This review highlights ceramic material options and their use. The newer high-strength ceramics in monolithic form have gained popularity despite the lack of long-term clinical data to support this paradigm shift. Although there are some encouraging clinical data available, there is a need to develop laboratory simulation models that can help predict long-term clinical performance for ceramic and adhesive cements.
Key points
- •
Ceramic systems have varying degrees of research to support their use.
- •
Adhesive cements are changing based on knowledge gained from older materials. New cements are designed to accomplish durable bonding with fewer steps.
- •
The relationship between the cement used and crown survival is not well understood.
Introduction
Improvement in the optical and mechanical properties of dental ceramic materials coupled with improved production techniques have largely caused a shift in the type of fixed restorations dentists are prescribing. The complete crown procedure remains the most common fixed prosthodontic procedure prescribed by dentists. It has been estimated that in 2012 about 54.5 million indirect restorations were placed in the United States. Most of those units were most likely single crowns. In a recent survey of 1777 dentists enrolled in the National Practice-Based Research Network, it was estimated that for single-unit crowns, the top 3 choices for anterior crowns were lithium disilicate (54%), layered zirconia (17%), and leucite-reinforced glass-ceramics (13%). Less than 10% prescribed the more traditional metal-ceramic restorations. For posterior teeth, the top 3 choices for crowns were full contour zirconia (32%), porcelain fused to metal (31%), and lithium disilicate (21%). The profession has made a dramatic shift toward the increased use of all-ceramic materials for crowns.
This review focuses on the ceramic materials most often prescribed and some newly developed glass-ceramic materials. There are many ceramic systems that will not be discussed, and more complete reviews on all-ceramic materials can be found elsewhere. With respect to cements, this review focuses on the adhesive cements that are used in conjunction with dental ceramic crowns. Finally, this review discusses crown fracture with respect to the ceramic/cement interface. For the purposes of discussion, the ceramics are characterized into 2 categories: silica-based ceramics and non–silica-based ceramics; this categorization method is related to the types of cementation techniques suggested.
All-ceramic materials
The silica-based ceramics are the oldest of the two categories, with the introduction of the feldspathic porcelain jacket crown in the early 1900s having evolved into the much stronger glass-ceramic materials in routine use today. The fluormica-based glass-ceramic (DICOR) introduced in 1984 was the first dental application of an all–glass-ceramic material for crown fabrication. The lithium disilicate (LDS) glass-ceramics have nearly a 20-year record in service with good clinical data to support their use. The new zirconia-containing lithium silicate (LS) glass-ceramics have been developed to compete with the very popular LDS glass-ceramics. InCeram (Vita), the first non–silica-based dental ceramic, was introduced into dentistry in 1990. The dense sintered zirconia polycrystalline ceramics have been the dominant ceramics in this category for some time and represent the product that is experiencing the most rapid developmental changes ( Table 1 ).
Silica-Based Ceramics | ||
---|---|---|
Lithium disilicate glass-ceramics | Zirconia lithium silicate glass-ceramics | Other silica-based ceramics |
IPS e.max Press (Ivoclar/Vivadent) | Obsidian (Glidewell Laboratory) | IPS Empress Esthetic (Ivoclar/Vivadent) |
IPS e.max CAD (Ivoclar/Vivadent) | Celtra Press (Dentsply Sirona) | IPS Empress CAD (Ivoclar/Vivadent) |
Celtra Duo (Dentsply Sirona) | VitaBlocs Mark II (Vita Zahnfabrik) | |
Suprinity (Vita Zahnfabrik) | ||
Non–Silica-Based Ceramics (Y-TZP) | ||
Original zirconia formula | High-translucency zirconia | Ultrahigh-translucency zirconia |
Lava Frame (3M ESPE) | Lava Plus (3M ESPE) | Lava Esthetic (3M ESPE) |
Zenostar MO (Ivoclar/Vivadent) | Zenostar T (Ivoclar/Vivadent) | Zenostar MT (Ivoclar/Vivadent) |
Cercon base (Dentsply Sirona) | Cercon ht (Dentsply Sirona) | Cercon xt (Dentsply Sirona) |
Prettau Zirconia (Zirconzahn) | Prettau Anterior (Zirconzahn) | |
Bruxir (Glidwell Laboratory) | Bruxir Anterior (Glidwell Laboratory) | |
Katana HT/ML (Kuraray/Noritake) | Katana UTML/STML (Kuraray/Noritake) |
Silica-Based Dental Ceramics
Lithium disilicate glass-ceramics
LDS glass-ceramic, the first glass-ceramic developed, provided knowledge from fundamental studies using this system, which formed the basis for further development of many different glass-ceramic materials. LDS glass-ceramic was introduced for crown application in 1998 by Ivoclar Vivadent. The material was marketed first as Empress 2 and later as eMax Press and eMax CAD. It is the most well documented and successful long-term all-ceramic crown material currently in use. Over time it has become a major restorative choice for single anterior crowns and to a lesser extent for posterior crowns.
A more complex multicomponent LDS system with increased translucency, easy machinability, and decreased reactivity with the refractory investment were all modifications needed to accommodate esthetic crown fabrication. LDS glass-ceramics required certain compositional and processing modifications to result in the strong (400 MPa), tough (2.5 MPa m 1/2 ), and translucent (55%) structure that is used today.
Detailed analysis of the thermal process for this particular glass-ceramic formulation has shown that there are actually 2 major crystal phases that begin to form at about 500°C. The lithium metasilicate (LMS) Li 2 SiO 3 crystals dominate at the lower temperatures with only minor amounts of the LDS (Li 2 Si2O 5 ) crystallites being present initially. At temperatures higher than 750°C, a secondary crystallization process occurs whereby the LMS crystals react with the surrounding glass to form additional LDS crystals with the aid of an intermediate lithium orthophosphate Li 3 PO 4 (LOP) nucleating agent. Under very specific heating conditions, the LMS crystals can be fully dissolved and the final product is composed entirely of LDS crystals (70 vol%) and a small amount of residual glass. It is less well known that LDS glass-ceramics contain a small amount of ZrO 2 (3%–4%), which has been shown to help increase translucency.
The LDS is supplied in 2 different crystalline states depending on the application. The CAD form (eMax CAD) is supplied in a precrystallized state that is more easily machined. The CAD form is primarily glassy with evidence of the presence of LMS and LOP crystals, which gives the e.max CAD milling blanks their characteristic “blue block” appearance. The milled restoration is subsequently heat treated to allow the crystallization process to continue to completion. In the final form the glass-ceramic contains primarily LDS crystals. The pressable pellets (e.max Press) supplied by the manufacturer are in the fully crystallized state. The influence of these differences on clinical performance is unknown.
Several clinical trials and meta-analysis of its performance as single crowns (SCs) has been reported. The cumulative survival meta-analysis of LDS SCs reported a 5-year survival rate of 97.8%. In another meta-analysis, which combined studies that included both LDS and leucite-reinforced glass-ceramics, the 5-year survival rate was 95.6% for SCs. The 8-year cumulative survival rates for Empress 2 were reported to be 94.8% for SCs. Most recently the 10-year cumulative survival rates of Empress 2 restorations were reported to be 81% for SCs and 93.4% for implant-supported crowns. A 10-year evaluation of molar crowns manufactured from e.max CAD materials was reported to be 83.5%.
Lithium silicate glass-ceramics
LS glass-ceramics are the newest glass-ceramic materials designed for crown applications. They were introduced in 2013 as zirconia-reinforced lithium silicate (ZLS) glass-ceramics, a stronger alternative to the LDS material. Commercially this material is marketed as Celtra (Dentsply Sirona) and Suprinity PC (Vita). The materials are similar in composition, as they were developed in a collaborative effort between the 2 manufacturers and the Fraunhofer Institute for Silicate Research in Wurzburg, Germany. Glidwell Dental laboratories, has introduced its own version of this material that is marketed under the name of Obsidian.
The microstructure has been examined by several investigators. In the fully crystallized form, these materials consist primarily of LMS crystals with minor amounts of LDS and LOP. The low concentration of tetragonal zirconia (10%) is not detectable by Raman spectroscopy and only slightly detectable by x-ray diffraction spectroscopy. Because the ZrO 2 crystals remain in the residual glass they are marketed as ZLS glass-ceramics. Whether they actually contribute to the overall strength of the final glass-ceramic product is unclear.
In the CAD form, the glass-ceramic is supplied in either a precrystallized or fully crystallized form. The precrystallized form is easier to machine because it is primarily a glassy structure with a small amount of very fine precipitated crystals of LMS and LOP. The machined precrystallized crown is subsequently heat treated to produce the fully crystallized form of the glass-ceramic. In the fully crystallized form they have strengths comparable with or greater than e.max CAD. These ceramics have demonstrated easier polishability and slightly more translucency than e.max CAD. There is little reported on the pressable form of this new glass-ceramic, but it would be expected to be similar. The manufacturer’s information (Celtra Press FactFile) shows the pressing pellets to contain very small plate-like crystals initially and larger more needle-like crystals after pressing, indicating that some crystal growth occurs during the pressing cycle. The unique characteristic of Obsidian is that this LS glass-ceramic composition containing 1% to 10% germanium, which claims to make the material more castable by lowering its viscosity and increasing the thermal expansion and the refractive index of the glass. This allows the material to be pressed to metal. No independent laboratory or clinical data are available for this material, but it is expected to be similar to the other products in this category.
Only one clinical evaluation of Celtra Duo CAD/CAM LS restorations could be found in the literature. The success rate after 1 year was reported to be 96.7% with 2 failures out of 60 restorations being reported from bulk fractures. Further long-term clinical results are needed to fully understand the clinical performance of these materials.
Non–Silica-Based Dental Ceramics
Polycrystalline zirconia
Of the non–silica-based ceramics in use today, dense sintered polycrystalline zirconia is the most commonly prescribed all-ceramic crown material. Its rapid increasing rate of application is attributed to its high strength, high toughness, reasonable esthetics, and claims by manufacturers and laboratories to be strong enough for use on posterior teeth even with a relatively conservative preparation.
The first commercial application of a dense sintered polycrystalline core material was Procera (Nobel Biocare). The Procera process was significant because it was the first commercial application of CAD/CAM manufacturing used within the laboratory industry. In the initial introduction, 99.9% alumina was used as the core material. The initial alumina coping materials generated strengths in the area of 550 MPa. Later, yttria-stabilized tetragonal zirconia oxide (Y-TZP) copings became available with much higher strength (>1200 MPa) and toughness (4.4 MPa m 1/2 ). The high cost of CAM equipment led to a business model, which had local laboratories scan the die with a digital scanner, design the coping, and send the design file via the Internet to one of two worldwide manufacturing sites. The coping was returned to the laboratory within 3 days. The local laboratory applied the porcelain veneer to the coping, completing the crown within the same timeframe as a metal-ceramic crown. The concept soon led other manufacturers to follow (ie, LAVA, 3M-ESPE, CERCON: Densply).
Unlike pure aluminum oxide (Al 2 O 3 ) powders, pure zirconium oxide (ZrO 2 ) powders are not able to be formed into a dense solid structure directly. The stresses generated on cooling from the smaller tetragonal crystals transforming to larger monoclinic crystals create stresses and cracking. Strengthened zirconia was first developed in the 1970s and details of the toughening mechanism have been described. Since the initial introduction of this material to dentistry, it has undergone several formulation changes and these have been summarized in some detail.
The zirconia products originally introduced in dentistry were 3 mol% yttria-stabilized tetragonal polycrystal (3Y-TZP). This material had the characteristics of high strength (>1100 MPa), high toughness (>4 GPa), high stiffness (>200 GPa), and high opacity. The high opacity limited their application to crown substructures. These original formulations are still applicable for layered crowns and very demanding structural applications such as implant-supported complete prostheses and long-span tooth-supported fixed dental prostheses. Much of the clinical longevity data that have been reported are from these layered crowns. Layered zirconia was demonstrated to have higher chipping rates than metal-ceramics. Several factors were thought to contribute to this clinical observation, and current chipping rates have been reduced.
The opaque nature of zirconia arises because of the birefringence character of the tetragonal crystals, the size of the crystals, and issues related to light scattering at the grain boundaries, which contain pores and impurities. Changes in formulations for the newer zirconia materials have been discussed. The first improvements were designed to modify the grain size and the grain boundaries through improved heat treatments and minor dopant composition changes. The translucency changes were modest and had little effect on the mechanical properties, making them suitable for monolithic posterior crowns. Many clinicians were introduced to this application through an online video displaying a monolithic zirconia crown being hammered into a piece of wood with no damage to the crown. Although this test has no relationship to clinical performance, the visual did have an impact on encouraging dentists to try these ceramics for posterior crowns. In addition, it was purported that reduced preparation dimensions and survival rates were similar to those of cast metal, and laboratories were supporting the claims with a 5- to 7-year manufacturer material warranty. The clinical performances of conservative monolithic crowns are beginning to appear in the literature. That there are few anecdotal or scientific reports of unusual problems with millions of units already in service is encouraging.
The ultrahigh-translucency zirconia is the latest generation of newly formulated compositions. These materials have increased their translucency by increasing the yttria dopant from 3 mol% to as high as 5 mol%. The higher yttria content stabilizes more cubic zirconia into the final polycrystalline structure. The cubic phase does not have the high scattering effect that the tetragonal stabilized crystals do, leading to higher translucencies. However, these materials do have a somewhat diminished fracture toughness (2.5 GPa) and strength (700 MPa).
Much has been written concerning the potential susceptibility of Y-TZP to low-temperature degradation (LTD), and this process has been reviewed in detail. The issue first arose from work done to understand a problem related to the premature failure of the Y-TZP ceramic heads on hip implants. The basic mechanism that gives this ceramic its unusual toughness and strength characteristics also exposes a potential Achilles heel. The crystal transformation induced by stresses can slow crack propagation, but the same process also can occur spontaneously by reaction with water, potentially leading to microcracking and strength loss. This reaction is autocatalytic, but the kinetics of the degradation process are greatly accelerated by temperature. Researchers study the process at elevated temperatures of 137°C to accelerate the effect. It was estimated that 1 h under these conditions is equivalent to 4 years of service in vivo. Numerous laboratory studies have reported on the effects of accelerated LTD on zirconia properties. The depth to which the LTD process penetrates the surface depends on the specific materials and amount of time held at 137°C, but is usually confined to the surface 5 to 10 μm. Even under the most extreme aging conditions, strength and toughness are either unaffected or only modestly decreased. Significant decreases in strength were noted only after more than 100 h of accelerated aging, comparable with 300 years. Keuper and colleagues have examined the LTD process at 37°C and have estimated the rate of progression to be 0.5 μm/y (1 grain/y). Although the process will progress over time, based on these findings the strength levels would not be affected for tens of years. There is clearly a need to understand this process under more clinically relevant conditions.
Adhesive cements for all-ceramic restorations
Adhesive cements are broadly categorized into 2 groups: water-based cements or resin-based cements ( Table 2 ). The current range of cements actually exists as a spectrum of materials that share characteristics of each group. Cements that require the application of a dental adhesive before cementation are considered to be more “composite-like” while those requiring no adhesive before cementation are considered more “glass-ionomer–like.” New developments in cements and adhesives have resulted from improved the understanding of the chemical and structural features of the interface that exists between dentin and adhesive materials. The use of visual and chemical analytical methods including transmission electron microscopy, field-emission scanning electron microscopy, x-ray photoelectron spectroscopy, infrared spectroscopy, and advanced sample preparation methods have advanced this knowledge, leading to the introduction of the newest class of cements and adhesives that are considered to be self-etching and self-adhesive.
Water-Based Adhesive Cements | ||
---|---|---|
Glass-Ionomer Cement | Resin-Modified GI Cements | Other Water-Based Cements |
Ketac Cem (3M ESPE) | Rely X Luting (3M ESPE) | Zinc phosphate (Fleck’s-Mizzy) |
Fuji 1 Cement (GC America) | Fuji Cem 2 (GC) | Zinc polycarboxylate (Durelon-3M) |
VivaGlas Cem PL (Ivoclar) | Rivia Luting Plus (SDI) | |
Hy-Bond CX plus (Shofu) | Nexus RMGI (Kerr) |
Resin-Based Cements | ||
---|---|---|
Self-Adhesive Resin Cements (DC) | Adhesive-Assisted Resin Cements | |
a Rely X Unicem (3M ESPE) | DC Cements | SC-Only or LC-Only Cements b |
a Panavia SA Cement Plus (Kuraray) | a Panavia F 2.0 with a SEA (Kuraray) | a Panavia 21 SC with a SEA (Kuraray) |
a Maxcem Elite (Kerr) | Panavia V5 with a SEA (Kuraray) | |
a SmartCem2 (Dentsply) | a Rely X Ultimate with a SEA or a ERA (3M) | b NX3 Nexus LC with a SEA or ERA (Kerr) |
a SpeedCem (Ivoclar) | VariolinkEsthetics DC with a SEA or a ERA (Ivoclar) | b Variolink Esthetics LC with a ERA or a SEA (Ivolclar) |
BisCem (Bisco) | Multilink with a SEA (Ivoclar) | b Rely X Veneer Cement LC with a ERA (3M) |
NX3 Nexus DC with a SEA or ERA (Kerr) | ||
NX3 XTR with a SEA (Kerr) |
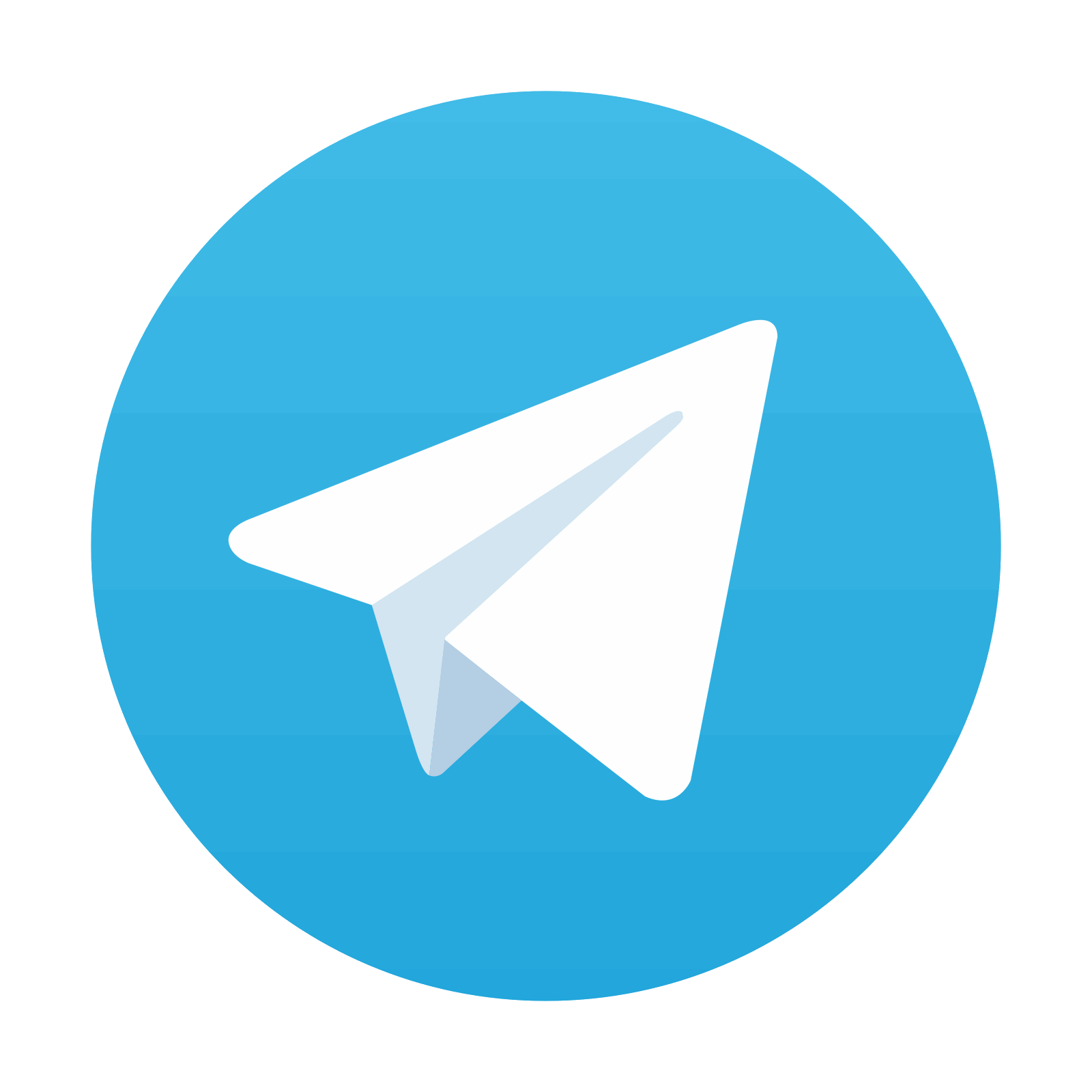
Stay updated, free dental videos. Join our Telegram channel

VIDEdental - Online dental courses
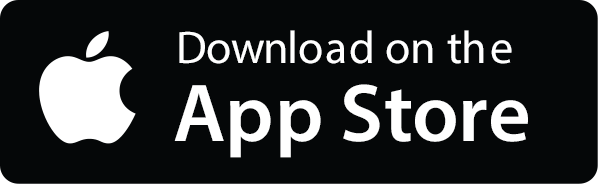
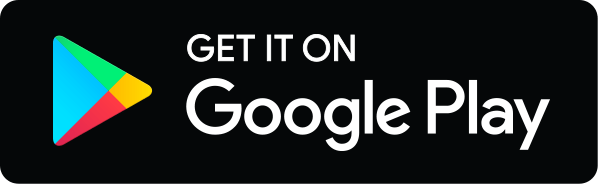
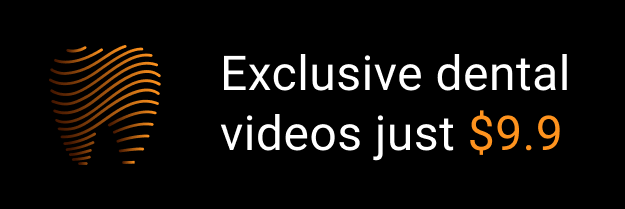