2
Biological Basis for Vital Pulp Treatment
Fionnuala T. Lundy1, Lara T. Friedlander2, and Paul R. Cooper2
1 The Wellcome‐Wolfson Institute for Experimental Medicine, School of Medicine, Dentistry and Biomedical Sciences, Queen’s University Belfast, Belfast, Northern Ireland, UK
2 Faculty of Dentistry, Sir John Walsh Research Institute, University of Otago, Dunedin, New Zealand
Dental Pulp – Structure and Function
The dental pulp is a unique connective tissue containing cells and extracellular matrix (ECM), along with an extensive neuronal and vascular plexus, encased in a rigid dentine structure. The development of the dental pulp is outlined briefly below, along with the roles of the major cellular and structural components.
Development and Anatomy of the Dental Pulp
In embryological terms, the process of tooth development begins at around the fifth embryonic week (crown development) and continues after birth (root development). The initial stages of tooth development in the embryo commence as a result of a series of reciprocal interactions between the odontogenic epithelium and the neural crest‐derived ectomesenchyme of the developing jaws (1, 2). These interactions play a fundamental role in tooth morphogenesis and regulate all aspects of tooth development including tooth morphogenesis, and the number, shape and spatial patterning of the teeth. Key signalling molecules act reiteratively during these processes to mediate crosstalk, including wingless‐related integration site (Wnt), bone morphogenic protein (BMP), fibroblast growth factor (FGF), sonic hedgehog (Shh) and Ectodysplasin (Eda) (3).
Tooth development is a continuous process and therefore few completely distinct stages can be delineated. However, a key event for the initiation of tooth development is the formation of localized U‐shaped thickenings, known as dental placodes (sixth embryonic week) within the primary epithelial bands, at the sites of the future dental arches of the embryonic mandible and maxilla. The first evidence of embryonic teeth is evident when the epithelial cells near the basement membrane multiply and invaginate into the underlying ectomesenchyme to give rise to the dental lamina in 10 areas of the upper arch and 10 areas of the lower arch. Each dental lamina is the origin of the tooth bud that will give rise to a tooth in the mandible and maxilla. Deciduous incisor and canine tooth buds are evident at the eighth embryonic week, and deciduous molar tooth buds are evident at the ninth week. In the bud stage, the ectodermal basement membrane progressively invaginates into the underlying ectomesenchyme, in which the cells are closely packed around the epithelial bud. Transformation of the epithelial bud into a concave or cap‐shaped structure initiates the establishment of the coronal architecture of the tooth. The cap, which consists of an epithelial outgrowth, is often called the enamel organ because it will later form the cells that produce enamel. The remainder of the tooth bud, known as the dental papilla, will give rise to the dentine and the pulp. An additional sac of cells, known as the dental follicle, surrounds the enamel organ and the dental papilla. The dental follicle contains the cells that give rise to the pulpal blood vessels and nerves. By the end of the cap stage, three different structures are apparent: the enamel organ, the dental papilla and the dental follicle. Within the enamel organ is the enamel knot, a transient structure that controls the morphogenesis of the dental cusps and determines the shape of the tooth at the bell stage. Two additional regions of the enamel organ are also apparent, the stratum intermedium and the stellate reticulum, both involved in facilitating enamel production.
A relatively large number of development changes occur during the transition from the cap stage to the bell stage and the cells of the enamel organ differentiate to perform specific functions. By this stage the enamel organ contains two layers of cuboidal cells named the inner and outer enamel epithelium. During the later bell stages, tooth morphogenesis is followed by a period of histodifferentiation, involving interactions between the cells of the inner enamel epithelium, which differentiate into pre‐ameloblasts and those of the epithelial‐mesenchyme, which differentiate into pre‐odontoblasts. The pre‐ameloblasts and pre‐odontoblasts ultimately polarize and secrete enamel and dentine, respectively. The outer enamel epithelium differentiates to form the junctional epithelium between the tooth and the gingival tissue. The dental papilla cells differentiate into periodontal cells to form the cementum‐periodontium‐like tissues.
In the mature tooth, the dental pulp occupies the pulp chamber, pulp horns and radicular canals. Odontoblasts are subjacent to the dentine and are capable of detecting sensory stimuli (4). Sensory nerves also innervate the dental pulp, including projecting into the odontoblast layer and extending into the dentinal tubules. The majority of the pulp contains fibroblasts and the ECM, along with smaller numbers of stem cells and immune cells. The cells of the dental pulp are discussed below in greater detail, along with the ECM, pulpal innervation and vasculature.
Cells of the Dental Pulp
The dental pulp extends from a central chamber within the crown of the tooth into one or more root canals (depending on the type of tooth). The pulp is encased by dentine, and the relationship between the soft tissue of the dental pulp and the hard dentinal tissue has often been emphasized using the phrase the ‘dentine‐pulp complex’.
Odontoblasts
Located subjacent to the dentine, specialized cells known as odontoblasts line the pulp chamber and root canals. Odontoblasts are columnar, post‐mitotic, polarized cells that are responsible for secreting a collagenous matrix, which mineralizes to form dentine. Dentine formation (dentinogenesis) occurs during tooth development, forming primary dentine at a rate of approximately 4 μm/day. Throughout their life span, quiescent odontoblasts synthesis secondary dentine, similar in structure and continuous with the primary dentine, at a much slower rate of approximately 0.4 μm/day. Secondary dentine is formed after the tooth has erupted and the root is fully formed. Tertiary dentine is produced in response to a carious lesion, injury or noxious reaction to dental materials. If the damage is mild, then the native odontoblast cells will synthesize tertiary dentine, in this case often referred to as reactionary dentine. However, more extensive damage will lead to the death of the odontoblasts in the local area of the lesion/injury, and in this case, the dentine is synthesized by odontoblast‐like cells (5, 6). This type of tertiary dentine, formed by odontoblast‐like cells, is often referred to as reparative dentine. The regulation and processes involved in dentinogenesis are discussed in greater detail below.
With their anatomical location in the outermost layer of the dental pulp, odontoblasts provide a natural barrier between mineralized dentine and the soft tissue of the dental pulp. Each odontoblast has a cellular process embedded in the mineralized dentinal tissue, giving dentine its tubular structure. The cell body of the odontoblast is located within the soft tissue of the pulp and is not encased in dentine (7). Evidence for the role of odontoblasts in forming the first line of defence in the dental pulp comes from their ability to detect a wide variety of pathogen‐associated molecular pattern molecules (PAMPs), via the expression of pattern recognition receptors (PRRs) such as Toll‐like receptors (TLRs) and nucleotide‐oligomerization binding domain (NOD) receptors (8, 9). In addition to this defensive role, odontoblasts sense the external environment and can recognize thermal, mechanical and chemical stimuli via the expression of a variety of channels and receptors, including the transient receptor potential (TRP) channels (4, 10, 11) and piezo channels (12). Although sensory nerve fibres are known to innervate the odontoblast layer and dentinal tubules (13), the exact mechanism(s) of signal transduction from odontoblasts to sensory nerves remains to be fully elucidated. Some evidence indicates that ATP may play a role in this signal transduction (14); however, further studies are required to improve our understanding.
Fibroblasts
Dental pulp fibroblasts are the most abundant cells in the dental pulp and are responsible for synthesizing ECM proteins, such as collagen and fibronectin, as well as enzymes, including matrix metalloproteinases (MMPs), which are responsible for ECM turnover (15, 16). Pulp fibroblasts may also contribute to healing and inducing angiogenesis following injury by the expression of growth factors (GFs) such as vascular endothelial growth factor (VEGF) and FGF (17).
In addition to their well‐recognized role in regulating the synthesis and turnover of the ECM, fibroblasts have defensive properties by virtue of their synthesis of complement system proteins (18). Fibroblasts also synthesize proinflammatory cytokines (19) and chemokines (20), and they express PRRs, suggesting their involvement in pathogen detection and the subsequent immune response (21). The synthesis of multiple GFs and cytokines by dental pulp fibroblasts also has a role in inducing the migration (22) and differentiation (23) of dental pulp stem cells (DPSCs) into odontoblast‐like cells, which synthesize tertiary dentine in response to caries ingress or physical trauma.
The expression of some neuropeptides (24) and their receptors (25), provides a link between fibroblasts and the pulpal neurogenic inflammatory response (26). Indeed, neuropeptides have been shown to regulate the expression of angiogenic factors by pulp fibroblasts (27), providing further evidence for the wide range of functional proteins synthesized by pulp fibroblasts. The expression of the TRP channels by dental pulp fibroblasts (28) indicates they are able to sense the external milieu.
Stem Cells
Postnatal DPSCs were first identified in the dental pulp over 20 years ago and were shown to possess self‐renewal capacity, multilineage differentiation capacity and clonogenic efficiency, with the ability to regenerate dentine‐pulp‐like complexes in vivo (29, 30). DPSCs are derived from the neural crest during development (31) and have the capacity to secrete neurotrophic factors (32) and differentiate into functional nerves (33, 34). Using genetic lineage tracing, Feng et al. described two locations for stem cells in the dental pulp; the perivascular region (pericytes) and peripheral nerve‐associated glial cells (35). In the healthy dental pulp, stem cells are generally maintained in a quiescent state. As outlined above, odontoblasts will respond to injury or infection by synthesizing dentine to protect the underlying pulp tissue. However, if injury or infection is sufficient to cause the death of the odontoblasts in the local area, events may trigger the proliferation of resident stem cells to differentiate into odontoblast‐like cells capable of tertiary, reactionary dentine secretion (35, 36). The cellular and molecular processes involved in this are described in greater detail below. Furthermore, DPSCs have also been reported to exhibit both immunomodulatory and antimicrobial functions. When cocultured with activated T cells, DPSCs were shown to decrease levels of proinflammatory cytokines, as well as increase levels of anti‐inflammatory cytokines (37). Recently, the cytokines and GFs present in the secretome of DPSCs, including hepatocyte GF, angiopoietin‐1, interleukin (IL)‐6 and IL‐8, were shown to have direct antibacterial activity against oral pathogens (38), indicating a novel role for stem cells in fighting infection.
Immune Cells
The dental pulp is served by a variety of resident and recruited immune cells. Following injury or ingress of caries, odontoblasts and sentinel immune cells within the pulp release cytokines and chemokines (39–41), some of which are involved in the recruitment and activation of additional immune cells from the bloodstream to the site of pulpal infection and inflammation (42). These immune cells aim to destroy and engulf the invading microorganisms, using a complex arsenal of antimicrobial peptides (AMPs) (43). A variety of immune cells, including monocytes, neutrophils, T cells and dendritic cells (DCs), are involved in the pulp’s immune response and may become activated once bacterial antigens are captured following their diffusion through dentinal tubules to the pulp tissue (40, 44). The complex cellular and molecular processes involved in the pulp’s immune response are described subsequently.
ECM
The cells of the dental pulp are located in a loose connective tissue, comprised mainly of collagen types I and III (45, 46), with much smaller amounts of type V collagen (47). Collagen fibrils are associated with non‐collagenous proteins that play roles in either stabilizing the fibril network or participating as inhibitors or activators in the mineralization process. Fibronectin is the principal non‐collagenous protein in the dental pulp, showing abundance within the odontoblast layer, around blood vessels and in the core of the dental pulp (46, 48). Proteoglycans, which are glycosylated proteins with covalently attached highly anionic glycosaminoglycans, are present in the dental pulp, as is the case in almost all connective tissue ECMs (46). Small leucine‐rich proteoglycans are associated with collagen fibrils in dentine, whereas glycosaminoglycans contribute to collagen fibril structure. The family of non‐collagenous proteins termed small integrin‐binding ligand N‐linked glycoproteins (SIBLINGS) play roles in regulating dentine formation and include; dentine sialoprotein (DSP), dentine phosphoprotein (DPP), dentine matrix protein‐1 (DMP‐1), bone sialoprotein (BSP), matrix extracellular phosphorglycoprotien (MEPE) and osteopontin (OPN) (49–51). Moreover, a complex array of GFs, cytokines, MMPs and neuropeptides are also detectable in the ECM and are implicated in pulpal homeostasis.
Nerves
The dental pulp is an extensively innervated tissue (52, 53). The majority of pulpal nerves are sensory trigeminal afferents that detect noxious stimuli in the dental pulp tissue and transmit signals to the central nervous system, which is perceived as pain. Sensory nerves innervate the entire dental pulp, including the odontoblast layer. Indeed, ultrastructural analysis suggests that sensory nerves also extend into the dentinal tubules in the inner portion of the dentine (13). Sensory nerve fibres are generally classified on the basis of their diameters and conduction velocities. ‘A fibres’ are myelinated nerves with large diameters and high conduction velocities, whereas ‘C‐fibres’ are unmyelinated with smaller diameters and lower conduction velocities. The afferent sensory nerves that innervate the dental pulp include both A fibres and C‐fibres, with approximately 87% of pulpal sensory nerves classed as unmyelinated C‐fibres (54, 55), and the remaining 13% are classified as myelinated A fibres (93% Aδ and 7% Aβ). Interestingly, although nerves entering the tooth through the apical foramen tend to be myelinated, many of them lose their myelin sheath on entering the dental pulp, or as they course and branch through the radicular and coronal pulp tissues (56, 57).
Upon stimulation, sensory nerve fibres not only transmit pain to the central nervous system but also release neuropeptides, such as substance P (SP), neurokinin A and calcitonin gene‐related peptide (CGRP) in the pulp tissue. Consequently, they contribute to the local neurogenic inflammatory response (26, 58). The remainder of the pulpal nerves are autonomic efferents that transmit signals from the central nervous system to the periphery. The autonomic (sympathetic and parasympathetic) nerves have been studied less comprehensively, but evidence suggests that the sympathetic nerves contribute to pulpal blood flow (59), and they may have sensory roles when the dental pulp is inflamed (60). There is also evidence that sympathetic nerves contribute to the recruitment and migration of inflammatory cells (61). Although there is no consensus regarding parasympathetic innervation of the dental pulp, it is worth noting that vasoactive intestinal polypeptide (VIP), considered to be a marker for parasympathetic nerves, has been demonstrated in the dental pulp (59, 62–64).
Vasculature
The dental pulp has a rich vascularity; however, in contrast to most other tissues, it lacks a collateral supply, meaning it is also vulnerable. A dense network of blood vessels perfuse the dental pulp to provide nutrition (65). Arterioles enter the apical foramen and ascend the central region of the pulp proper, branching to form a dense capillary network to bring oxygenated blood to the peripheral pulp tissue. A network of venules returns deoxygenated blood, via the apical foramen, to the circulation (66). During the pulpal inflammatory response to injury and infection, vasodilation of blood vessels and capillary proliferation will occur (67). Blood vessels in the dental pulp are in close association with nerves and pulpal blood flow is considered to be under neural local control (60). Recent evidence shows that the dental pulp lacks lymphatic vessels (68). Instead, the drainage of fluid within the pulp is likely to be governed by a tissue pressure gradient (driving pressure) and is transported in loose connective tissue gaps surrounding vessels and nerve fibres (68).
Ageing
Ageing reduces the dimensions and volume of the pulp chamber due to the accumulation of secondary and tertiary dentine. Deposition of dental cementum with increasing age narrows the apical foramen and decreases perfusion of the tissue and the extent of innervation. There is also some atrophy of the dental pulp tissue; a decline in the total number of pulp cells has been reported with ageing (69). Significant decreases in numbers of sub‐odontoblast cells with ageing have been observed in rodent and human pulp (70, 71), and a progressive decrease in fibroblasts is considered to be part of the pulp ageing process in human teeth (71). The ageing of the pulp tissue is associated with reduced vascularity and is proposed to reduce blood flow and the draining capacity of the tissue, making it more vulnerable to circulatory failure (68).
Healing Capability of the Dental Pulp
As indicated above, and similar to other tissues around the body, the dentine‐pulp complex has an innate ability to heal following disease or trauma. The response whereby new dental hard tissue is formed postnatally by the dental pulp tissue is termed tertiary dentinogenesis (Figure 2.1). This tertiary dentine provides a localized physical barrier beneath the site of injury and contributes to tissue integrity via repair and regenerative processes (72). Tertiary dentine can describe a range of hard tissue deposited including a regular tubular structure continuous with the developmentally derived primary and physiological secondary dentine, as well as a more dysplastic atubular matrix (73, 74). Importantly, the molecular and cellular processes responsible for the different tertiary dentine depositions vary significantly. Consequently, tertiary dentine is classified as being either reactionary or reparative (75), and this occurs in response to mild (e.g. slowly progressing carious lesions) or more severe (e.g. rapidly progressing carious lesions) external stimuli, respectively.

Figure 2.1 Schematic of the two types of tertiary dentine formation processes, reactionary and reparative dentinogenesis, formed in response to carious or traumatic lesions of differing intensities. Component tissues shown are A = Enamel; B = Dentine; C = Pulp.
Reactionary dentine is stimulated in response to mild irritation and deposited by surviving primary odontoblasts (75). In contrast, in more severe injury where primary odontoblasts are lost, reparative dentine is deposited following the differentiation of a new generation of odontoblast‐like cells. Interestingly, this local odontoblast death may occur in the absence of pulpal exposure but may be due to trauma or deep and rapidly progressing caries. A range of bioactive molecules are important regulators and stimuli for both of these dentinogenic processes (76). Comparatively, reactionary dentinogenesis requires a less complex process as it involves only the re‐stimulation of the existing primary odontoblasts to deposit dentine at a rate greater than that already observed during secondary dentinogenesis (77). However, reparative dentinogenesis involves the recruitment of progenitor dental pulp cells, which are subsequently signalled to differentiate into odontoblast‐like cells (78). The stimulated reparative dentine differs from primary and secondary dentine in terms of the quality of the dentine deposited. It generally lacks a tubular structure, is an amorphous mineralized tissue bridge and may contain so‐called ‘tunnel’ defects. The mineralized hard tissue deposited may be more similar to bone than it is to dentine (72, 74, 79, 80). Currently, there is significant debate as to the origin of the cells which deposit this mineralized bridge. Some researchers have proposed that this hard tissue occurs due to the activity of perivascular cells (81), fibroblasts (80) or fibrocytes (82) rather than cells that have been more traditionally termed odontoblast‐like cells (83, 84). Interestingly, if pulpal fibroblasts were to produce the mineralized tissue in the reparative response, there would still need to be cytodifferentiation as these cells do not naturally produce hard tissues in their basal state. However, some evidence does exist that supports this cellular process being induced under appropriate conditions (85–88).
Whilst reactionary and reparative dentinogenesis is generally considered as occurring separately due to the disease or tissue stimulatory events present, some researchers have proposed that in relatively deep and active carious lesions, both processes may occur simultaneously (83). Indeed, in a dynamic and rapidly advancing carious lesion, active parts of the lesion may contain reactionary dentine formation, whereas, in more advanced areas of the lesion, reparative dentinogenesis may be present (89).
Molecular and Cellular Regulatory Processes in Tertiary Dentinogenesis
Bioactive Molecules
During primary and secondary dentinogenesis a plethora of bioactive molecules, termed dentine matrix components (DMCs), are deposited and archived within the dentine as the tooth develops and during dental tissue homeostasis. These DMCs include many GFs, cytokines, chemokines, MMPs as well as other bioactive molecule subclasses (83). DMCs are known to modulate a range of cellular processes important in tissue repair, including chemotaxis (84, 90, 91), angiogenesis (92), mineralization (91), stem cell recruitment (93) and neurogenesis (94). In particular, well‐characterized GFs, which have been shown to be central to orchestrating and modulating dentine‐pulp complex repair and regeneration, include members of the transforming GF (TGF) superfamily (95, 96) and insulin‐like GFs (97, 98). Other GFs include angiogenesis‐promoting molecules, such as VEGF, fibroblast GF‐2 (FGF‐2), platelet‐derived GF (PDGF) and placenta GF (PlGF) (91, 99), as well as neurogenesis promoters including brain‐derived neurotrophic factor (BDNF) and growth/differentiation factor 15 (GDF‐15) (98).
As caries advance towards the pulp the dentine becomes progressively demineralized, and this results in the increased release of the archived DMCs (100). Interestingly, previous work has demonstrated that selected MMPs, including MMP‐2, ‐8 ‐9 (101), are contained within the DMCs and are able to hydrolytically break the exposed dentine organic matrix (102) to generate potentially more potent bioactive molecules (103) that diffuse down the dentinal tubules and enter the pulp. These molecules have an important role in modulating the caries process and are known to be potent stimulators of tertiary dentine formation and other associated pulpal reparative processes, including angiogenesis and neurogenesis (97, 104). The holistic promotion of dentine‐pulp complex repair is understood to be important due to the synergistic interaction of all component cells and tissue types (83).
The potential to therapeutically harness the bioactive DMCs has been a focus of considerable recent research activity (83). The use of several clinical reagents, treatments and chemicals, including ethylenediaminetetraacetic acid (EDTA) (90, 105), hydraulic calcium silicate cement (106), calcium hydroxide (105), dental adhesives (107) and ultrasonic agitation (108), to release DMCs have been explored. EDTA treatment of dentine promotes the migration and differentiation of DPSCs and remains the gold standard, with the TGF‐β family members being used as key biomarkers demonstrating the release of the bioactives from the dentine (90). In contrast, 6% sodium hypochlorite (NaOCl) treatment of dentine has demonstrated a deleterious effect, particularly on the survival, viability and differentiation capability of DPSCs (109). This has led to clinical guidelines that balance disinfection with cellular vitality and recommend that irrigation with 1.5–3% NaOCl, followed by a final rinse of 17% EDTA, should be used for vital pulp treatment procedures (109, 110).
Importantly, the bioactive molecules can act synergistically at nanomolar concentrations to regulate many cellular processes. To stimulate the reactionary dentinogenic response, the DMCs directly act on the surviving primary odontoblasts to upregulate their dentine secretory and synthetic activity. However, as reparative dentinogenesis is more complex, bioactive molecules also regulate the progenitor cell recruitment process, proliferation and differentiation steps. Consequently, multifactorial signalling provides the flexibility required for the tissue and is required to invoke repair responses (84).
Cellular Responses
Cellular studies of tertiary dentinogenic responses have mainly focused on the role of odontoblasts (111) or the mesenchymal SC populations (112). Regeneration involves the replacement of tissue damaged by trauma or disease with that which resembles the native tissue, and pluri‐ or multi‐potent stem cells under the regulation of growth and differentiation signals are required for this process. Several dental stem cell (SC) populations have been described including DPSCs, which comprise less than 5% of all pulp cells (30), as well as stem cells of the apical papilla (SCAP), stem cells from human exfoliated deciduous teeth (SHED), dental follicle stem cells (DFSCs) and periodontal ligament stem cells (PDLSCs) (113–117). Notably, several of these stem/progenitor cell populations have been proposed to contribute to dentine‐pulp complex healing. In addition, animal models have also shown that there is the potential that SCs external to the tooth can be attracted to migrate into the pulp to enable tissue repair (35, 112). Indeed, recent data using bone‐marrow fibrocytes have shown that they can migrate to the injured pulp to participate in early wound healing events including new blood vessel formation (82). It is evident that while there is a lack of consensus regarding the exact progenitor population involved in reparative dentinogenesis, marker expression analysis indicates that several cell types of mesenchymal origin may contribute to this process (118). Notably, at a molecular level, the relative influence of both dentine and pulp cell‐derived factors on tertiary dentinogenesis is currently impossible to quantify; however, the healing process is likely influenced by their temporal bioavailability and the tissue environment (83).
Immune‐Inflammatory Response in Dentine‐Pulp Complex Repair – A Double‐Edged Sword
While it is well known that the odontoblasts main function is regarded as being responsible for dentinogenesis, their immunocompetent role is also well described (119). During the early stages of caries and disease, the enamel and dentine become demineralized and soften due to the action of acids produced by the cariogenic bacteria (120). This process will further open up the porous tubular dentine, allowing bacterial products and components to traverse the hard tissue where they will initially be detected by receptors on the odontoblasts, leading to activation of the immune and inflammatory response beneath the lesion (121). If the carious environment is not appropriately arrested either by partial or complete caries excavation, the invading microbial biofilm will further evolve and will become mainly anaerobic in nature deeper within the pulp tissue (122–124). At the same time, the inflammatory response begins to amplify, and disease progression can lead to irreversible pulpitis, pulp necrosis and may ultimately result in apical periodontitis (125).
The molecular signalling response which occurs during the progressing infection is complex as it drives the host’s defensive reaction within the pulpal tissue. In addition to the odontoblasts, pulpal cells including fibroblasts, stem cells, neurones and endothelial cells, express receptors which detect bacterial components. Members of the TLR family (TLRs 1‐9) are shown to be present on many of the cell types within the pulp where they sense the presence of bacterial wall components, such as lipopolysaccharides (LPS) and lipoteichoic acids (LTA), and bacterial DNA (126–128). In particular, TLR2 and TL4 have important roles in early pulpitis and the innate immune response to bacterial infection. Specifically, TLR2 is involved in the recognition of peptidoglycans and LTA Gram‐positive bacteria, while TLR4 mediates the effects of LPS on Gram‐negative bacteria (39, 129). Once the bacterial ligand is bound, downstream intracellular signalling pathways are invoked, involving the nuclear factor kappa B (NF‐kB) and mitogen activated protein (MAP) kinase (130) pathways. Consequently, AMPs which directly aim to kill the bacteria are released, as well as inflammatory regulating cytokines invoking immune cellular responses including cell recruitment, extravasation, activation, differentiation and antibody production by immune cells. Classic cytokines present during disease in the pulp include the IL‐1α, ‐1β, ‐4, ‐6, ‐8, ‐10 and tumour necrosis factor (TNF‐α) (131). It is only once the infection is removed or the microbial load reduced to a critical level, allowing a reparative host response, for example, following caries removal, that the inflammation becomes resolved and the levels of cytokines decrease towards levels present during tissue homeostasis. However, if this clinical outcome is not achieved, then chronic molecular inflammation will persist along with tissue swelling, pain and tissue necrosis. Under these conditions, the possibility of any tissue repair processes occurring is severely limited (132, 133).
The role of neurogenic inflammation within the pulp is also significant. As described above, the dental pulp is highly innervated with pulpal nerves located at sites of injury. Once activated during disease, these nerves contribute to inflammatory signalling by the release of neuropeptides (27, 59). Indeed, neuropeptides including SP, CGRP and VIP are upregulated in pulps containing deep carious lesions as well as in teeth with symptomatic pulpitis (58, 62, 134). It is understood that neuronal sprouting and activation of the complement system, which are associated with pulpal inflammation, along with the local release of neuropeptides, mediate an enhanced protective pain reflex as well as a neurogenic inflammatory response which aims to combat infection whilst facilitating tissue healing and repair (18). The locally released neuropeptides contribute to the regulation of pulpal blood flow and, along with angiogenic GFs, they can facilitate tissue healing (27, 60). Further, as previously indicated, neuropeptides exhibit antimicrobial activities against many endodontic pathogens, and these molecules may therefore be important in determining disease progression (135).
Immunological Response in the Dental Pulp
Comparable with other peripheral organs and tissues including the skin, gastrointestinal tract and lungs, the healthy dental pulp contains immunosensory leukocytes, such as macrophages, DCs, mast cells and T cells, which are able to detect disease‐causing agents in the local environment and subsequently orchestrate the appropriate response (Figure 2.2) (40, 127, 136, 137). Indeed, studies have demonstrated that these immunosurveillance leukocytes can represent ~1% of the total cell population in human teeth (137). When pathogens are detected, leukocyte numbers significantly increase due to the signalling which occurs following the elevation of the molecular inflammatory process described in the previous section. This inflammation is aimed at being protective, with the ensuing immune response triggering the recruitment of additional leukocyte populations from the circulatory system. These leukocytes subsequently adhere to the endothelial cells lining the blood vessel walls and migrate out into the diseased site. The neutrophils are initially recruited to engulf and destroy the infecting bacteria; subsequently, there is a macrophage response which occurs following their development from monocytes, and this differentiation process is also regulated by the levels of the inflammatory molecules present. Notably, studies have reported on neutrophils and macrophages progressively increasing in diseased pulp tissue in response to irritants from the caries process (138, 139
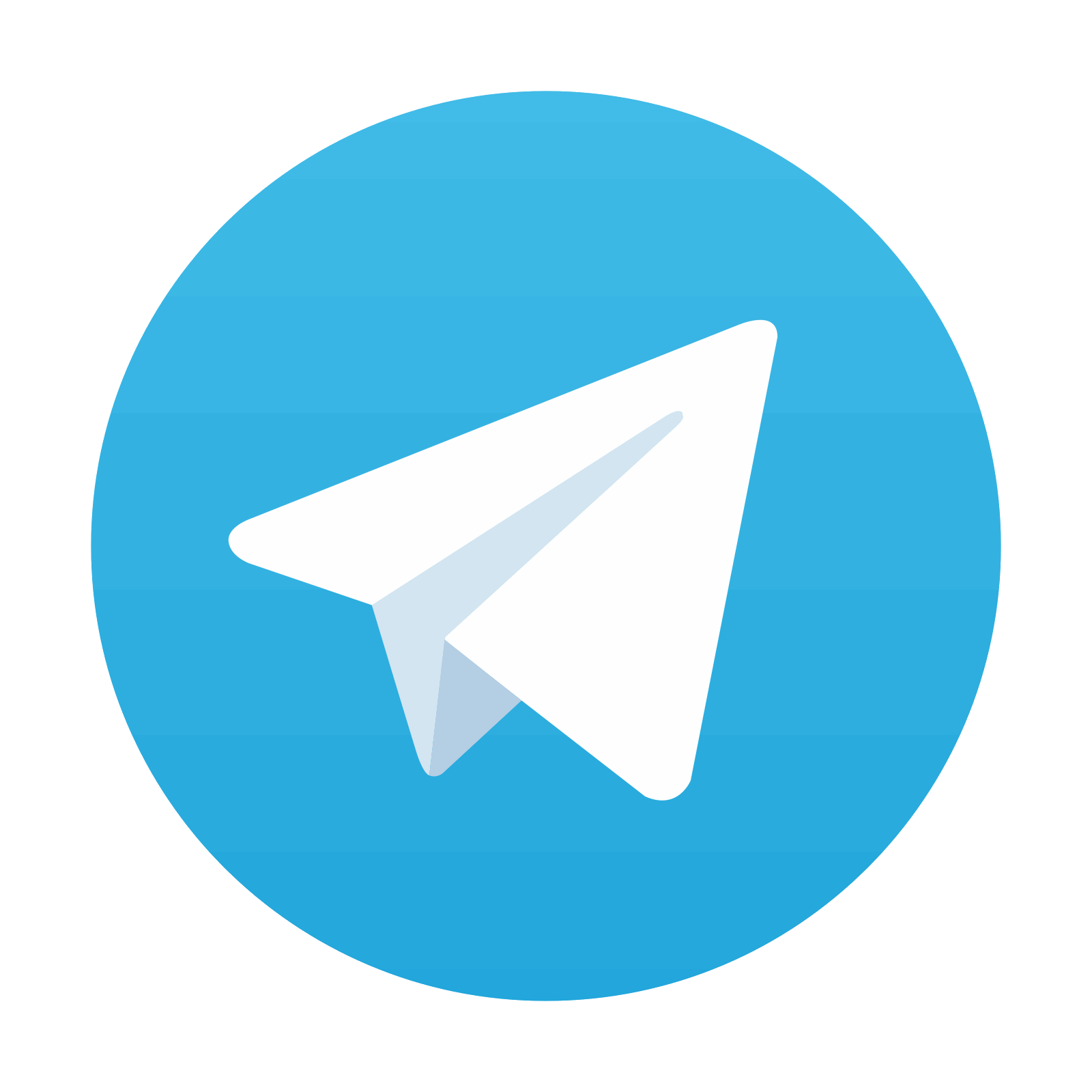
Stay updated, free dental videos. Join our Telegram channel

VIDEdental - Online dental courses
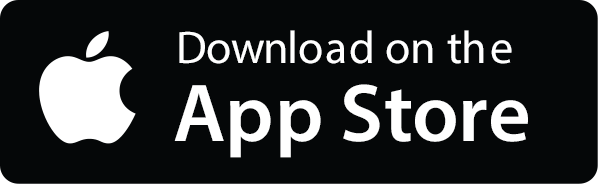
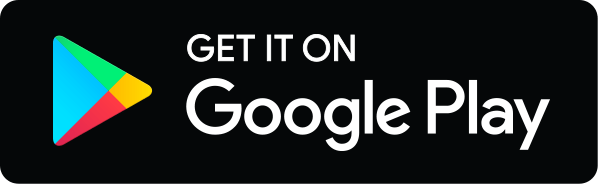