchymoses. (B) Extrinsic stains on teeth from erythrocyte degradation following continual gingival bleeding.
Table 18‐2 Clinical features of bleeding disorders.
Feature | Vascular or Platelet Disorders | Coagulation Disorders |
---|---|---|
Bleeding from superficial cuts and scratches | Persistent, often perfuse | Minimal |
Delayed bleeding | Rare | Common |
Spontaneous gingival bleeding | Characteristic | Rare |
Petechiae | Characteristic | Rare |
Ecchymoses | Characteristic, usually small and multiple | Characteristic, usually large and solitary |
Epistaxis | Common | Common |
Deep dissecting hematomas | Rare | Characteristic |
Hematoma and hemarthrosis | Rare | Characteristic |
Laboratory Studies
When a bleeding disorder is suspected, laboratory studies should be initiated prior to an invasive procedure. There are a variety of laboratory tests that help identify a deficiency of required elements or dysfunction of the phases of coagulation (Tables 18‐3 and 18‐4). To evaluate primary hemostasis involving platelets, there are platelet count and platelet function tests (e.g., bleeding time [BT]) and other assays (e.g., platelet function analyzer [PFA‐100]). Tests to evaluate the status of coagulation function include PT/INR, activated partial thromboplastin time (aPTT), thrombin time (TT), FDPs, specific coagulation factor assays (e.g., F VII, F VIII, F IX, fibrinogen), and coagulation factor inhibitor screening tests (blocking antibodies).
Table 18‐3 Laboratory tests for assessing hemostasis.
Test | Normal Range |
---|---|
Platelet count | 150,000–450,000/mm3 |
Bleeding time | <7 min (by simplate); 1–6 min (modified Ivy’s test) |
PFA‐100 closure time | CT‐EPI < 164 s; CT‐ADP < 116 s |
PT/INR | Control ± 1 s (e.g., PT: 11–13 s / INR 1.0) |
Activated partial thromboplastin time (aPTT) | Comparable to control (e.g., 15–35 s) |
Thrombin time (TT) | Control ± 3 s (e.g., 9–13 s) |
Fibrin degradation products (FDPs) | <10 μg/dL |
Fibrinogen assay | 200–400 mg/dL |
von Willebrand antigen | 60–150% vWF activity |
Coagulation factor assays (e.g., F VIII assay) | 60–100% F VIII activity |
Coagulation factor inhibitor assays (e.g., Bethesda inhibitor assay for F VIII) | 0.0 Bethesda inhibitor units |
CT‐ADP, closure time with collagen and adenosine diphosphate membrane; CT‐EPI, closure time with collagen and epinephrine membrane; F, factor; INR, international normalized ratio; PT, prothrombin time; vWF, von Willebrand factor
Initial tests for patients with a suspected bleeding disorder include a complete blood count (CBC) with platelet count, PT/INR, and aPTT. Subsequent testing can be performed based on initial clinical and laboratory findings or the suspected underlying condition.
Platelet Count
Platelet counts are obtained as part of a standard CBC. Normal values are 150,000–450,000/mm3. Spontaneous hemorrhage is usually not observed with platelet counts above 10,000–20,000/mm3. Many hospitals have established a critical value of 10,000/mm3 platelets, below which platelets are transfused to prevent serious bleeding sequelae, such as hemorrhagic stroke; however, such thresholds are dependent upon the anticipated risk of bleeding based on the procedure. Surgical or traumatic hemorrhage may be more likely with severely reduced platelet counts.
Table 18‐4 Laboratory test results for select disorders.
Screening Laboratory Tests | ||||
---|---|---|---|---|
Bleeding Disorder | Platelet Count | PT/INR | aPTT | BT |
Thrombocytopenia | ↓ | N | N | ↑ |
Leukemia Liver disease |
||||
F VIII, IX, XI deficiency | N | N | ↑ | N |
Heparin anticoagulation Thrombin inhibitor anticoagulation |
||||
F II, V, X deficiency | N | ↑ | ↑ | N |
Vitamin K deficiency | ||||
Intestinal malabsorption | ||||
F VII deficiency | N | ↑ | N | N |
Coumarin anticoagulation F Xa inhibitor anticoagulation |
||||
Liver disease | ||||
von Willebrand disease | N, ↓ | N | N, ↑ | ↑ |
DIC | ↓ | ↑ | ↑ | ↑ |
Severe liver disease | ||||
F XIII deficiency | N | N | N | N |
Vascular wall defect | N | N | N | ↑ |
aPTT, activated partial thromboplastin time; BT, bleeding time; DIC, disseminated intravascular coagulation; INR, international normalized ratio; N, normal; PT, prothrombin time; ↑ = increased; ↓ = decreased.
Tests of Platelet Function
The Platelet Function Analyzer (PFA‐100) measures the time it takes for blood flow to stop under shear stress in a capillary tube that has a membrane lined with collagen and epinephrine, or collagen and ADP, and then exposed to shear stress, which is deemed the closure time (CT).23 This test was found to be more sensitive to aspirin‐induced platelet dysfunction and VWD, and was more rapid and less expensive than the BT.24 However, because it is a global test system and also sensitive to low hematocrit, low platelet counts, and platelet dysfunction (both congenital and acquired), it is neither specific for, nor predictive of, any particular disorder (inclusive of VWD), therefore it is recommended that a CBC be performed along with this test.25 It is also insensitive to other therapeutic antiplatelet agents such as clopidogrel, ticlopidine, and cyclooxygenase‐2 (COX‐2) inhibitors.26,27 Though one clinical trial reported the use of the PFA‐100 CT as a screening tool for oral surgery patients on antiplatelet therapy,28 the role of the PFA‐100 CT in routine therapeutic monitoring of platelet function for dental procedures remains to be established.
For assessing the response to clopidogrel, a US Food and Drug Administration (FDA)‐approved test for assessing P2Y‐12‐mediated platelet function is called the “Plavix Response” or the “VerifyNow P2Y‐12 assay.” This uses anticoagulated whole blood for turbidometric detection of platelet aggregation in which activated platelets bind to nearby platelets via fibrinogen‐coated beads in the assay.
Bleeding Time
The BT test was previously used as a screening test for platelet function. It is thought to identify qualitative or functional platelet defects. The modified Ivy test involves a standardized incision on a forearm distal to a blood pressure cuff inflated to 30 mm Hg. The wound is blotted with filter paper and monitored until absorption of blood on the paper ceases. Normal range is between 1 and 6 minutes and is considered significantly prolonged when greater than 15 minutes. However, because of the technique’s sensitivity, it lacks specificity. The skin BT test has been shown to be a poor indicator of clinically significant bleeding at other sites, including oral postoperative bleeding after oral surgical procedures, and its use as a predictive screening test has been discouraged.29,30
Prothrombin Time and International Normalized Ratio
The PT and INR tests measure the time it takes for blood to clot by forming thrombin. They evaluate the extrinsic and common coagulation pathways, screening for the presence or absence of fibrinogen (F I), prothrombin (F II), and F V, F VII, and F X. The normal range of PT is approximately 11–13 seconds. Because of individual laboratory reagent variability and the desire to be able to reliably compare the PT from one laboratory with that from another, the PT test is commonly reported with the INR.31,32 The INR, introduced by the World Health Organization in 1983, is the ratio of PT that adjusts for the sensitivity of the thromboplastin reagents, such that a normal coagulation profile is reported as an INR of 1.0, and higher values indicate abnormal coagulation.33 Its most common use is to measure the effects of VKAs and reduction of the vitamin K–dependent F II, F VII, F IX, and F X. It is not effective for hemophilias A and B, since it does not measure F VIII or F IX. Although most patients on VKAs are monitored by monthly venous blood draws and laboratory analysis, the CoaguChek system allows Clinical Laboratory Improvements Amendments (CLIA)‐waived point‐of‐care PT/INR testing of fingerstick blood in physicians’ and dentists’ offices.34
Activated Partial Thromboplastin Time
Also among the standard initial tests that measure clotting via thrombin formation, aPTT is used to evaluate the intrinsic coagulation pathway, and screen for deficiencies in F VIII, F IX, F XI, and F XII, in addition to prekallikrein and high molecular weight kiningen. It is performed by calcifying plasma in the presence of a thromboplastic material (i.e., phospholipid tablet) and a contact activator that is a negatively charged substance (e.g., kaolin) in the absence of TF. It is considered normal if the control aPTT and the test aPTT are within 10 seconds of each other. Control aPTT times are usually 15–35 seconds. Normal ranges depend on the manufacturer’s limits, as each supplier varies slightly. As a screening test, the aPTT is prolonged only when the factor levels in the intrinsic and common pathways are less than approximately 30%. It is altered in hemophilias A and B and with the use of the anticoagulant heparin, which may result in clinical bleeding problems. However, elevated aPTT due to deficiencies in F X II, prekallikrein, and high molecular weight kiningen do not correlate with clinical bleeding.
Thrombin Time
TT measures the final step in the clotting cascade, the cleavage of fibrinogen to fibrin. It specifically tests the ability to form the initial fibrin clot from fibrinogen, by adding thrombin to plasma. Its normal range is 9–13 seconds, with values in excess of 16–18 seconds considered to be prolonged. It is used to measure the activity of thrombin inhibitor anticoagulants (e.g., heparin, dabigatran), FDPs, or other paraproteins that inhibit the conversion of fibrinogen to fibrin. Fibrinogen can also be specifically assayed and should be present at a level of 200–400 mg/dL.
Fibrin Degradation Products
Disorders of fibrinolysis (clot breakdown) often show delayed bleeding. FDPs are measured using a specific latex agglutination system to evaluate the presence of the D‐dimer of fibrinogen and/or fibrin above normal levels. Such presence indicates that intravascular lysis has taken place or is occurring. This state can result from primary fibrinolytic disorders or disseminated intravascular coagulation (DIC).
Specific Clotting Factor Assays
Specific activity levels of factors are measured if one or more of the screening tests are abnormal, in order to further identify factor deficiencies and their level of severity. Normal factor activity is usually in the 60%–150% range. Coagulation factor inhibitor tests, often referred to as the Bethesda titer assay, measured in units, are essential when sufficient factor concentrate to correct the factor deficiency under normal conditions fails to control bleeding. To identify the specific type of VWD (types I–III and platelet type), additional studies, such as ristocetin cofactor, ristocetin‐induced platelet aggregation studies, and monomer studies, are helpful. These are discussed in more detail later in this chapter.
Tests of Capillary Fragility
The tourniquet test for capillary fragility is useful for identifying platelet disorders and is the only test to demonstrate abnormal results in vessel wall disorders. A moderate degree of stasis is produced by a tourniquet or by inflating a blood pressure cuff around the arm in the usual manner to a pressure halfway between systolic and diastolic levels, and maintained for 5–10 minutes. At 2 minutes following tourniquet or cuff deflation and removal, a 2.5 cm diameter region (size of a quarter) of skin on the volar surface of the arm at 4 cm distal to the antecubital fossa is observed for petechial hemorrhages. This petechial display is called the Rumpel–Leede phenomenon; normally the petechiae count does not exceed 5 in men and 10 in women and children, and is considered abnormal at more than 10–20.
BLOOD VESSEL DISORDERS
Vessel wall disorders can be due to structural malformation of vessels and inherited or acquired disorders of connective tissue. They can result in hemorrhagic features, though bleeding is usually mild and confined to the skin, mucosa, and gingiva. Vascular purpura can result from damage to capillary endothelium, from abnormalities in the vascular subendothelial matrix or extravascular connective tissue bed, or from abnormal vessel formation.
Scurvy
Scurvy results from a dietary deficiency of water‐soluble vitamin C, when dietary vitamin C falls below 10 mg/d. Vitamin C is necessary for the synthesis of hydroxyproline, an essential constituent of collagen. The US Recommended Daily Allowance of vitamin C is 90 mg daily for men and 75 mg daily for women. Scurvy is found primarily in regions of urban poverty, among either infants on nonsupplemented processed milk formulas, the elderly who cook for themselves, adults with alcohol or drug dependencies, children with special needs and underlying medical conditions such as sickle cell anemia or thalassemia with iron overload from multiple transfusions, neurologic conditions, and a history of chemotherapy or aversion to vitamin C–rich foods, or the intellectually disabled.35,36,37,38 Many of the hemorrhagic features of scurvy result from defects in collagen synthesis. Among the first clinical signs are petechial hemorrhages, corkscrew hair at the hair follicles, and purpura on the back of the lower extremities that coalesce to form ecchymoses. Hemorrhage can occur in the muscles, joints, nail beds, and gingival tissues. Severe vitamin C deficiency is considered a systemic risk factor for periodontitis, as it compromises antioxidant micronutrient defenses to oxidative stress and adversely influences collagen synthesis, resulting in weakened capillary blood vessel walls and enhanced gingival bleeding.39 Gingival involvement may include swelling, friability, bleeding, secondary infection, and loosening of teeth. Implementation of a diet rich in vitamin C and administration of 1 g/d of vitamin C supplements provides rapid resolution.
Cushing’s Syndrome
Cushing’s syndrome, resulting from excessive exogenous or endogenous corticosteroid intake or production, leads to general protein wasting and atrophy of supporting connective tissue around blood vessels. Patients may show skin bleeding or easy bruising. Aging causes similar perivascular connective tissue atrophy and lack of skin mobility. Tears in small blood vessels can result in irregularly shaped purpuric areas on arms and hands, called purpura senilis. Other metabolic or inflammatory disorders resulting in purpura include Schönlein–Henoch or anaphylactoid purpura, hyperglobulinemic purpura, Waldenström’s macroglobulinemia, multiple myeloma, amyloidosis, and cryoglobulinemia.
Ehlers–Danlos Syndrome
Ehlers–Danlos syndrome (EDS) is an autosomal dominant or autosomal recessive inherited disorder of connective tissue matrix, generally resulting in fragile skin blood vessels and easy bruising. It is characterized by hyperelasticity of the skin and hypermobile joints. Thirteen types have been identified with unique biochemical defects and varying clinical features, and were sorted using a pathogenetic scheme into the 2017 International Classification:40,41
- Classic EDS (former type I) presents with soft, velvety, hyperextensible skin; easy bruising and scarring; hypermobile joints; varicose veins; and prematurity.42
- Periodontal EDS (former Type VIII), which was mapped to chromosome 12q13,43 has skin findings similar to those in classic EDS, with easy bruising following minor trauma due mainly to the resulting fragility of the oral mucosa and blood vessels, and is characterized by early‐onset periodontal disease with severe loss of alveolar bone and permanent dentition.44
- Children with arthrochalasia EDS (former type VII) may present with microdontia and collagen‐related dentinal structural defects in primary teeth, in addition to bleeding after tooth brushing.45
- Patients with vascular EDS (former type IV) may have a characteristic facial appearance, gingival recession, and gingival fragility.
- Those with spondylodysplastic EDS (former progeroid type) may have characteristic facial features and tooth discoloration or dysplastic teeth.
Other oral findings include fragility of the oral mucosa, gingiva, and teeth, as well as hypermobility of the temporomandibular joint (TMJ) and stunted teeth and pulp stones on dental radiographs.46 Oral health may be severely compromised as a result of specific alterations of collagen in orofacial structures. A number of tissue responses (mucosa, periodontium, pulp) and precautions (e.g., prevention of TMJ dislocation) should be considered when planning dental treatment.47
Hereditary Hemorrhagic Telangiectasia
Hereditary hemorrhagic telangiectasia (HHT), also called Rendu–Osler–Weber syndrome, is a group of autosomal dominant disorders with abnormal telangiectatic capillaries, frequent episodes of nasal and gastrointestinal (GI) bleeding, and associated brain and pulmonary lesions.48,49,50 The Scientific Advisory Board of the HHT Foundation International’s consensus clinical diagnostic criteria, known as the Curaçao criteria, requires the presence of at least three of the four following criteria:51
- Spontaneous and recurrent epistaxis.
- Multiple telangiectasia at characteristic sites (lips, oral cavity, nose, fingers).
- Visceral arteriovenous malformations.
- First‐degree relative with HHT history.
Perioral and intraoral angiomatous nodules or telangiectases are common with progressive disease, involving areas of the lips, tongue, and palate that may bleed upon manipulation during dental procedures.52 Small nonpulsating visceral arteriovenous malformations may blanch in response to applied pressure, unlike petechiae or ecchymoses. Mucocutaneous lesions may bleed profusely with minor trauma, or on occasion spontaneously.53 Persistently bleeding oral lesions may be treated with cryotherapy, laser ablation, electrocoagulation, or antifibrinolytics.54 Submucosal and intravenous (IV) bevacizumab, a humanized recombinant monoclonal antibody to the angiogenic vascular endothelial growth factor that is elevated in HHT, has recently shown promise in treatment of epistaxis in HHT.55 It has also been suggested that antibiotic prophylaxis should be considered before dental care to reduce the occurrence of cerebral abscesses for patients with HHT and concomitant pulmonary arteriovenous malformation.56
PLATELET DISORDERS
Congenital Platelet Disorders
Congenital abnormalities of platelet function or production are rare and the causes are quite diverse. Causes range from defects in receptors critical to platelet adhesion and aggregation, to defects in signaling molecules, or in transcription factors important for the production of functional platelets. Conditions may present with large, small, or normal‐sized platelets and be associated with other diseases or clinical features (Table 18‐5).57
Bernard–Soulier Syndrome
Bernard–Soulier syndrome, a hemorrhagioparous thrombocytic dystrophy, is a rare autosomal recessive disorder that results from an identified absence of the platelet membrane GPIb‐IX‐V complex from the platelet membranes, rendering the platelets unable to interact with VWF.58,59 Features include epistaxis as the most common clinical sign, giant platelets, decreased platelet count, and increased bleeding time. The main treatment modality for Bernard–Soulier syndrome is supportive measures combined with specific treatment of bleeding episodes, typically with human leukocyte antigen (HLA)‐matched platelet transfusions.58
Glanzmann’s Thrombasthenia
Glanzmann’s thrombasthenia is a qualitative disorder characterized by mucocutaneous bleeding due to mutations in the ITGA2B and ITGB3 genes encoding the integrin αIIbβ3.60 As a result, platelet membrane GPIIb/IIIa cannot bind to fibrinogen and cannot aggregate with other platelets, although platelet count is not altered. Clinical signs include bruising, epistaxis, gingival hemorrhage, and menorrhagia. Rarely, Glanzmann’s thrombasthenia may be acquired in association with pregnancy, autoimmune conditions (e.g., systemic lupus erythematosus [SLE], immune thrombocytopenia), and therapeutic GPIIb/III antagonists (e.g., abciximab, eptifibatide). Treatment of oral surgical bleeding involves platelet transfusion, antifibrinolytics, recombinant factor (rF) VIIa, and local hemostatic agents, alone or in combination.61 Treatment of bleeding episodes in the patient with Glanzmann’s thrombasthenia is usually not warranted unless hemorrhage is life‐threatening.
Wiskott–Aldrich Syndrome
Wiskott–Aldrich syndrome is a rare X‐linked recessive disease, with ill‐defined pathophysiology, characterized by mild to severe presentation of cutaneous eczema (usually beginning on the face), bleeding from thrombocytopenic purpura, and propensity to infection due to an immunologic defect.62 It is a quantitative and qualitative platelet disorder. Oral manifestations include gingival bleeding and palatal petechiae. Thrombocytopenia of Wiskott–Aldrich syndrome may be managed with platelet transfusions, antifibrinolytic drugs, splenectomy (with risk of increased infections), or allogeneic hematopoietic stem cell transplantation, with hope of gene therapeutic approaches available in the future.62
Table 18‐5 Classification of platelet disorders. Source: Based on Sharma R, Perez Botero J, Jobe SM. Congenital disorders of platelet function and number. Pediatr Clin North Am. 2018;65(3):561–578.
Thrombocytopenic—quantitative platelet deficiency | Nonthrombocytopenic—qualitative or functional platelet defect | ||
---|---|---|---|
Decreased platelet activity | Increased platelet activity | ||
Congenital | |||
May–Hegglin anomaly | X | ||
Wiskott–Aldrich syndrome | X | X | |
Gray platelet syndrome | X | ||
Neonatal alloimmune thrombocytopenia | X | ||
Glanzmann’s thrombasthenia | X | ||
Platelet‐type von Willebrand disease | X | ||
Bernard–Soulier syndrome | X | ||
Sticky platelet syndrome | X | ||
Acquired | |||
Primary immune thrombocytopenia | X | ||
Thrombotic thrombocytopenic purpura | X | ||
Leukemia | X | ||
Aplastic anemia | X | ||
Myelodysplasia | X | ||
Systemic lupus erythematosus | X | ||
Disseminated intravascular coagulation | X | ||
Drug induced—cancer chemotherapy | X | X | |
Drug induced— therapeutic antiplatelet agents: ASA, NSAIDs, clopidogrel, ticlopidine, dipyridamole, cilostazol, abciximab, epitifibatide, tirofiban Penicillin, cephalosporins | X | ||
Cardiopulmonary bypass | X | X | |
Renal disease | X | ||
Alcohol dependency | X | X | |
Liver disease | X | X | |
Diabetes | X | ||
Dysproteinemia (i.e., myeloma, myeloproliferative disorders, macroglobulinemia) | X | ||
Acquired platelet‐type von Willebrand disease | X |
NSAIDs, nonsteroidal anti‐inflammatory drugs.
May–Hegglin Anomaly
May–Hegglin anomaly, one of the now four autosomal dominant MYH9‐related inherited thombocytopenias (along with Sebastian, Fechtner, and Epstein syndromes), is a rare hereditary condition characterized by the triad of thrombocytopenia, giant platelets, and inclusion bodies in leukocytes.63 Clinical symptoms include mild bleeding, and possible kidney dysfunction, deafness, and cataracts later in life.
Acquired Platelet Disorders—Systemic
An outline of acquired platelet disorders is found in Table 18‐5.
Primary Immune Thrombocytopenia
Primary immune thrombocytopenia (also known as idiopathic thrombocytopenic purpura [ITP] or immune thrombocytopenic purpura) is caused by autoantibodies against platelet antigens.
In reviews from the United States, prevalence was approximately 9.5 per 100,000 persons, with 8 per 100,000 in children, 12 per 100,000 in adults, and an overall female to male ratio of 1.9:1.64,65
ITP is assumed to be caused by accelerated antibody‐mediated platelet consumption. While genetic factors are considered for the onset of ITP, some cases have inciting events, either infections and/or systemic conditions that disrupt immune function.
Viral infections are the most common infectious cause. Human immunodeficiency virus (HIV), hepatitis C virus (HCV), cytomegalovirus (CMV), and varicella zoster virus have been implicated. It has been proposed that antibodies against viral antigens may cross‐react with normal platelet antigens.66,67,68 Bacterial infection is a less common trigger, with proposed mechanisms including lipopolysaccharide attachment to platelet surfaces and increased platelet phagocytosis, molecular mimicry via antibodies, immune alterations, and activities of bacterial products.69,70 Immune‐mediated thrombocytopenia may occur in conjunction with HIV disease in approximately 15% of adults, being more common with advanced clinical disease and immune suppression, although less than 0.5% of patients have severe thrombocytopenia with platelet counts below 50,000/mm3.71
In autoimmune disorders, the development of autoantibodies occurs, as seen in antiphospholipid syndrome (APS), SLE, Evans syndrome, hematopoietic cell transplantation, chronic lymphocytic leukemia (CLL), and other low‐grade lymphoproliferative disorders.72,73 Antibody production in ITP is driven by CD4‐positive helper T cells reacting to platelet surface glycoproteins, with splenic macrophages being the major antigen‐presenting cells.74,75 Autoimmune thrombocytopenia is found in patients with SLE, with mild thrombocytopenia (platelet counts between 100,000 and 150,000/mm3) noted in 25%–50% of patients and severe thrombocytopenia (platelet counts <50,000/mm3) in 10% of patients.76 Severe bleeding from ITP due to SLE is only experienced by a minority of patients; however, when it occurs it requires aggressive treatment.77
Many patients with ITP are asymptomatic and overall risk of bleeding is low. Symptomatic patients can have fatigue and reduced quality of life. Bleeding may occur in up to two‐thirds of patients. Clinical symptoms include petechiae and purpura over the chest, neck, and limbs, usually more severe on the lower extremities. Mucosal bleeding may occur in the oral cavity and GI and genitourinary tracts. In severe cases of ITP, oral hematomas and hemorrhagic bullae may be the presenting clinical sign (Figure 18‐6).78
There is a lack of sensitive or specific diagnostic tests for ITP and other causes of thrombocytopenia, therefore posing a challenge, relying on a diagnosis of exclusion. Primary diagnostic investigations in patients with suspected ITP should include CBC with differential, peripheral blood smear, and HIV and HCV testing. Secondary tests may include PT, aPTT, Helicobacter pylori testing, antinuclear antibody, and vitamin B12 and folate levels. By consensus, the criteria for ITP is a platelet count of <100,000/mm3, with greatest concern for bleeding with platelet counts <20,000/ mm3.79 Large platelets are often noted on the peripheral blood smear; however, their absence does not exclude the diagnosis. ITP is not accompanied by abnormal numbers or morphology of red and white blood cells, or abnormal coagulation parameters. If any of these are seen, then other conditions should be considered (e.g., infection, leukemia, thrombotic thrombocytopenic purpura [TTP], and DIC).
The goal of ITP therapy is to reduce the risk of clinically significant bleeding. Active interventions are guided by clinical symptoms and the platelet count. The objectives during treatment are to provide a safe, rather than normalized, platelet count.80
For ITP patients with severe bleeding, treatment is immediate platelet transfusion along with IV immunoglobulin (IVIg) and high‐dose corticosteroids. For a new diagnosis of ITP and a platelet count <20,000/mm3, IVIg and corticosteroids are also indicated to prevent thrombocytopenia from persisting and worsening. Target platelet counts are between 20,000 and 30,000/mm3, except in the case of those with a history of bleeding at higher counts, other hemostatic defects, those requiring surgery, or at increased risk of bleeding in general (e.g., peptic ulcer, high activity, high risk of falls).
Splenectomy may be necessary in chronic ITP to prevent antiplatelet antibody production and sequestration and removal of antibody‐labeled platelets.81
ITP may be acute and self‐limiting (2–6 weeks) in children. In adults, ITP is typically more indolent in its onset, and the course is persistent, often lasting many years, and may be characterized by recurrent exacerbations of disease. The natural history and long‐term prognosis of adults with chronic ITP remain incompletely defined.82
Platelet count thresholds for surgery are higher than those to prevent spontaneous bleeding. While there are no published guidelines for oral and maxillofacial surgery, 50,000/mm3 has been the recommended threshold for most major surgery, and as high as 100,000/mm3 for neurosurgery or ocular surgery83 with lower values (i.e., 20,000/mm3) acceptable for less invasive procedures such as central line placement. A recent systematic review failed to find evidence to support this 50,000/mm3 threshold for the safety of invasive dental procedures in thrombocytopenic patients.84
Thrombotic Thrombocytopenic Purpura
TTP is a rare acute disease that, until recently, was uniformly fatal. It can be hereditary or acquired due to an autoantibody inhibitor. Incidence of acquired TTP is 3 cases per million adults per year, and 1 per 10 million per year in children.85 It is a thrombotic microangiopathy caused by severely reduced activity of the VWF‐cleaving protease ADAMTS13. Small‐vessel platelet‐rich thrombi form that cause thrombocytopenia, microangiopathic hemolytic anemia, and sometimes organ damage. Causes include metastatic malignancy, pregnancy, mitomycin C, and high‐dose chemotherapy. If untreated, it carries a high mortality rate.
Initial presentation may include fatigue, dyspnea, petechiae, or other bleeding. In addition to thrombocytopenia, clinical presentation of TTP includes GI symptoms, weakness, and neurologic findings ranging from coma, stroke, and seizure to headache and confusion. Microangiopathic hemolytic anemia, fluctuating neurologic abnormalities, renal dysfunction, and occasional fever also can occur. Microvascular infarcts due to the thrombi occur in gingival and other mucosal tissues and are present in about 60% of cases. Serial studies of plasma samples from patients during episodes of TTP have often shown VWF multimer abnormalities.86
Although there are numerous therapeutic options, there is no consensus among experts or clear algorithms to treat TTP. Plasma exchange therapy combined with acetylsalicyclic acid (ASA)/dipyridamole or corticosteroids have recently lowered the mortality rate for patients with TTP over that previously obtained by treatment with fresh frozen plasma (FFP) infusions.87,88 In addition, there is a role for newer therapies with diverse mechanisms of action, such as rituximab, anti‐D, and thrombopoietin‐like agents.89
Liver Disease
Patients with liver disease may have a wide spectrum of hemostatic defects, depending upon the extent of liver damage, which affect both the platelet and coagulation phases of hemostasis.90 These changes increase the risks of both bleeding and thrombosis.
Liver disease, both chronic and acute, results in both quantitative and qualitative platelet defects. Chronic liver disease with cirrhosis leads to thrombocytopenia due to portal hypertension, and sequestration and breakdown of platelets by the spleen, in addition to decreased thrombopoietin production. Thrombocytopenia in acute hepatitis is hypothesized to be linked to systemic inflammatory response syndrome and resultant multiorgan system failure.91 Liver failure may also be considered as a potential cause of unexplained thrombocytopenia.92 For more on clinical manifestations, diagnosis, and management of patients with liver disease, see the section on liver disease–associated coagulation disorders.
Chronic Kidney Disease
The failure of the kidney to properly eliminate the breakdown products of proteins results in increased levels of urea and creatinine in the blood, creating a uremic bleeding risk, which, although multifactorial in pathogenesis, is largely related to platelets. Proposed mechanisms for platelet disorders in chronic kidney disease (CKD) include platelet metabolic defects, deficiencies in platelet‐endothelial interactions, and the effect of anemia on normal platelet function.93 With anemia, it is proposed that the decreased number of red blood cells allows platelets to be more dispersed within the lumen of blood vessels, making them less likely to adhere to the endothelium and form a platelet plug in the event of injury. In addition, anemia may also affect ADP and TXA release, circulating nitric oxide (an inhibitor of platelet aggregation), and cyclic guanosine monophosphate concentrations.94 In addition to platelet disorders, bleeding tendencies in CKD patients are also increased due to coexisting coagulopathies and the heparin use during hemodialysis. Ecchymoses, epistaxis, and GI and genitourinary bleeding are commonly seen in renal failure patients. Spontaneous intraoral mucocutaneous bleeding can also occur.
The routine use of dialysis has decreased the incidence of spontaneous bleeding episodes.95 While the severity of CKD does not directly correlate with abnormal platelet aggregation, the degree of anemia secondary to decreased renal erythropoietin production due to CKD does. Therefore, correction of anemia with blood transfusions, erythropoietin, or erythropoiesis‐stimulating agents reduces the bleeding time in many patients; however, it does not decrease the incidence or risk of bleeding.96 Desmopressin (DDAVP) is an analog of antidiuretic hormone with vasopressor activity. It appears to work by increasing the release of F VIII/VWF multimers from endothelial cells and increase platelet membrane glycoprotein expression.97 DDAVP is administered at a dose of 0.3 μg/kg IV (in 50 mL of saline over 15–30 minutes), or 3 μg/kg intranasally, as in Stimate nasal spray (one spray per nostril, 150 μg each spray) 1–2 hours before procedure. Improvement in BT is seen in approximately 1 hour, and lasts 4–8 hours.98 Conjugated estrogens, 0.6 mg/kg IV daily for 5 days, or 2.5–25 mg orally per day, or 50–100 μg of transdermal estradiol twice weekly, are most commonly indicated in patients on dialysis who have chronic GI tract bleeding when other treatment is contraindicated.99 Cryoprecipitate is thought to enhance platelet aggregation by increasing F VIII/VWF multimers and fibrinogen. When administered IV (10 units every 12–24 hours), BT improves in uremic patients, beginning in 1 hour and lasting for 4–24 hours.100
Cardiopulmonary Bypass
Cardiopulmonary bypass (CPB), also known as a heart–lung machine or extracorporeal membrane oxygenation (ECMO), causes significant platelet dysfunction due to platelet interaction with nonphysiologic surfaces of the bypass machine, complement activation, release of cytokines, thrombin generation, and hypothermia during bypass. Collectively, these lead to premature platelet activation, secretion of contents, and alteration in surface glycoprotein expression (decreased GPIb and IIb/IIIa), leading to compromised adhesion, activation, and aggregation. Due to the dysregulated activation and secretion of contents, platelet–neutrophil and platelet–monocyte conjugates are formed, thought to be central in a post‐CPB inflammatory syndrome. Thrombocytopenia also occurs in CPB due to hemodilution, and adherence of platelets to nonphysiologic surfaces in the machine. Bleeding occurs in 30%–50% of patients on CPB and this can be life‐threatening.101
Dysproteinemia
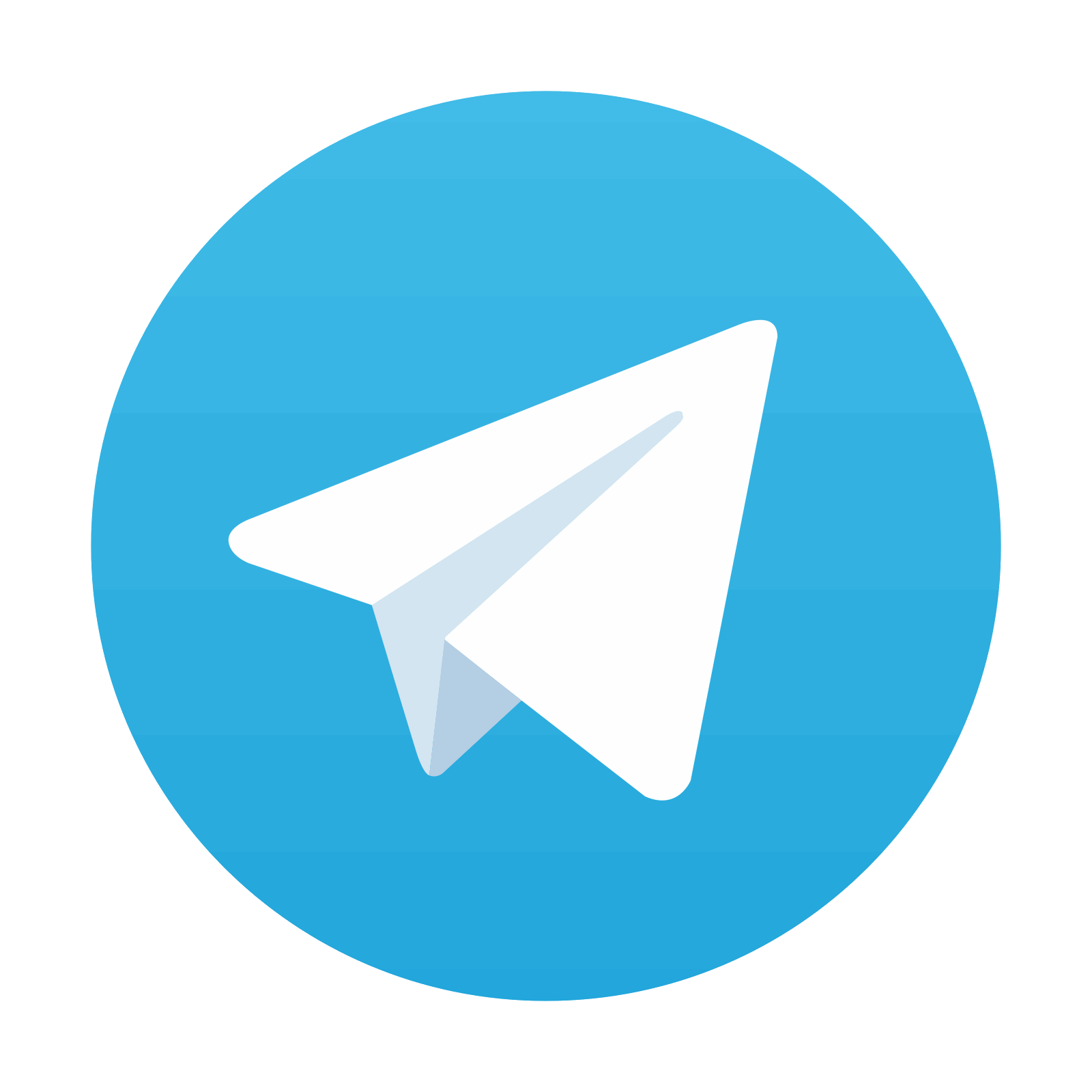
Stay updated, free dental videos. Join our Telegram channel

VIDEdental - Online dental courses
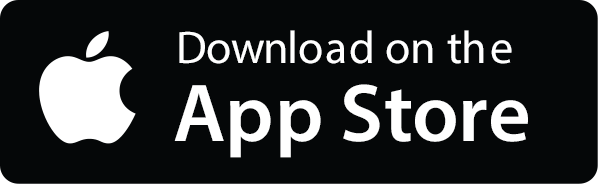
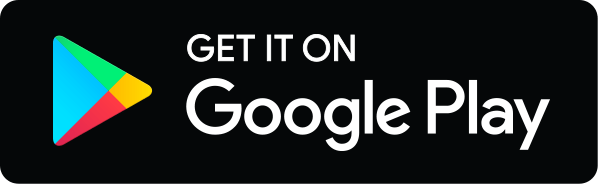