Abstract
Objective
To investigate the contributions of BisGMA:TEGDMA and filler content on polymerization stress, along with the influence of variables associated with stress development, namely, degree of conversion, reaction rate, shrinkage, elastic modulus and loss tangent for a series of experimental dental composites.
Methods
Twenty formulations with BisGMA:TEGDMA ratios of 3:7, 4:6, 5:5, 6:4 and 7:3 and barium glass filler levels of 40, 50, 60 or 70 wt% were studied. Polymerization stress was determined in a tensilometer, inserting the composite between acrylic rods fixed to clamps of a universal test machine and dividing the maximum load recorded by the rods cross-sectional area. Conversion and reaction rate were determined by infra-red spectroscopy. Shrinkage was measured by mercury dilatometer. Modulus was obtained by three-point bending. Loss tangent was determined by dynamic nanoindentation. Regression analyses were performed to estimate the effect of organic and inorganic contents on each studied variable, while a stepwise forward regression identified significant variables for polymerization stress.
Results
All variables showed dependence on inorganic concentration and monomeric content. The resin matrix showed a stronger influence on polymerization stress, conversion and reaction rate, whereas filler fraction showed a stronger influence on shrinkage, modulus and loss tangent. Shrinkage and conversion were significantly related to polymerization stress.
Significance
Both the inorganic filler concentration and monomeric content affect polymerization stress, but the stronger influence of the resin matrix suggests that it may be possible to reduce stress by modifying resin composition without sacrificing filler content. The main challenge is to develop formulations with low shrinkage without sacrificing degree of conversion.
1
Introduction
In recent years, polymerization stress has gained considerable attention from researchers and manufacturers as potentially being one of the main causes for interfacial debonding of composite restorations . Though a definite association between interfacial gap and secondary caries is yet to be established clinically, significant correlations between gap size and lesion depth or mineral loss around composite restorations were found in vitro .
Studies employing commercial materials reported correlations between polymerization stress and composite shrinkage and elastic modulus . However, it is virtually impossible to draw conclusions regarding the influence of organic content or interaction effects between matrix and filler content testing proprietary formulations. Studies using experimental materials reported a negative correlation between stress and base-monomer:diluent ratio and positive correlations between stress and shrinkage or degree of conversion . A negative correlation between stress and inorganic content was verified among composites with similar organic phase . However, no study has systematically evaluated the effect of concomitant variations in both organic and inorganic contents.
Reaction rate and viscoelastic behavior have been associated with polymerization stress development, with controversial results. While some studies verified a reduction in stress with composites cured at slower rates , others reported little to no effect . Considering all the variables involved, establishing which factors significantly affect stress development, as well as the influence of composite organic and inorganic content, would provide helpful information for interpreting results from commercial materials, as well as providing guidance for the development of new composite formulations with low contraction stress.
This study aimed at determining the influence of BisGMA:TEGDMA ratio and inorganic content on polymerization stress and related variables, namely, degree of conversion, reaction rate, volumetric shrinkage, flexural modulus, and loss tangent. The response variables showing a significant relationship with polymerization stress were identified by regression analysis. The working hypotheses were that: (1) both matrix and filler influence the tested variables and (2) polymerization stress is significantly related to the other variables.
2
Materials and methods
2.1
Composite formulations
Twenty formulations were prepared containing BisGMA (2,2-bis[4-(2-hydroxy-3-methylacryloxypropoxy)-phenyl]propane): TEGDMA (2-methyl-2-propenoic acid) molar ratios of 3:7, 4:6, 5:5, 6:4 or 7:3 (ESSTECH Technology Inc., Essington, PA, USA) and 40, 50, 60 or 70 wt% of silanated barium glass particles with irregular shape (average size: 2 μm; density: 2.75 g/cm 3 , Specialty Glass Products, Willow Grove, PA, USA). Camphorquinone and 2-(dimethylamino)ethyl methacrylate (Sigma–Aldrich Inc., St. Louis, MO, USA), 2 mol% each, were used as photoinitiators. Chemicals were used as provided by the manufacturers. Composites were mixed by mechanical mixer (SpeedMixer DAC 150 FVZ, Hauschild Engineering, Alemanha), in order to avoid the incorporation of air bubbles and to obtain a homogeneous mixture. Materials were kept refrigerated until 1 h before testing.
2.2
Polymerization stress
Poly(methyl methacrylate) rods (radius, 3 mm; length, 13 or 28 mm) had one of their flat surfaces coarsened with #180-grit sandpaper, primed with a thin layer of methyl methacrylate liquid and then coated with unfilled resin (Scotchbond Multipurpose Plus; 3 M ESPE, St. Paul, MN, USA), which was light-cured for 40 s (VIP Junior, Bisco Inc., Schaumburg, IL, USA). The opposing surface of the shorter rod was mirror-polished to increase light transmission. The rods were attached to a testing machine (Instron 5565, Canton, MA, USA), with the short rod inserted into a stainless steel hollow fixture that allowed the light guide to contact its polished surface. The composite (1 mm thick) was inserted between the treated surfaces and light-cured through the short rod. The radiant exposure actually reaching the composite was set at 16 J/cm 2 (500 mW/cm 2 × 32 s). Composite height was monitored for 10 min using an extensometer (model 2630-101, Instron) attached to both rods, near the gap with the composite, at a resolution of 0.1 μm. The load cell registered the force necessary to maintain the initial specimen height in opposition to the composite shrinkage force. Maximum nominal stress was calculated by dividing the contraction force by the area of the rod ( n = 7).
2.3
Degree of conversion and maximum polymerization rate (RP max )
Polymerization was monitored for 10 min using near-infra red spectroscopy (Vertex 70, Bruker Optik GmbH, Germany). The composite was inserted in a silicone matrix (radius, 3.5 mm, height, 0.8 mm) and pressed between two glass slides (invisible to near infra-red radiation). Two spectra (two scans each) per sec were collected, with 4 cm −1 resolution. Three spectra were obtained prior to light-curing and used as an uncured reference. The change in area under the 6165 cm −1 absorption peak corresponding to the first overtone of the C–H stretch of the methacrylate group was used to calculate the degree of conversion according to the formula ( n = 5):
For each specimen, RP max was calculated as the first derivative of the conversion versus time curve.
2.4
Volumetric shrinkage
A mercury dilatomer was used to determine composite volumetric shrinkage at 10 min after photopolymerization (ADAHF, Gaithersburg, MD, USA, n = 3). Approximately 50 mg of composite was inserted into a glass tube (radius, 2 mm, height, 2 mm) bonded to a glass slide. The glass slide was clamped to a glass column, which was carefully filled with mercury. A linear variable differential transducer probe was placed on the mercury surface. Photoactivation was conducted through the glass slide (500 mW/cm 2 × 32 s). The probe readings were used to calculate shrinkage based on the mass and specific gravity of the specimen (measured on the cured specimen using Archimedes method).
2.5
Flexural modulus
Bars (12 mm × 2 mm × 1 mm) were made using a silicone mold ( n = 10). Pressure was applied to glass slides over mylar strips covering the surfaces of the composite that was confined within the mold to ensure smooth surfaces and a consistent thickness in all specimens. Ten minutes after photoactivation (500 mW/cm 2 × 32 s), the specimens were subjected to three-point bending, at 0.5 mm/min crosshead speed (QTest, MTS System Corporation, NC, USA). Flexural modulus ( E , in GPa) was calculated from data obtained from the initial linear portion of the load-displacement curve, according to the formula:
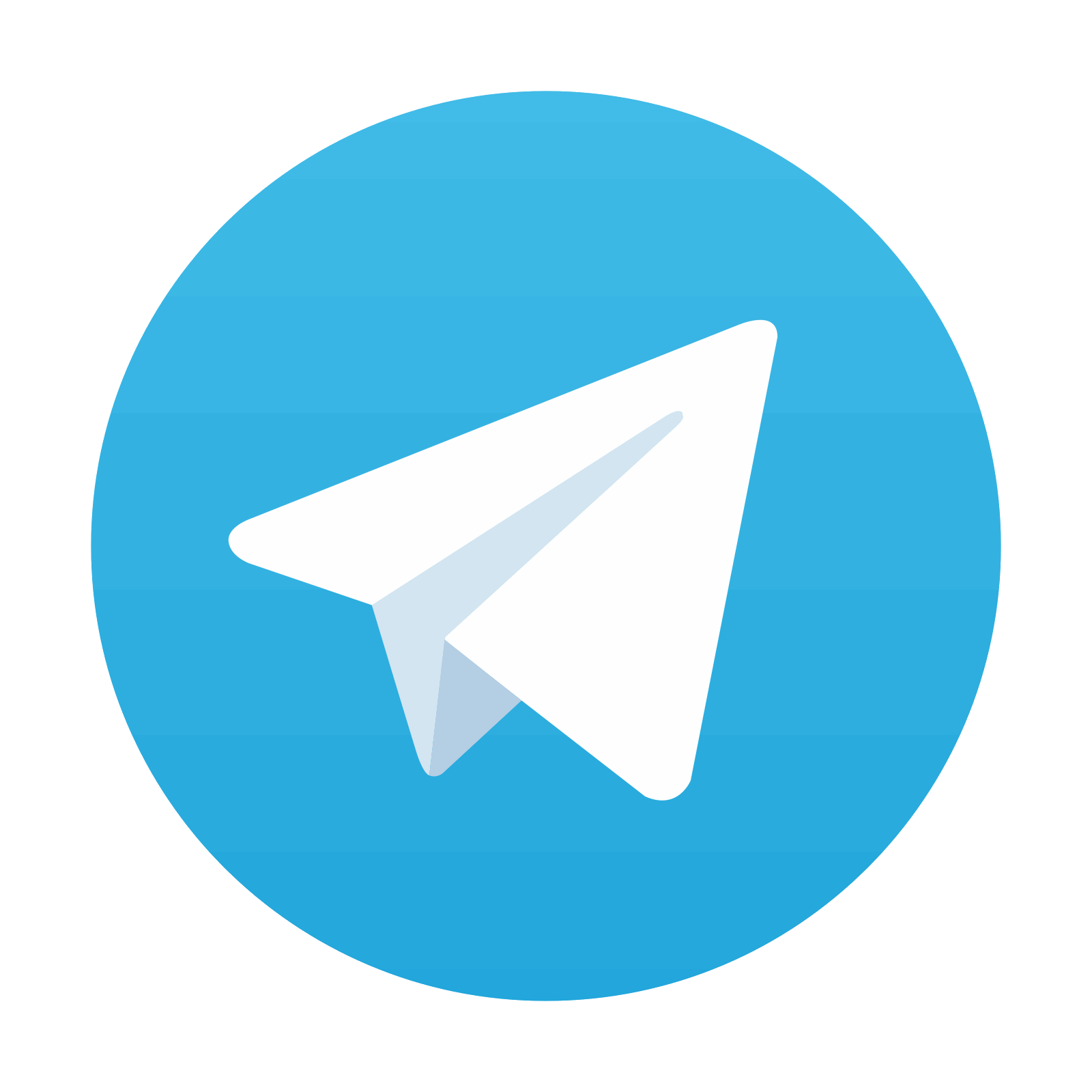
Stay updated, free dental videos. Join our Telegram channel

VIDEdental - Online dental courses
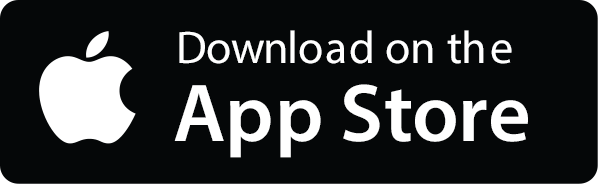
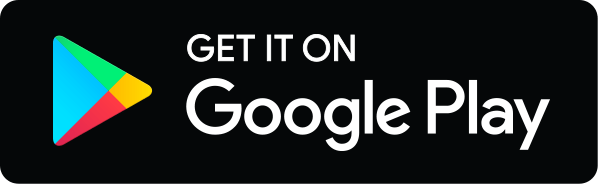