Abstract
Obstructive sleep apnea (OSA) is a disorder characterized by repetitive, episodic collapse of the pharyngeal airway. Over the last two decades, understanding of the pathophysiology of sleep disordered breathing, which includes OSA, has improved. Once thought to be predominately related to anatomic constriction of the maxillomandibular complex, central nervous system regulation of breathing is now recognized as a significant contributor to the pathogenesis of OSA. Ventilator control, the central response to chemoreceptor phenomena, has important implications for oral and maxillofacial surgeons who treat OSA, particularly for patients who appear refractory to treatment with maxillomandibular advancement (MMA). The purpose of this article is to review the biomechanics of the upper airway as it relates to the pathophysiology of OSA, to discuss emerging concepts of ventilator control mechanisms in normal sleep versus sleep-disordered breathing and to discuss the concept of complex sleep apnea, a new category of sleep disordered breathing with both obstructive and central features.
Obstructive sleep apnea (OSA) is a well-recognized disorder that affects at least 4% of the population in the USA . It is characterized by repetitive collapse of the upper airway, commonly defined as the soft tissue region bounded by the nasopharynx superiorly, the epiglottis inferiorly, the maxillomandibular complex anteriorly and the spinal column posteriorly. Collapse of the upper airway decreases its intra-luminal diameter and increases airway resistance, in accordance with Pouiseille’s Law ( Fig. 1 ). This increased resistance to flow results in reductions in ventilation (hypopneas) or complete cessation of ventilation (apneas), with corresponding metabolic disturbances (respiratory acidosis due to hypoventilation) and sleep fragmentation. Compensation for metabolic disturbances occurs primarily in the form of alterations in respiratory effort, which is the primary determinant of arousals from sleep. While brief transitions to the wake state provide ventilator compensation to correct blood gas abnormalities, this comes at the cost of further destabilizing respiratory control at the sleep–wake interface. Concomitant activation of the sympathetic nervous system results in increases in blood pressure, which, over the long term, have been linke to increased risk for sudden death, stroke and myocardial infarction . Sleep-event related arousals produce fragmentation of sleep patterns, resulting in excessive daytime somnolence, neurocognitive impairment and increased risk for accidents related to sleep deprivation .

A significant focus in the oral and maxillofacial surgical literature has been to identify anatomic parameters related to OSA and to determine the effect of surgical intervention (e.g. maxillomandibular advancement, MMA) on airway anatomy . The success of MMA in patients with purely obstructive disease has been well documented . The small percentage (10–20%) of patients who fail to respond to MMA are often presumed to have a component of central apnea or are labelled as ‘refractory’ to treatment if the anatomic goals of MMA have been achieved .
Recent advances in understanding the pathophysiology of sleep apnea are inconsistent with the classical notion of OSA as an anatomic disorder . The complex interplay between anatomic, sleep-related and central nervous system factors may explain the variable results seen after MMA ( Fig. 2 ). Specifically, a subset of patients with sleep apnea have been found who challenge two commonly held beliefs: that (1) central and obstructive apneas are independent processes and (2) poor sleep is a secondary result of OSA rather than a primary cause. The study of these patients considered to have ‘complex sleep apnea’, has shed light on the variable pathophysiology of sleep apnea.

The purpose of this article is to review the factors that contribute to upper airway collapse, to introduce the role of ventilator control on the pathogenesis of OSA and to describe the phenomenon of complex sleep apnea.
Upper airway patency and collapse
Patency of the upper airway is insured by a balance between collapsing forces and dilating forces ( Table 1 , Fig. 3 ) . Akin to Starling’s Law for fluid flow within capillaries, the upper airway can be described as a Starling resistor, the caliber of which is determined by the pressure differential between the collapsing forces and dilating forces, P crit ( Fig. 4 ) . Collapsing forces are related to intra-luminal upper airway pressures during inspiration and the composition of the pharyngeal wall. Dilating forces are predominately related to pharyngeal dilator muscle tone, with contributions from airway traction during inspiration, and increases in lung volume increases .
Dilating forces | Collapsing forces |
---|---|
Pharyngeal dilator muscle activity | Negative intra-luminal pressure during inspiration |
Airway traction from lung expansion | Pressure from parapharyngeal tissue |


Collapsing factors
Collapsing forces on the airway can be broadly classified as those acting within the airway (intra-luminal) and those that act on the airway wall itself (extra-luminal). These factors result in decreased intra-luminal diameter and obstruction due to increased resistance to airflow. Conceptually, the threshold pressure required for airway collapse can be determined using pressure-flow curves . Nasal airway pressure required to maintain airway patency is defined as the critical closing pressure ( P crit ). Numerous studies have demonstrated that P crit is related to anatomical features and lung volumes. Anatomically, P crit has been shown to correlate with soft palate length in obese patients and airway length and hyoid-mandibular distance in non-obese patients . These findings correlate with those reported in the surgical literature, which demonstrate that patients with OSA have longer soft-palates and longer airways . Since pressure is the force applied over a given cross-sectional area, an increase in the critical closing pressure (i.e. an increased propensity for collapse) is seen in individuals with narrower and longer airways . Since P crit is influenced by a number of anatomic factors, it is likely that P crit varies with individual patient characteristics as noted above. This may explain the observation that some patients with large diameter airways have severe OSA, while patients without OSA can have relatively small airways. Thus, it is plausible that the magnitude of MMA required to improve or eliminate OSA may be related to these individual anatomic characteristics rather than to a fixed number of millimeters.
Intra-luminal forces acting to collapse the airway are related to pressure changes during respiration and vary by respiratory patterns. In the upper airway, pressure is generated during inspiration and expiration, even though the direction of airflow is different. The caliber of the upper airway is therefore dependent on pressure within the airway lumen ( P lumen ). During inspiration, the volume of the thorax increases, and the pressure decreases, allowing for airflow into the lungs, but the decrease in pressure results in a relative negative intra-luminal pressure within the upper airway, predisposing toward collapse. Collapse is generally prevented in these patients due to reflex activation of dilator muscles, as discussed below.
During expiration, intra-luminal pressure initially increases, which acts to dilate the upper airway. At the end of expiration, intra-luminal pressure begins to fall to a point at which the airway can collapse. Such collapse occurs in the setting of extra-luminal factors, discussed below, which affect the elastance of the pharyngeal airway. Airway cross-sectional area is lowest at the end of expiration. Expiratory narrowing is more significant in patients with OSA than in normal controls .
The second set of factors responsible for upper airway collapsibility is related to extra-luminal pressure or pressure from the tissues that comprise and support the pharyngeal airway. The upper airway is a flexible structure composed of varying amounts of muscular and adipose tissue. Though some of the pharyngeal musculature is attached to the facial skeleton or vertebral column, the upper airway is, for the most part, only passively supported by bony structures. This relative lack of rigid support causes the upper airway to behave like a collapsible cylinder with well-defined anatomic factors that predispose to upper airway collapse. These factors can be broadly classified as soft tissue, myoneural and skeletal.
Soft tissue factors include fat deposition within the parapharyngeal structures, pharyngeal edema/inflammation, adenotonsillar hypertrophy and macroglossia . Imaging studies of obese patients with OSA have demonstrated increased adipose tissue in the lateral pharyngeal fat pads, tongue and soft palate. This has been hypothesized to increase tissue pressure on the pharyngeal wall, predisposing toward collapse . In addition, deposition of fat within the airway wall has been shown to increase overall mass within these tissues, which may also increase tissue pressure, resulting in airway collapse and decreased airway volume . Pharyngeal edema likewise results in decreased airway caliber and has been shown to be increased in patients who snore and those with apnea . Fluid secretions on the airway surface have been shown to contribute to collapse in OSA patients by increasing wall tension .
There has been increasing interest in the role of ‘myoneural factors’ (i.e. changes in the sensory and neural structures influencing function of the upper airway) . These include increased sensory thresholds and two-point discrimination, evidence of injury to intra-epithelial nerve endings, and myopathic changes . Such changes may contribute to disease progression. Direct effects of snoring vibrations have also been proposed to play a role .
Skeletal factors that influence upper airway caliber are those most often corrected by the oral and maxillofacial surgeon and include maxillary, mandibular and hyoid bone position. Patients with OSA typically have mandibular or bimaxillary retrognathism and a low-lying hyoid . Skeletal size can also play an indirect role in maintaining the airway. Among patients without OSA, there is a relative concordance between the size of the skeletal airway enclosure (maxillomandibular complex and spinal column) and the soft tissue airway ( Fig. 5 ) . In patients with OSA, a narrow airway can result from excessive soft tissue on a normal skeleton or from normal amounts of soft tissue on a deficient skeleton .

Upper airway patency – dilating factors
Collapsing forces related to soft tissue and skeletal abnormalities are typically offset by pharyngeal dilator muscle activity. Though there are a number of pharyngeal muscles that act to dilate the airway, the genioglossus is the largest airway dilator and the most extensively studied . The genioglossus is an extrinsic muscle of the tongue that attaches to the lingual aspect of the mandible. When it contracts, the tongue moves anteriorly and the pharyngeal airway dilates at the retroglossal level . The motor nerve of the genioglossus is the hypoglossal nerve and its activity is modulated by: respiratory pattern generation; pressure receptors within the pharyngeal wall; and central and peripheral chemoreceptors ( Fig. 6 ) .
Medullary neurons associated with respiratory control regulate the action of the genioglossus muscles. On inspiration and the generation of negative intra-luminal pressure, mechanoreceptors within the pharyngeal wall transmit afferent information via the superior branch of the internal laryngeal nerve, which acts via a reflex pathway to cause genioglossus contraction . This provides a counteracting force to prevent airway collapse . Application of topical local anesthetics can induce airway collapse supporting the concept that airway patency is also maintained by baseline genioglossus muscle tone . Neurons responsible for modulating arousal generally increase genioglossus activity, linking pharyngeal dilation with the arousal state .
It has been demonstrated that pharyngeal muscle dilator activity, as a reflex response to negative intra-luminal pressure, is normal or slightly elevated among awake patients with OSA. This is presumably due to the increased inward collapsing force resulting from anatomic factors . However, during sleep, particularly non-rapid eye movement (NREM) sleep, this reflex activity is significantly diminished or lost . This may result in inspiratory narrowing of the airway in patients with OSA during NREM sleep.
In addition to pharyngeal dilator muscle activity, pharyngeal patency is influenced by caudal traction on the airway due to lung expansion . Expansion of the thoracic cavity during inspiration applies an inferior stretching force on the airway walls, creating tension within the wall that opposes the negative intra-luminal pressure and prevents collapse. Thus, decreases in lung volumes, which can be associated with parenchymal disease or obesity, as well as with supine posture and the transition to sleep, generally reduce tension on the airway and promote collapse .
Upper airway patency – dilating factors
Collapsing forces related to soft tissue and skeletal abnormalities are typically offset by pharyngeal dilator muscle activity. Though there are a number of pharyngeal muscles that act to dilate the airway, the genioglossus is the largest airway dilator and the most extensively studied . The genioglossus is an extrinsic muscle of the tongue that attaches to the lingual aspect of the mandible. When it contracts, the tongue moves anteriorly and the pharyngeal airway dilates at the retroglossal level . The motor nerve of the genioglossus is the hypoglossal nerve and its activity is modulated by: respiratory pattern generation; pressure receptors within the pharyngeal wall; and central and peripheral chemoreceptors ( Fig. 6 ) .
Medullary neurons associated with respiratory control regulate the action of the genioglossus muscles. On inspiration and the generation of negative intra-luminal pressure, mechanoreceptors within the pharyngeal wall transmit afferent information via the superior branch of the internal laryngeal nerve, which acts via a reflex pathway to cause genioglossus contraction . This provides a counteracting force to prevent airway collapse . Application of topical local anesthetics can induce airway collapse supporting the concept that airway patency is also maintained by baseline genioglossus muscle tone . Neurons responsible for modulating arousal generally increase genioglossus activity, linking pharyngeal dilation with the arousal state .
It has been demonstrated that pharyngeal muscle dilator activity, as a reflex response to negative intra-luminal pressure, is normal or slightly elevated among awake patients with OSA. This is presumably due to the increased inward collapsing force resulting from anatomic factors . However, during sleep, particularly non-rapid eye movement (NREM) sleep, this reflex activity is significantly diminished or lost . This may result in inspiratory narrowing of the airway in patients with OSA during NREM sleep.
In addition to pharyngeal dilator muscle activity, pharyngeal patency is influenced by caudal traction on the airway due to lung expansion . Expansion of the thoracic cavity during inspiration applies an inferior stretching force on the airway walls, creating tension within the wall that opposes the negative intra-luminal pressure and prevents collapse. Thus, decreases in lung volumes, which can be associated with parenchymal disease or obesity, as well as with supine posture and the transition to sleep, generally reduce tension on the airway and promote collapse .
Ventilator control: loop gain
While the coordination between collapsing and dilating forces is an important concept in the pathogenesis of OSA, there is increasing evidence that the quantity and pattern of ventilation plays a substantial role in airway collapse ( Fig. 7 ) . Though patients with OSA generally have narrower and longer airways that are more prone to collapse, not all individuals with such features have OSA. Conversely, not all patients with OSA have narrow airways. For example, some patients with OSA may have larger airways, which are less prone to collapse. Airway narrowing occurs due to negative intra-luminal pressures generated during inspiration. Airway collapse occurs at the end of expiration, with noted increased collapsibility in patients with longer expiratory times. Thus, ventilator control plays an important role in the pathogenesis of OSA.
The feedback loop that links respiratory control to airway collapsibility can be described in terms of an engineering concept known as loop-gain . Loop-gain describes the relative strength and speed of response to disturbance in a system that is regulated by feedback loops. Loop-gain can be conceptualized as the magnitude of the system response relative to the magnitude of the disturbance. Thus, a high gain system (loop-gain > 1) will respond to any perturbation with disproportionately high vigor and rate (a destabilizing response), whereas a low-gain system (loop gain ≪ 1) will respond proportionally in a stabilizing manner. Loop-gain has been suggested to increase during NREM Stages 1–2 of sleep and decrease during NREM Stages 3 and 4 .
With regard to the pathogenesis of OSA, ventilator responses to obstruction need to be efficient but well-controlled, so as not to cause disruption from sleep. Arousals contribute to ventilator instability and promote upper airway collapsibility . Airway obstruction leads to hypercarbia, which is sensed via peripheral and central chemoreceptors. This elicits a central response to hypoventilation. During sleep, the threshold partial pressure of carbon dioxide (PCO 2 ) which results in ventilator response is increased relative to the threshold during wakefulness . The rate and magnitude of this response is the loop-gain of the system. A high-gain system generates a robust response which, though rapid in generation, takes a long time to produce ventilator stability and periodic breathing occurs ( Fig. 8 ). A low-gain system responds to increased PCO 2 to produce ventilator stability quickly.
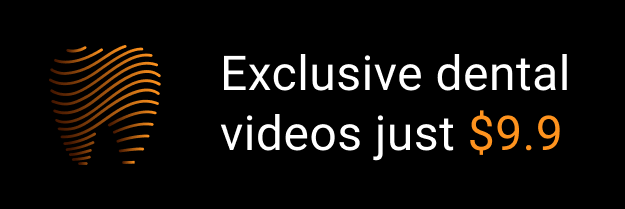