Abstract
The optimal management of mandibular angle fractures remains controversial. The aim of this experimental study was to test the stability and resistance to mechanical force of a new titanium miniplate design. Thirty fresh sheep hemimandibles, sectioned at the angle region, were used to evaluate two plating techniques. One group received fixation via a new design single non-compression titanium miniplate and the second group via a six-hole straight non-compression titanium miniplate. A custom-made biomechanical test model was used for the samples. Each hemimandible was subjected to compressive and tensile forces using an Instron machine. The biomechanical forces ( N ) that caused 4-mm displacement or fixation loosening were compared. Comparison between the groups showed that fixation with the new design miniplate had more resistance to lateral compression forces than with a six-hole straight miniplate ( P < 0.009). Moreover, the new design miniplate fixation displayed more resistance to vertical compression and tensile forces ( P > 0.46 and P > 0.61, respectively). The study demonstrated that mandibular fracture fixation with the new design non-compression titanium miniplate offered greater resistance to lateral displacement forces and may also provide increased resistance to vertical compressive and tensile forces than a conventional six-hole straight miniplate.
Rigid internal fixation with a miniplate has become the standard treatment modality in the management of mandibular fracture. Mandibular angle fractures account for 23–42% of all cases of mandibular fracture. Most commonly, these injuries occur as a result of sports-related accidents, physical assault, motor vehicle accidents, or falls. The frequent involvement of the mandibular angle in mandibular fractures can be attributed to changes in the lines of calcification and strength from the horizontal body to the vertical ascending ramus, the thinner cross-sectional area, and the presence of impacted or partially erupted third molars. In addition, the mandibular angle is regularly subjected to heavy muscle forces. Despite the significant developments that have been made in fixation techniques, the treatment of fractures in the mandibular angle is still associated with a high frequency of complications owing to the biomechanics of this region.
Since the principles first described by Michelet et al. and Champy et al., the placement of a single four- or six-hole monocortical osteosynthesis plate has become an acceptable therapy for mandibular angle fractures. Numerous authors have documented low complication rates with monocortical non-compressive miniplate fixation (Champy technique). However, based on recent experimental and clinical studies, the stability provided by the miniplate fixation of mandibular angle fractures has become a point of contention among surgeons. Various plate and screw systems that are used for the treatment of mandibular angle fractures have been evaluated.
Authors have argued that internal fixation with a passive miniplate along the external oblique line, in accordance with Champy’s ideal osteosynthesis line principle, produces a tension effect that may yield a failure of osteosynthesis or a compromise in stability.
A new non-compression miniplate has been designed that provides similar advantages to the Champy technique: an intraoral approach, a single miniplate, and the use of monocortical screws. This new miniplate differs from others used in the treatment of mandibular angle fractures by displacing the lateral forces that are exerted on the miniplate.
The aim of this experimental study was to evaluate the stability and resistance of a new design non-compression titanium miniplate in mandibular angle fractures.
Materials and methods
Fresh, frozen cadavers of 15 healthy sheep were obtained for the study. All were of similar age (9–11 months) and had been fed a natural diet. All of the mandibles were stripped of their soft tissues and sectioned through the midline, between the central incisors. The specimens were kept moist and refrigerated until all testing had been completed. Hemimandibles ( n = 30) were randomly divided into two groups of 15 (group 1 and group 2). These groups were then fixed with two different plating techniques: (1) the new design non-compression titanium miniplate (group 1), and (2) a titanium six-hole straight non-compression miniplate (group 2). Groups 1 and 2 were then further subdivided into three equal subgroups, groups 1A, 1B, and 1C, and groups 2A, 2B, and 2C, on the basis of the mechanical resistance tests to which each hemimandible would be subjected (vertical, lateral, or tensile).
To simulate the angle fracture, the hemimandibles were sectioned in the mandibular angle region using a diamond disc guided by an acrylic resin template. For all groups, to avoid poor adaptation, the miniplates were pre-bent prior to the hemimandible being cut. After adjustment to the hemimandible, one corresponding hole was drilled in each segment with a standard drill and the plate screwed to the hemimandible. The screw was then removed from the distal segment and a cut was made in the mandibular angle to mimic the angle fracture. The cut segments were reduced, leaving a maximum 1 mm space between the bone segments, as described by Ribeiro-Junior et al. The screw was then replaced at the pre-drilled site in the distal segment. The remaining holes, which had not been drilled prior to the cut, were drilled, and the plate screwed to the hemimandibles on both sides of the cut.
In group 1, all hemimandibles were fixed with a new design single six-hole non-compression titanium miniplate (Trimed Titanium Implant Systems; Trimed, Ankara, Turkey). This plate was produced exclusively for the study by Trimed (grade 4 commercially pure titanium) ( Fig. 1 ). The new miniplate design basically has one straight section and two lateral extensions. The straight section of this new design, which has four holes, was adapted to the superior border of the external oblique ridge. Two lateral extensions, with one hole each, were then bent over to adapt to the buccal cortex of the ascending ramus along the external oblique ridge. The miniplate was secured using six 2.0 × 6-mm monocortical titanium screws (Ti 6 AlV 4 alloy) ( Fig. 2 ). In group 2, all hemimandibles were fixed with a single straight six-hole titanium non-compression miniplate (Trimed Titanium Implant Systems; Trimed, Ankara, Turkey). This miniplate was adapted to the superior border of the external oblique ridge in accordance with the Champy technique and fixed with six 2.0 × 6-mm monocortical titanium screws (Ti 6 ALV 4 alloy) (Trimed Titanium Implant Systems; Trimed, Ankara, Turkey) ( Fig. 3 ).



Different forces were applied to the mandibles in the different subgroups: subgroups 1A and 2A were loaded for the assessment of vertical compressive strength, subgroups 1B and 2B were loaded for the assessment of lateral compressive strength, and subgroups 1C and 2C were loaded for tensile strength by applying a pulling force using holes that had been prepared in the mental area. Force was applied until a 4-mm displacement occurred between the segments, or fixation loosening occurred.
All the hemimandibles were submitted to compression testing in a universal testing machine similar to that designed by Armstrong et al. and further studied by Ribeiro-Junior et al. (Model 4202; Instron, Norwood, MA, USA). This machine uses a testing procedure in which the forces applied by the masticatory muscles are simulated in vitro . The models were positioned in such a way as to allow examination of the resistance of the osteosynthesis methods during simulated mastication. The proximal parts of the hemimandibles were fixed into the loading device, embedded into an acrylic resin box, to prevent any undesirable movement. The load cell was adapted to the testing machine in order to simulate the resistance produced by the food bolus during mastication, for which the compression load was applied in the occlusal region of the second molar ( Fig. 4 ).

Continuous linear compressive loads were applied to the models by the Instron machine until a 4-mm displacement or fixation loosening was observed between the two fractured segments, either vertically or laterally. The value of the load that produced this displacement was recorded for all subgroups. To simulate the tensile (pulling) force, an attachment to the load applicator was created by passing a wire through a hole drilled approximately at the level of the mental foramen. Tensile forces were then applied on the models on the same basis, and the force at which a 4-mm displacement occurred was recorded. A three-dimensional (3D) evaluation of the biomechanical test was obtained by measuring the displacement of the bone segments close to the mandibular base. Accurate measurement was obtained using indentations that were made with a bur in the mandibular base region of each segment. The distance between these indentations was measured prior to each test with a millimetre surgical compass until an increment of 4 mm had been recorded.
All of the quantitative data were analyzed statistically using IBM SPSS Statistics for Windows, version 20.0 (IBM Corp., Armonk, NY, USA). Mean, median, maximum, and minimum values and standard deviations were calculated. The statistical significance of differences between the two groups was evaluated using the Mann–Whitney U -test. For comparisons of the subgroups, the Kruskal–Wallis H -test was used followed by Bonferroni correction. P -values less than 0.05 ( P < 0.05) were accepted as statistically significant.
Results
The maximum loads (kN) required for a 4-mm fragment displacement or fixation loosening are shown in Table 1 . Results are recorded for the application of a lateral or vertical compressive load, or a pulling load on sheep mandible models submitted to mandibular angle fracture and osteosynthesis with two different plate systems.
Subjects | Group 1 (new design miniplate n = 15) | Group 2 (six-hole straight miniplate n = 15) | ||||
---|---|---|---|---|---|---|
Compressive forces (kN) | Tensile forces (kN) | Compressive forces (kN) | Tensile forces (kN) | |||
Vertical | Lateral | Vertical | Lateral | |||
1 | 99 | 299 | 165 | 85 | 18 | 101 |
2 | 101 | 194 | 37.4 | 114 | 112 | 94 |
3 | 122 | 399 | 169.1 | 65 | 96 | 134 |
4 | 170 | 260 | 147 | 119 | 86 | 91.4 |
5 | 98 | 326 | 80 | 102 | 115 | 111 |
Mean ± SD | 118 ± 30.7 | 295.6 ± 76.2 | 119.7 ± 58.4 | 97 ± 22.2 | 119 ± 39.3 | 106.3 ± 17.3 |
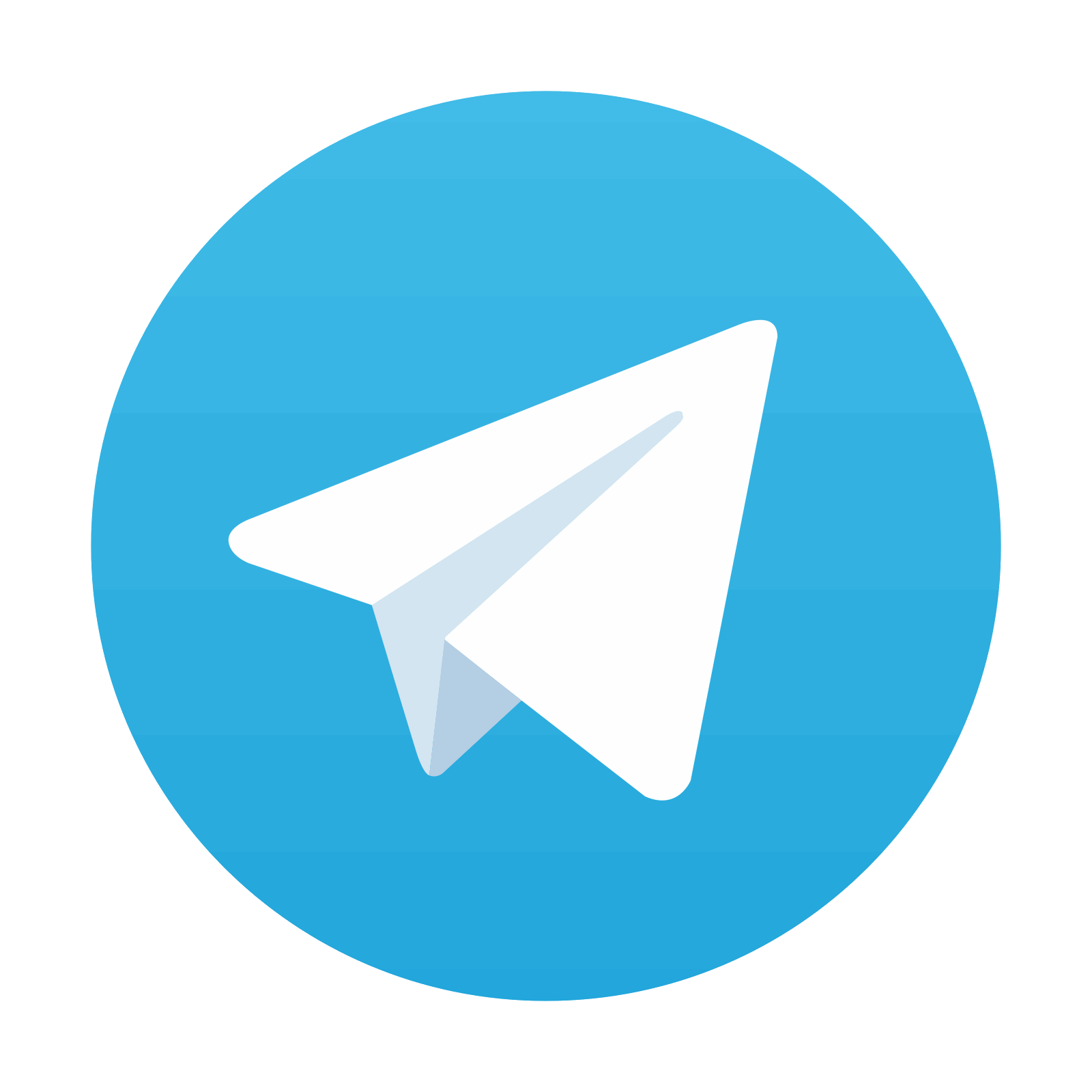
Stay updated, free dental videos. Join our Telegram channel

VIDEdental - Online dental courses
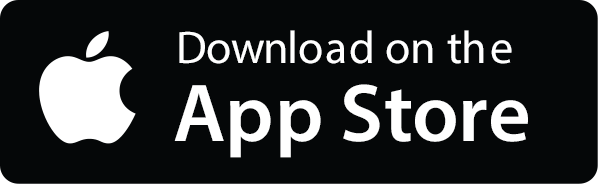
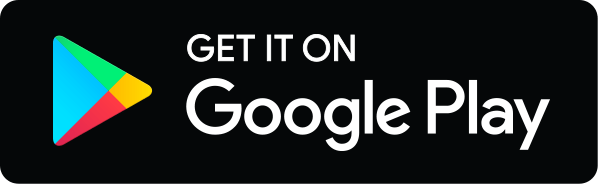
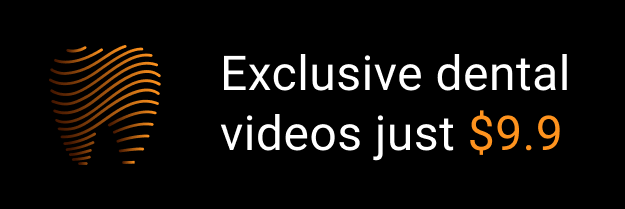