Property
Co-Cr
Ti
Au
GC
Al2O3
YZP
PFR
FRC
Isotropicity
+
+
+
+
+
+
+
+/−
Anisotropicity
−
−
−
−
−
−
−
+/−
Toughness
−
+/−
+/−
−
−
−
−
+
Flexural strength
+
+
+/−
−
+/−
+
−
+a
Isoelasticity with dentine
−
−
−
−
−
−
+/−
+b
In situc processability
−
−
−
−
−
−
+
+
Thermal isolation
−
−
−
+
+
+
+
+
Radiopacity
+
+
+
+/−
+
+
+/−
+/−
CT compatible
−
−
−
+
+
−
+
+
Milling processability
+
+
+/−
+
+
+
+
+
3D printable
+
+
−
−
−
−
+
−
Technical bondingd
+/−
+
+/−
+
−
−
−
+e
Thermal expansion matchf
−
−
−
+
+
−
−
+/−g
Clinically important properties of solid biomaterials which have been used in cranioplasty implants excluding in situ cured bone cements (modified from [94])
Property |
AB |
Titanium |
HA |
TCP |
BGS53P4 |
PEEK |
PMMA |
PE |
FRC-BG |
---|---|---|---|---|---|---|---|---|---|
Resorbability |
+/−a |
− |
+ |
+ |
+ |
− |
− |
− |
+/−b |
Osteoconductivity |
+/−a |
+ |
+ |
+ |
+ |
− |
− |
− |
+ |
Osteoinductivity |
+/−a |
− |
+ |
+ |
+ |
− |
− |
− |
+/−c |
Neovascularization |
+/−a |
− |
+ |
+ |
+ |
− |
− |
− |
+/−c |
Flexural strength >600 MPa |
− |
+ |
− |
− |
− |
− |
− |
− |
+ |
Thermal isolation |
+ |
− |
+ |
+ |
+ |
+ |
+ |
+ |
+ |
Bone-like radiopacity |
+ |
− |
+ |
+ |
+ |
− |
− |
− |
+ |
MRI compatible |
+ |
+/− |
+ |
+ |
+ |
+ |
+ |
+ |
+ |
Antimicrobial |
− |
− |
− |
− |
+ |
− |
− |
− |
+ |
In situ moldable |
− |
+/− |
− |
− |
− |
− |
− |
− |
− |
Overlay structure |
− |
+ |
− |
− |
− |
+/− |
− |
+/− |
+ |
5.2 Dental Applications for FRC
Development of new biomaterials toward clinical use has to follow regulations which are covering medical devices and biomaterials in Europe and worldwide. Risks, which relate to the newly developed biomaterials, can be controlled by selecting the first applications to be short term of use or the device to be removable in nature. Development of FRC has begun by introducing FRC for removable dental prostheses [8–13]. It took almost 40 years until the FRCs were started to be used in larger scale clinically. Delay in getting FRC for clinical use was due to problems in combining resin systems to reinforcing fibers, in difficulties in handling the FRC technically, and in rebuttal of accepting new type of materials by clinical dental profession and dental laboratory technicians. However, development of the FRC with new type of resin systems and understanding of designing principles behind of constructing devices, and the clinical experience, have led to the use of FRCs in a variety of disciplines and applications: in removable prosthodontics [14–18], fixed prosthodontics [19–47], restorative dentistry [46–61], periodontology [39, 62], root canal systems [63–73], orthodontics [74, 75], and in repairs of fixed prostheses [76, 77]. Critical evaluation of the available FRC materials and correct patient selection are of importance for successful use of the material.
What is rationale to use FRCs in dentistry? Although there are several proven dental materials and treatment options based on conventional dental materials, a large number of the partially edentulous patients are not treated by fixed dental prostheses to replace their missing teeth or to repair their damaged biting function. This is often due to high cost of the state-of-the-art type of treatments by fixed prostheses and due to irreversible damage by the treatment when creating space for metal and ceramic crowns by cutting enamel and dentine of abutment teeth. It is paradoxical that the dental profession is removing the most durable bonding site of teeth simply due to limitations of processing properties of conventional and some novel dental materials. Although some novel nonmetallic alternative materials, such as yttria-stabilized zirconia, have become available, the use of zirconia requires cutting equal or even larger amount of enamel and dentine of abutment tooth than by using conventional porcelain-fused-to-metal material combinations.

Stress-strain curves for polymer (matrix), reinforcing fiber (fiber), and fiber-reinforced composite
FRC has properties, which relate to the direction of fibers: FRC can be isotropic, orthotropic, or anisotropic. The mechanical, optical, and thermal properties of the FRC are dependent on the fiber quantity and orientation. A high-quality glass FRC material with high fiber quantity (up to ca. 65 vol%) provides high flexural strength (with E-glass ad 1250 MPa) [66]. A positive correlation exists between water sorption of polymer matrix and the reduction of flexural properties. For instance, high water sorption of polyamide (nylon) matrix causes reduction of over 50% in strength of FRC, but the FRC with less hydrophobic polymer matrix of acrylates has reduction of strength of less than 20% only. The reduction of the flexural properties is reversible, i.e., dehydration of the FRC recovers the mechanical properties. No significant reduction of flexural strength and modulus of elasticity by hydrolytic effect of water even in long-term water storage (ad 10 years) of glass FRC occurs which demonstrates the hydrolytic stability of good quality glass fibers, and their silane coupling agent mediated adhesion with the polymer matrix [78, 79].
The continuous unidirectional fibers provide the highest strength and modulus of elasticity for the FRC, but the property is available only in the direction of stress equal to that of direction of the fibers. Anisotropic behavior of unidirectional FRCs can also be seen in other properties, such as thermal expansion, optical properties, and polymerization shrinkage of the composite [80, 81]. Controlling the polymerization shrinkage by aligned discontinuous glass fibers is utilized in a novel filling composite resin for bilayered resin composite restorations. When the reinforcing effect of the fibers is divided into two or more directions, FRCs are called orthotropic and isotropic with regard to the thermal, optical, and physical properties.
5.3 All Started from Removable Dentures
Research on dental FRCs started in the early 1960s when first experiments on using glass fibers in denture base polymers were made. Glass fibers were selected as the most suitable fibers due to their translucency and possibility to achieve chemical bonding between the fiber and polymer matrix with silane coupling agents. Some tests were made to reinforce denture base polymers also with carbon/graphite fibers and polyethylene fibers. Only little attention was paid during that time to the reasons of limited reinforcing effects of fibers which was later showed to relate fraction of poorly impregnated fibers by powder-liquid-type denture base resins. In the 1990s, studies were published, which showed that highly viscous resin mixture of polymethyl methacrylate (PMMA) powder and monomer liquid was not able to adequately impregnate the fibers [82–84]. Understanding the fundamental problem in resin impregnation leads to the development of preimpregnation system of the reinforcing fibers with porous PMMA. Porous PMMA between the silanized glass fibers behaves as a polymer powder in the acrylic resin mixture. It lowers the polymerization shrinkage of the resin between the fibers, and as the results, well-impregnated FRC was achieved.
The fiber reinforcements in denture bases are divided into two categories. Ladizesky and co-workers reported a method where fibers were distributed through the entire denture base. The approach by Vallittu is based on the concept that only the weakest part of the denture base (location of fracture initiation) is reinforced by precisely aligned and positioned fiber reinforcement. Two fiber-reinforcing concepts are called as total fiber reinforcement (TFR) and partial fiber reinforcement (PFR) [9]. Clinical studies have been performed with FRC-reinforced removable dentures, which suggested that PFR offers an effective and technically easy method to eliminate fractures in denture base [9].
5.4 Next Step: Fixed Dental Prostheses

Example of a minimally invasive glass FRC fixed dental prostheses with three pontics. Prostheses is retained by an inlay in D17 and an inlay with labial bonding wing in D13
Surface-retained FRC FDPs are typically used in anterior and premolar region. The recent laboratory investigations have suggested that optimally designed FRC FDP made on non-prepared abutments can provide even higher load-bearing capacity than conventional porcelain-fused-to-metal FDPs [43]. Inlay-/onlay-retained FDPs are made by combining the cavities of the abutments by continuous unidirectional fibers. FRC framework on the flat bottom of the cavity supports the FDP against vertical occlusal loads. The FDP can be made indirectly or directly, and this type of FDP is preferred in the premolar region and the molar region. In premolar and especially in molar region, the requirement for the FRC FDP is adequate vertical space for connectors and inlays. In the connectors, 4 mm of vertical space is needed, and in the inlays (onlays, crowns) minimum of 2 mmof occlusal space is required for the FRC and overlaying veneering resin composite. Full-coverage crown-retained FDPs are made by layering woven FRC on prepared abutments. Abutments are connected with continuous unidirectional fibers and by having an additional piece FRC to support cusps of the pontics. Veneering is made with particulate filler resin composite. The use of full-coverage crowns as retaining elements of FDPs does not allow treatment to be according to the principles of minimal invasiveness. FRCs can also be used as reinforcements of provisional FDPs during fabrication of conventional FDPs [88].
5.5 Into the Root Canal
Root canal anchoring systems are in the same category of medical device regulatory requirements than FDPs. The first FRC root canal posts were used in Japan in 1600 century. The posts of that time were made of wood, which is a composite of cellulose fibers and lignin polymer matrix. The Father of Dentistry, Pierre Fauchard, presented silver posts for retaining crowns in the 1800s. Silver was thereafter replaced by dental gold alloys, which became standard for over hundred years of time. Metal posts are structural and due to material properties rigid in constructions, which effectively transfer occlusal loads to the fragile dentine of the root. Repeated stresses cause fatigue of dentine and can cause vertical fracture of the root. By adding so-called extraradicular metal ferrule of width of 1.5–2.0 to the crown, the root fractures can to large extent be eliminated. However, the present nonmetallic crown materials of glass ceramics and resin composites do not have metal ferrule, and thus, the root fracture elimination has to be done intraradicularly. The so-called modulus compensation is a method to lower the magnitude of local stress in root dentin. The modulus compensation is achieved by selection post material and post design, which matches to the modulus of elasticity of root. Glass FRCs fulfill the requirement of isoelasticity with dentine. The use of FRC in root canal posts to anchor cores and crowns has rapidly increased [63–73]. FRC can be used in root canal as prefabricated solid posts and individually formed posts, the latter representing to the most optimal post design [70].
The prefabricated posts are made of reinforcing fibers (carbon/graphite, glass, quartz) and finally polymerized resin matrix between the fibers which form a solid post of a predetermined diameter. Individually formed posts are made of non-polymerized fiber-resin prepregs, consisting of glass fibers and light-curing resin matrix. The rationale of the individually formed FRC post is to fill the entire space of the root canal by FRC material [70, 73]. The increased fiber quantity, especially in the coronal part of the root canal, increases load-bearing capacity of the system. Biomechanical behavior of restored tooth can also better be simulated because the fibers are located closer to the dentine walls, where the highest stresses exist. A tooth restored with individually formed root canal post system withstands cyclic loading of high magnitude for a long period of time without catastrophic failure or marginal breakdown of the crown, which can predispose to the secondary caries. For transferring the occlusal loads from crown to the individually formed FRC post, dentine, and periodontium, good bonding between the luting cements, post, and dentine is essential. Adequate bonding of resin composite luting cements to the post can clinically be achieved by using FRC post system where the polymer matrix is composed of interpenetrating polymer network (IPN) system which allows monomers of the cement to dissolve the surface of the post [63, 89–92]. Cross-linked polymer matrix of all present prefabricated FRC posts does not enable bonding of luting cements or core built-up resin composites to the post, and therefore mechanical retention of posts may be used.
5.6 To Replace Amalgam

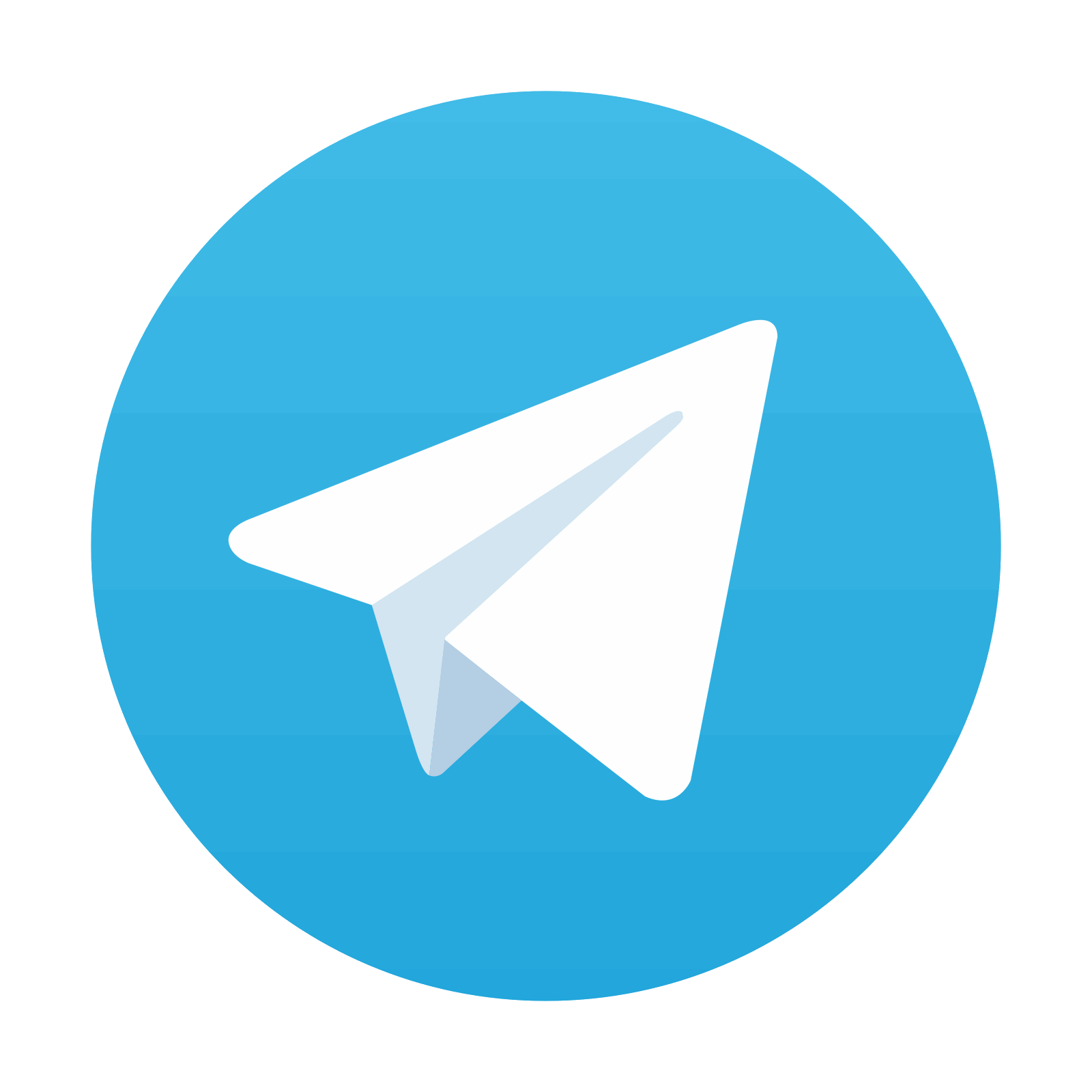
Stay updated, free dental videos. Join our Telegram channel

VIDEdental - Online dental courses
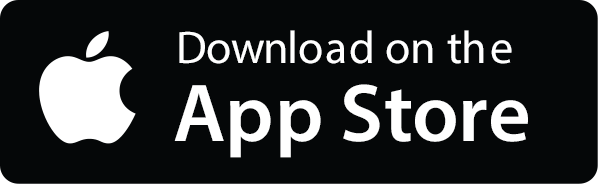
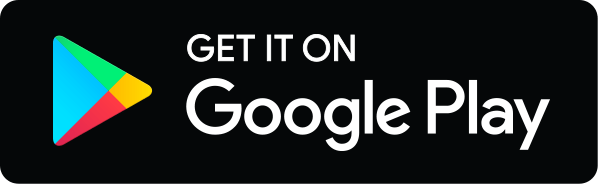