Abstract
Objectives
To evaluate the effect of restoration design (‘2.5-mm deep endocrown’, ‘5-mm deep endocrown’ or ‘5-mm deep post&crown’) and CAD/CAM material type (composite or lithium disilicate glass-ceramic) on the load-to-failure of endodontically treated premolars in absence of any ferrule.
Methods
The crowns of 48 single-rooted premolars were cut and the roots were endodontically treated. Teeth were randomly divided into six groups (n = 8); teeth in each group were restored using one of the two tested materials with standardized CAD/CAM fabricated endocrowns (with either 2.5-mm or 5-mm deep intra-radicular extension) or conventional crowns (5-mm deep post&crown). After cementation using luting composite, the specimens were immersed in distilled water and subjected to 1,200,000 chewing cycles with a load of 50 N applied parallel to the long axis of the tooth (0°). After cyclic loading, a compressive load was applied at 45° to the tooth’s long axis using a universal testing machine until failure. Load-to-failure was recorded (N) and the specimens were examined under a stereomicroscope with 3.5x magnification to determine the mode of failure.
Results
All specimens survived the 1,200,000 chewing cycles. A significant interaction between restoration design and CAD/CAM material was found using two-way ANOVA. In the ‘2.5-mm deep endocrown’ groups, the composite achieved a significantly higher load-to-failure than the lithium disilicate glass-ceramic, while no differences between materials were found in the ‘5-mm deep endocrown’ and ‘5-mm deep post&crown’ groups. More unfavorable failures (root fractures) were observed for higher load-to-failure values.
Conclusions
Only following a ‘2.5-mm deep endocrown’ design, composite appeared more favorable than lithium disilicate glass-ceramic as crown material; this may be explained by their difference in elastic modulus.
Clinical significance
Shallow endocrown preparations on premolars present less surface for adhesive luting and a difference in crown material becomes apparent in terms of load-to-failure. The use of a more flexible composite crown material appeared then a better option.
1
Introduction
The dental practitioner is often faced with a clinical dilemma when deciding how to restore endodontically treated premolars (ETPM) that suffered significant coronal loss. Due to poor structural integrity, those teeth have a higher risk of fracture and controversy exists with regard to the most suitable restoration technique .
In order to retain conventional crowns, the use of intra-root canal post&cores are standardly advocated to provide macro-retention . Unlike metal posts that may weaken the root, fiber posts have an elastic modulus more similar to that of dentin; this characteristic is claimed to reduce the risk of catastrophic root fractures if the tooth is overloaded . Furthermore, the development of effective adhesive luting composites promoted the distribution of occlusal stresses through the remaining tooth structure . The ‘endocrown’ restoration was proposed by Pissis in 1995 as an alternative crown for molars, depending on the availability of remaining tooth structure. The strategy consists of a single monobloc that comprises the entire crown and an intra-radicular extension that fits into the “endo-preparation”. The preparation involves a circular equigingival butt-joint margin and a central cavity in the pulp chamber. All-ceramic endocrowns are retained by adhesive luting, and it is known that ceramics bonded to tooth structure induce a strengthening effect and an increase in fracture resistance . Due to fewer clinical steps, no-post endocrowns seem also more practical in terms of time and costs .
The question that remains to be answered is the suitability of endocrowns to restore ETPM. The preliminary results of a clinical trial conducted by Bindl et al. suggested endocrowns as a promising and efficient treatment method for crown reconstruction of molars and premolars. However, after four and a half years, the same authors considered endocrowns as inadequate for premolars . They reported that this restorative approach was successful for 61 of the 70 restored molars (12% failure), while endocrowns on premolars underwent a higher failure incidence of 31% (5 out of 16 restorations). Loss of adhesion was the only failure reason for the premolars, thus indicating that the surface available for adhesive bonding may not have been large enough. Moreover, the unfavorable ratio between crown basis and crown height might cause a moment of force. Although suggested by Pissis that the cavities must have 5-mm depth, Bindl et al. reported that the depth of the central cavity was not standardized and ranged from 1 to 4 mm. It seems reasonable to hypothesize that the deeper the pulp-cavity preparation for an endocrown and the deeper the resultant intra-radicular extension, the greater the surface area for adhesive retention and the better the transmission of masticatory forces to the root . Yet, there is a lack of data about the influence of the endocrown design on the biomechanical behavior of restored ETPM, and up till now, no studies have investigated the effect of the length of the intra-radicular extension. Another consideration is the use of newer and more flexible composite CAD/CAM milling blocks instead of ceramic ones that were originally described for the endocrown technique and used by Bindl et al. . From a biomimetic perspective, these less brittle composite CAD/CAM blocks exhibit mechanical properties that more closely approximate those of human dentin . Some in vitro studies showed a higher fracture resistance and more favorable fracture mode in molars ; in this way they were also suggested to have great potential for endocrowns. However, a recent study conducted by Gresnigt et al. reported that endocrowns made of composite are more vulnerable under non-axial loading. Ultimately, no studies reported the application of these novel CAD/CAM materials to restore extensively decayed premolars.
Therefore, the objective of this in vitro study was to evaluate the effect of the restoration design (‘2.5-mm deep endocrown’, ‘5-mm deep endocrown’ or ‘5-mm deep post&crown’) and the CAD/CAM material type (composite or lithium disilicate glass-ceramic) on the load-to-failure of restored ETPM. The null hypotheses tested were that the restoration design and the CAD/CAM material type do not have a significant influence on the load-to-failure of restored ETPM.
2
Materials and methods
The brands, manufacturers, types, compositions and batch numbers of the materials used are listed in Table 1 . Forty-eight sound and single-rooted premolars (gathered following the protocol approved by the Commission for Medical Ethics of KU Leuven under file number S57622), stored in 0.5% chloramine, were selected by visual inspection. All teeth had one radiographically visible root canal and similar dimensions at the cemento-enamel junction (CEJ; bucco-lingual: 7.2 ± 1.0 mm; mesio-distal: 5.0 ± 0.5 mm) and a root length of 13 ± 1.0 mm. Teeth with widely curved or atypically shaped roots were excluded.
Brand | Manufacturer | Type | Composition | Batch no. |
---|---|---|---|---|
Cerasmart | GC, Tokyo, Japan | Resin composite CAD/CAM block | Silica (20 nm) and barium glass (300 nm) nanoparticles (71 wt.%), Bis-MEPP, UDMA and DMA polymers (29 wt.%) | 1403101 |
IPS e.max CAD | Ivoclar Vivadent, Schaan Liechtenstein | Lithium disilicate glass-ceramic CAD/CAM block | SiO 2 (57–80%), Li 2 O (11–19%), K 2 O (0–13%), P 2 O 5 (0–11%), ZrO 2 (0–8%), ZnO (0–8%) and other colouring oxides (0–12%) | T08295 |
Fiber Post | GC | 1.6-mm diameter tapered fiber post | Methacrylates and glass fibers (11.85 μm diameter), fibers/matrix ratio: 57.83% | 400001 |
Gradia Core + Self-etching Bond A + B | GC | Dual-curing composite for core build-up & post cementation | 20–30% methacrylic acid ester, 70–75% fluoro-alumino-silicate glass, 1–5% silicon dioxide | 1312201 |
Ceramic Primer II, GC | GC | Ceramic and composite bonding primer | 90–100% ethanol, 1–5% 2,2′-ethylene dioxydiethyl dimethacrylate, 1–5% methacryloyloxydecyl dihydrogen phosphate, <1% (1-methylethylidene) bis[4,1-phenyleneoxy(2-hydroxy-3,1- propanediyl)] bismethacrylate | 1401221 |
Monobond Plus | Ivoclar Vivadent | One component primer | Ethanol, 3-trimethoxysilsylproylmethacrylate, methacrylated phosphoric acid ester | S02028 |
Clearfil Esthetic Cement + ED Primer II A & B | Kuraray Noritake, Tokyo, Japan | Dual-curing composite cement | Paste A: bis-GMA, TEG-DMA, hydrophobic aromatic dimethacrylate, silanated barium glass filler, colloidal silica Paste B: bis-GMA, TEG-DMA, hydrophobic aromatic dimethacrylate, hydrophilic aliphatic dimethracrylate, silanated barium glass filler, silanated silica, colloidal silica, catalysts, dl-camphorquinone, pigments |
590012 |
IPS Ceramic Etching Gel 5% | Ivoclar Vivadent | Ceramic etching gel | Aqueous solution of hydrofluoric acid (<5%) | S51072 |
Aluminum Oxide | Danville Materials, San Ramon, CA, USA | Particles for sandblasting | Aluminum oxide 27-μm particles | 28482 |
The crowns of the teeth were removed by cutting at the level of the CEJ, using a water-cooled, low-speed diamond saw (Isomet 1000, Buehler, Lake Bluff, IL, USA) ( Fig. 1 ). No enamel was left on the remaining roots. They were endodontically treated following a standardized crown-down technique using a rotary files system (Pro-Taper, Dentsply-Maillefer, Konstanz, Germany; X-Smart-Endo-motor, Dentsply-Maillefer). The apical foramen was prepared to size 30 and the root canal was irrigated with 2.5% NaOCl solution after each instrument change. Root canals were dried with paper points and filled with an epoxy resin-based root-canal sealer (Top Seal, Dentsply-Maillefer) and tapered gutta-percha points using a hot continuous-wave condensation technique (System-B Heat Source, Sybron Endo, Amersfoort, The Netherlands). The root access was temporarily filled with composite (Clearfil AP-X, Kuraray Noritake, Tokyo, Japan) and all teeth were stored in distilled water at 37 °C during 24 h.
All roots were covered at the outside with an air-thinned layer (approximately 0.3-mm thick) of latex solution (Erkoskin, Erkodent, Pfalzgrafenweiler, Germany) to simulate the periodontal ligament ( Fig. 1 ). Specimens were then positioned in a cylindrical plastic mold (20-mm high; 10.5-mm diameter) and embedded in methylmethacrylate resin (ClaroCit, Struers, Ballerup, Denmark) at 3 mm from the CEJ to simulate an acceptable biological width between the preparation finish lines and the alveolar bone. The teeth were randomly distributed in accordance with the three different preparation designs ( Fig. 1 ):
- •
‘2.5-mm deep endocrown’: in each root, a standardized 2.5-mm deep central inlay-type cavity was prepared using a 5° tapered and 80-μm grit diamond bur (SBR5 Smooth Cut, GC) mounted in a high-speed air turbine (650, KaVo, Biberach, Germany), which was guided by a custom-made plexiglass matrix (MicroSpecimen Former, University of Iowa, Iowa City, IA, USA). The cavities were oval-shaped with a 2-mm mesial-distal width and a 4.5-mm buccal-palatal width at the top and dentin margins of at least 1-mm wide ( Fig. 1 ). The internal cavo-surface line angle was rounded and the finish lines were polished using a 25-μm grit diamond bur (SBR5f Smooth Cut, GC) and abrasive discs (Sof-Lex 2382C and 2382F, 3 M ESPE).
- •
‘5-mm deep endocrown’: each root was prepared and finished in the same standardized manner as the previous group; however, the depth of the central inlay-type cavity was 5 mm
- •
‘5-mm deep post&crown’: post spaces were prepared at a 5-mm depth into the root canal with a 1.6-mm diameter using a low-speed tungsten bur (Drill Refill, GC, Tokyo, Japan). Each prepared root canal was rinsed with distilled water and dried with paper points. Translucent 4° tapered glass-fiber posts (GC Fiber Post − color code blue, GC) of 1.6-mm coronal and 0.7-mm apical diameters, were cut at 10-mm length from the most apical part, cleaned with alcohol and silanized during 60 s (Ceramic Primer II, GC). The post spaces were treated with a self-etch adhesive (Gradia Core Self-Etching Bond A + B, GC) for 5 s and after 30 s they were dried with medium air-pressure and light-cured for 10 s using a LED curing unit (Bluephase 20i, Ivoclar Vivadent, Schaan, Lichtenstein) with an approximate output of 1200 mW/cm 2 , verified with a radiometer (Bluephase Meter, Ivoclar Vivadent). Posts were cemented using a dual-curing luting composite (Gradia Core, GC) and cured from the top of the posts for 15 s. Standardized cores were built with the same dual-curing composite material, which was dispensed into a custom-made transparent silicon matrix (2.4-mm mesio-distal x 4.4-mm bucco-lingual x 5-mm high; Memosil 2, Heraeus Kulzer, Hanau, Germany). Each post was fully covered by the matrix, which was centrally positioned by the same operator and dentin margins of at least 1 mm in width were ensured. After light-curing during 10 s from each surface (buccal, lingual, mesial, distal and occlusal), the matrix was removed and the core preparation was finished using a 5° tapered and 25-μm grit diamond bur (SBR5f Smooth Cut, GC) and abrasive discs (Sof-Lex 2382C and 2382F, 3 M ESPE, Seefeld, Germany).
A custom-made dental typodont allowed a standardized positioning of the methylmethacrylate cylinders, where teeth were previously fixed. A niche with dimensions identical to those of the cylinders (20-mm high; 10.5-mm diameter), located at the upper second premolar site was provided with a stop at the cervical area. Hence, a tight fit and exact positioning of each sample into the typodont, with the preparation margins of all specimens at the same level, was ensured. Digital optical impressions were taken with a powder-free chairside intraoral scanner (Cerec Omnicam, Sirona, Bensheim, Germany). The camera was rotated from the occlusal aspect to the buccal and lingual sides with an angle to the tooth long axis. Additionally, for the ‘5-mm deep endocrown’ specimens, images of the internal surfaces of the preparations were properly acquired by using a wave motion of the camera in the mesio-distal and bucco-lingual directions. An intact lower jaw typodont was also scanned and used as antagonist for occlusal bite registration. Restorations were automatically designed by the Cerec AC CAD/CAM software (SW 4.3, Sirona) using the Biogeneric Variation of 0.20 ( Fig. 2 ). The restoration-design parameters were: 50-μm spacer, 0-μm occlusal milling offset, 25-μm proximal contact strength, 25-μm occlusal contacts strength, 25-μm dynamic contacts strength, 1000-μm minimal radial thickness, 1000-μm minimal occlusal thickness and 500-μm margin thickness.
Specimens from each preparation design were divided in two groups and restorations were milled from a 14-size CAD/CAM block: composite (Cerasmart, GC) or lithium disilicate glass-ceramic (IPS e.max CAD, Ivoclar Vivadent). All restorations were milled at the same CAM unit (Cerec MC XL, Sirona) using the ‘normal’ milling mode, and immediately luted without use of provisional restorations. This resulted in 6 experimental groups (n = 8) according to the preparation design and the CAD/CAM block type.
The intaglio surface of each restoration was treated according to the manufacturer’s instructions for the respective block material. The composite surface was sandblasted with 27-μm aluminum oxide particles (MicroEtcher CD, Danville Materials, San Ramon, CA, USA), perpendicular to the surface from a distance of 10 mm during 20 s with 0.28-MPa pressure. Remaining particles were removed using a gentle air-blow for 5 s. A silane solution (Ceramic Primer II, GC) was applied and allowed to dry for 60 s. The lithium disilicate glass-ceramic surface was etched with 5% hydrofluoric acid gel (IPS Ceramic Etching Gel (5% HF), Ivoclar Vivadent) for 20 s and then cleaned for 5 min in an ultrasonic bath. A silane coupling agent (Monobond Plus, Ivoclar Vivadent) was applied and allowed to dry for 60 s.
After cleaning the preparations with pumice slurry, followed by drying, the restorations were luted using a dual-curing cementation system (Clearfil Esthetic Cement, Kuraray Noritake). Equal amounts of the self-etch adhesive components were mixed (ED Primer II A & B, Kuraray Noritake), applied on the preparation surface and left for 30 s. The excess was wiped away using mild oil-free air. The two luting composite pastes (Clearfil Esthetic Cement Paste A & B, Kuraray Noritake) were dispensed from the automix syringe on the preparation surface and the inner restoration surface. All specimens were placed under a constant load of 1 kg maintained perpendicularly to the occlusal surface for 5 min. The restoration margins were covered with a glycerin gel (Liquid Strip, Ivoclar Vivadent) to prevent oxygen inhibition of polymerization, and light-cured for 20 s from each side using the same LED curing unit. The cementation lines of the restorations were finished with sandpaper polishing discs (Sof-Lex, 3 M ESPE).
After one-day storage in distilled water at 37 °C, the specimens were subjected to a fatigue aging of 1,200,000 cycles using a chewing simulator machine (SD Mechatronik, Chewing Simulator, Willytec, Munich, Germany). With the specimens positioned into chambers containing distilled water, a load of 50 N was applied with a 6-mm diameter ceramic ball-shaped stylus (Steatite, CeramTec, Plochingen, Germany) in the center of the occlusal surfaces. Load was applied parallel to the long axis of the teeth (0°) at a frequency of 1.6 Hz. The machine was equipped with infrared LVDT (Linear Variable Displacement Transducer) displacement-sensors connected to PC software and able to detect failures due to displacements of 100 μm.
Next, specimens were mounted in a fracture-test setup (5848 MicroTester, Instron, Norwood, MA, USA) with a 45° inclination (oblique to the long axis of the tooth) and loaded in compression until failure. The load was applied towards the inner slope of the buccal cusp using an antagonist 6-mm diameter stainless-steel ball at a crosshead speed of 0.5 mm/min. The maximum load-to-failure was recorded in Newton (N) and mean values were calculated per group. The failure mode was determined under a stereomicroscope with 3.5x magnification and categorized based on a 2-examiner agreement either as ”unfavorable” when a root fracture was observed (which in a clinical situation would require tooth extraction) or ”favorable” when the cause of failure was only de-bonding and/or cohesive fracture of the restoration.
The Shapiro-Wilk test was used to assess normal distribution of the data. As the load-to-failure data (N) were normally distributed (p > 0.05), groups were compared using two-way analysis of variance (two-way ANOVA) for the factors ‘restoration design’ and ‘CAD/CAM material type’, and post-hoc Tukey multiple comparisons at a significance level of p < 0.05. To make sure that the teeth were properly randomized regarding the dimensions, the Pearson’s correlation was calculated. The relationship between load-to-failure and failure mode was analyzed using Student’s t -test (p < 0.05). The data were analyzed with statistical softwares (SPSS 21.0, IBM Corp., Chicago, USA; R Core Team, Foundation for Statistical Computing, Vienna, Austria).
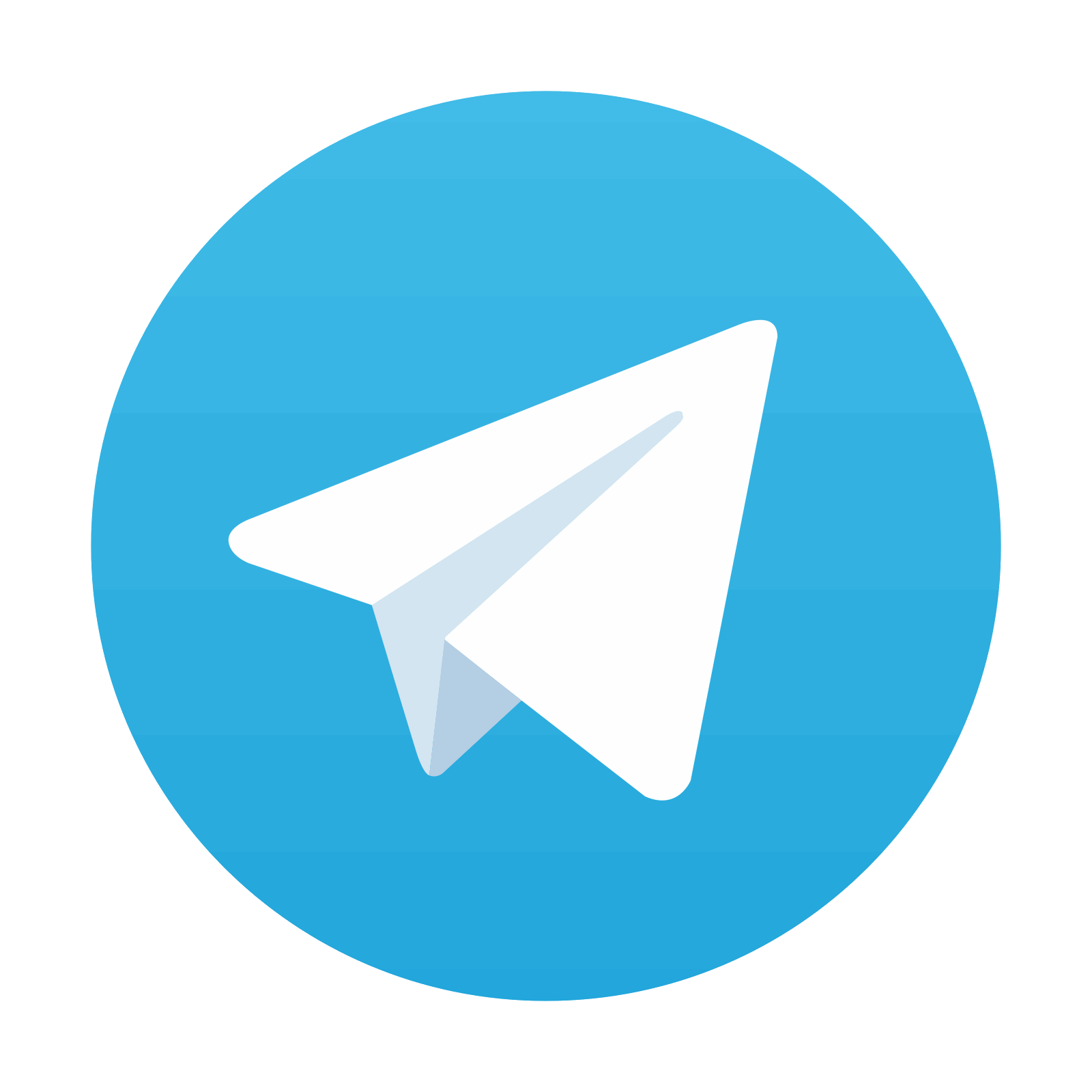
Stay updated, free dental videos. Join our Telegram channel

VIDEdental - Online dental courses
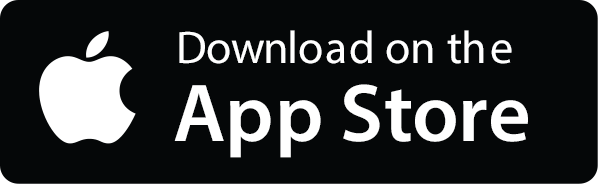
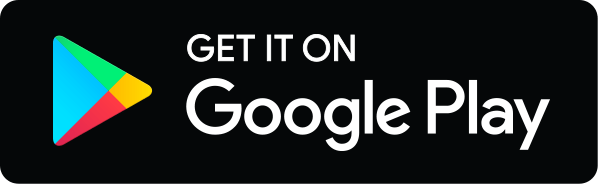