2
Biologic Basis of Bone Regeneration and Wound Healing
Unlike machines, the human body does not require space-demanding storage of spare parts; rather, self-repair unfolds on demand from a few pluripotent stem cells. These lie as space-saving pericytes in the walls of the blood vessels, which is practical because neoangiogenesis is already a prerequisite for tissue regeneration for nutritional reasons.
2.1 Bone Tissue Structure
Vascular supply and bone marrow
Bone consists of soft and hard tissue. The soft tissue includes the bone cells, the marrow, and the vessels necessary to supply them. Compact bone is nourished by the central vessels of the haversian system (named after Clopton Havers, English anatomist, 1650–1702) (Fig 2-1). Only the outermost layers are nourished by diffusion from the vessels of the periosteum. After surgical detachment of the periosteum, this part of the bone becomes insufficiently supplied. Among other things, this phenomenon is used as an explanation for why after periosteal detachment, a small surface resorption of the bone of about 0.5 mm is observed.
The maxilla has a rather spongy structure and a peripheral blood supply type. The maxilla is largely peripherally perfused with periosteal vessels via various arterial flow areas (greater palatine artery, anterior and posterior alveolar arteries, nasopalatine artery) through a plexus (Fig 2-2). Thus, the maxillary bone is still nourished via the periosteum even if parts of the internal vascular supply are interrupted, for example, by osteotomies or bone segment formation.
The mandible, on the other hand, has a much more sensitive vascular supply of the central type. Large parts of the horizontal branch are perfused almost exclusively via the central vessel of the inferior alveolar artery. Only the middle portion of the chin arch receives its perfusion through some vessels from the floor of the mouth. The central artery is often occluded as a result of arteriosclerosis, especially in older patients, in which case there is a reversal of flow in the mental artery.1 In addition to the nourishing vessels, the bone houses the hematopoietic tissue in the bone marrow, which is a fluid tissue without inherent stability. Inside the bone, unlike other sites in the body, the bone marrow is located in a place of mechanical rest and external stability. This is also the location of mesenchymal stem cells, which are the origin of bone healing.
Fig 2-1 a. Bone is one of the tissues with a strong blood supply. There are cross connections between the central vessels of the osteons, to the periosteum and into the bone marrow. b. In toluidine blue staining, a cross-section through cortical bone looks quite homogeneous (Laboratory MKG Kiel, hard ground section, pig, original magnification ×20). c. A similar preparation to 2-1b after intravital labeling with fluorescent dyes appears much more vivid. It shows the formation of osteons in compact bone. After illumination with UV light, the growth bands are shown by xylenol orange (2 and 3 weeks), calcein green (4 and 5 weeks), and alizarin complexone red (6 and 7 weeks) (Laboratory MKG Kiel, undecalcified hard ground section, pig, original magnification ×20).
Fig 2-2 a. Peripheral blood flow type in the maxilla via multiple periosteal vessels. b. Central circulation type in the mandible via the central vessel of the inferior alveolar artery.
Bone cells
The bone building cells possess progenitor cells (pluripotent mesenchymal stem cells, osteoprogenitor cells), which represent a regenerative reserve. In addition to the bone marrow, it is now known that the pericytes or perivascular cells of the blood vessels are the pluripotent mesenchymal stem cells.2 This is practical because there can be no major bone regeneration without a blood supply. When needed, these cells differentiate into osteoprogenitor cells and finally, after adherence to a matrix, into osteoblasts that build osteoid and then wall themselves into the matrix as osteocytes. Some cover the entire bone surface toward the medullary cavity without gaps as lining cells. If there are gaps in the lining cell layer, eg, due to surgical trauma, this is a signal for osteoclast development. This also explains the surface resorption after periosteal detachment.
Osteoclast function
Osteoclast function is under the influence of osteoblasts. Osteoblasts possess receptors for a wide variety of hormones and messenger substances and can produce RANKL (receptor activator of nuclear factor kappa-beta ligand) under the influence of parathyroid hormone. Together with M-CSF (macrophage colonystimulating factor), they thus control the confluence of monocytic progenitor cells into multinucleated osteoclasts. Osteoclasts are immune cells and, like macrophages, are derived from bloodstream monocytes, which in turn are derived from bone marrow hematopoietic stem cells. At sites where the lining cells detach, osteoclasts attach to the vacant bone sites. The attachment requires the protein osteopontin from the bone matrix, which binds in a ring with integrins from the osteoclasts. This creates a kind of suction cup for the osteoclasts, within which a very strongly acidic environment can be generated by proton pumps without the acid escaping into the rest of the tissue. The acid decalcifies the bone so that its proteins are now exposed for attack by acidic enzymes such as cathepsin. The Howship lacuna is formed (named after John Howship, surgeon and pathological anatomist, London, 1781–1841). By endocytosis, materials in solution in the lacuna are transported to the interior of the cell, where they are further digested. If bacterial lipopolysaccharide is present among these molecules, rapid inflammatory resorption can be initiated by Toll-like receptors,3 which usually leads to very rapid removal of bone or tooth tissue. In contrast to replacement resorption, inflammatory resorption does not fill the bone during bone remodeling; it is incompatible with bone formation due to the proinflammatory wound environment. It leaves defects and sequestra, so the bone surgeon takes preventive measures to ensure a low bacterial presence in the wound.
Basic bone substance
The hard tissue of the bone is a composite material consisting of fibers and filler, with the fibers absorbing tensile loads and the filler absorbing compressive loads. There are many examples of such composites in engineering (eg, reinforced concrete or glass fiber–reinforced plastic in boat building). The bone matrix consists mainly of collagen (fibers) and mineral in the form of hydroxyapatite crystals (filler). This mineral is practically insoluble in water at pH 7.4, but this changes rapidly at an acidic pH (as in caries formation). The mineral can be released from the bone composite by acid treatment to access the proteins of the bone. The bone mineral hydroxyapatite is the model for many bone substitutes; the body accepts certain technologically produced materials as artificial bone matrices, or the bone precursor cells cannot recognize them as foreign due to the lack of protein structures.
Nonsoluble hard substance proteins
The proteins remaining after dissolution of the mineral are divided into soluble and insoluble bone proteins. Collagen type I is the most important representative of the insoluble proteins in terms of quantity. The stability of bone is determined by its internal structure. This internal structure results from the direction of the collagen fibers, which, like wires, provide internal tensile strength to the bone. The internal direction of the collagen fibers can be visualized in polarized light. The basic building block of the mature cortical bone is the osteon with the central haversian canal. In the lamellar bone, the collagen fibers are wound around the osteon in a parallel, helical pattern in opposite directions, like circular plywood, which explains the high mechanical stability of this bone type. In contrast, the immature woven bone initially forms during wound healing. In woven bone, the collagen fibers are not arranged in parallel but in a braid-like pattern and can thus absorb forces evenly from all directions.
Fig 2-3 Bone components as they can be extracted by stepwise treatment with acids and solvents. On their own, bone morphogenetic protein (BMP) and bone matrix are inactive. Only when the individual components are recombined does an osteoinductive bone substitute material result. For example, recombinant BMP can be combined with bone substitute material to obtain an active graft.
Fig 2-4 The four temporally overlapping phases of wound healing in soft tissue.
Soluble hard substance proteins
The soluble bone proteins can be solubilized from the decalcified bone matrix by the solvent guanidine hydrochloride. The collagenous bone matrix then remains (Fig 2-3). The soluble protein fraction contains, among other things, signal molecules (growth factors and differentiation factors) and about 40 known bone-specific proteins, such as osteopontin, bone sialoprotein, and osteocalcin. The soluble fraction also contains bone morphogenetic proteins (BMPs; about 1 mg per kg bone). Allogeneic bone grafts are sometimes partially decalcified during manufacture so that the natural BMPs are more available in the wound. The material is called demineralized freeze-dried bone allograft (DFDBA).
2.2 Wound Healing
For didactic purposes, wound healing can be divided into four phases: exudative, inflammatory, proliferative, and remodeling. Every wound, whether in bone or soft tissue, passes through these four phases, which overlap in time (Fig 2-4).
Exudative phase (coagulum)
The first phase of wound healing is the exudative phase, which lasts from a few minutes to hours. It is characterized by hemostasis by platelets and polymerization of fibrin, which forms a provisional extracellular matrix. This is necessary for the storage of growth factors and as a scaffold structure for the migration of the wound-healing cells. When the bone is mechanically injured by an implant osteotomy, a defect is created. Bleeding into this defect occurs from the bone marrow. The coagulum adheres to the wound edges, and the wound is mechanically stabilized by coagulation and proteins, eg, fibronectin, in the first hours. Dentists are familiar with the importance of blood coagulum due to the clinical picture of painful fibrinolytic alveolitis (ie, dry socket).
Inflammatory phase (purification)
This phase is initiated by the degranulation of platelets and lasts for hours to days. The signal substances released, vasoactive substances such as bradykinin, act on the blood vessels in the wound margin. The blood vessels become permeable, and edema develops. The blood flow velocity slows down so that granulocytes can more easily become adherent to the vessel wall and ultimately leak through fine gaps between the endothelial cells into the perivascular space (diapedesis). Here they begin the destruction and phagocytosis of bacteria, chemotactically attracted by bacterial products such as lipopolysaccharides and by complement signals (opsonization = marking of bacteria by complement proteins). Chemotaxis means attraction with specific directional indication for migrating cells by a concentration gradient. The additional cell volume of the inflammatory cells is clinically recognizable as swelling. Granulocytes generate a toxic wound environment through free oxygen radicals when they occur in large numbers, for example, because they continually encounter new bacterial antigens. By disintegrating, granulocytes release tissue-dissolving enzymes from their lysosomes until the tissue is liquid and pus forms. Pus is a physiologic cleansing mechanism of the body in continuation of the work of granulocytes.
The granulocytes are followed by the macrophages, which can survive under the oxygen-deficient conditions of a wound margin and here can initiate new vessel formation and thus the next phase by secreting vascular endothelial growth factor (VEGF).
Proliferative phase (healing)
When a wound is clean, macrophages can secrete growth factors such as transforming growth factor-b (TGF-b), insulin-like growth factor (IGF), and VEGF. These factors induce the formation of the granulation tissue that replaces the provisional matrix of the coagulum. The proliferative phase overlaps with the adjacent phases and lasts for days to weeks. Stimulated by VEGF from macrophages, perivascular cells of the adjacent blood vessels detach, divide, and assemble into infiltrates. They coalesce and form tubes that connect to existing blood vessels. They can then be perfused, improving oxygen supply to the defect area. Following the vessels, fibroblasts migrate chemotactically. These encounter the stored growth factors in the extracellular matrix of the blood coagulum and begin collagen formation. Up to this point, wound healing is largely nonspecific and similar in soft and hard tissue wounds. However, the phases that now follow occur much faster in soft tissue than in bone.
BMPs are responsible for the difference between wounds in bone and those in soft tissue. They are stored in the bone and are released when the bone is injured by fracture or osteotomy. An important source is also bone chips (eg, via a bone scraper) or the drill dust in an implant socket, which should therefore preferably not be rinsed out before implant placement. BMP leads to the differentiation of mesenchymal stem cells into bone precursor cells. The resulting osteoblasts can only exist on a firm foundation and anchor themselves to bone structures in their environment, mediated by integrins and osteopontin. When these anchorages signal mechanical quiescence to the cells (mechanoreceptors), they begin expressing bone-specific matrix proteins (osteoid), starting from the injured bone surface.
If the proliferative phase proceeds undisturbed, the matrix mineralizes, and woven bone is formed within a few weeks (Fig 2-5). Bone formation can also proceed on osteoconductive surfaces within reach of osteoblasts. Osteoconductive surfaces are dental implants or bone substitutes (Fig 2-6), which must first be covered with a protein film. The fibronectin contained in this layer is used by osteoblasts for mechanical anchorage. An intermediate stage of cartilage (endochondral ossification), as in the extremities, does not occur in the jawbone, where intramembranous ossification predominates.
Fig 2-5 Initial woven bone formation in the course of a sinus elevation with bone substitute mixture after 6 weeks. Preosteoblasts condense and accumulate around an osteoid matrix, which is already increasingly mineralized in the direction of the right margin of the image (Laboratory MKG Kiel, undecalcified hard section, toluidine blue, pig, original magnification ×200).
Remodeling phase (conversion)
This phase lasts weeks to years in bone. It involves the load-dependent remodeling of immature woven bone (collagen fibers disordered in random direction) into mature lamellar bone (collagen fibers parallel in tensile direction). Freely grafted bone and, within limits, some bone graft substitutes are completely degraded over time and replaced by new bone. This remodeling is also load-dependent because it has been observed that bone is retained on occlusally loaded teeth and dental implants, whereas in unloaded areas of the jaw it is usually quickly degraded again.
Fig 2-6 Particles of xenogeneic bone substitute material (pink) are covered by blue woven bone during healing following sinus elevation and are bonded together and integrated into the bone. The foreign material is osteoconductive; it covers itself with fibronectin, on which osteoblasts attach (Laboratory MKG Kiel, undecalcified hard section, toluidine blue, pig, original magnification ×200).
2.3 Wound Balance: Proinflammatory and Anti-inflammatory Wound Environment
The image of a scale (Fig 2-7) helps the physician to understand wound healing as a quantitative problem, for example, the quantitative depletion of defense cells when bacterial inoculation becomes too high or the quantitative consumption of antibodies when antigens become prevalent.
In the wound environment, a distinction must be made between proinflammatory polarization and anti-inflammatory polarization as the two sides of the scale. Regeneration and tissue formation only occur in the anti-inflammatory milieu, while the proinflammatory milieu ensures degradation of the extracellular matrix of the tissue. The amount of collagen currently present in the tissue is the equilibrium of constant formation and degradation of collagen. When degradative enzymes are secreted by granulocytes, the balance is shifted in the direction of degradation, because the granulocytes make room for themselves in order to be able to move through the tissue more easily. The clinical effect is the bleeding tendency of the inflamed gingiva, which bleeds on probing because collagen has been degraded. The proinflammatory milieu (Fig 2-8) is accompanied by matrix metalloproteinases and other matrix-degrading enzymes and consequently a low content of extracellular matrix and growth factors. Furthermore, proinflammatory cytokines, free radicals, and an acidic environment are found. Acid belongs to inflammation and is part of the bacterial defense mechanism of inflammation. The proinflammatory milieu also includes the urokinase plasminogen activator (uPA), which leads to dissolution of the coagulum and can cause fibrinolytic alveolitis (dry socket). Other examples of non-healing wounds that chronically persist in the proinflammatory mode are leg ulcers or gastric ulcers. Chronic secreting wounds remain in the proinflammatory state.
The anti-inflammatory environment includes the opposite, ie, much extracellular matrix and many growth factors. Important components of the extracellular matrix are fibronectin and proteoglycans. Important tissue growth factors include fibroblast growth factor (FGF), IGF, and TGF. High levels of tissue inhibitors of metalloproteinases (TIMPs) inactivate proteolytic enzymes. The anti-inflammatory environment is slightly basic with the natural tissue pH of 7.4. A basic pH is part of regeneration. Such a wound no longer secretes and heals dry.
The scales of wound healing can therefore tilt in both directions (see Fig 2-8). In both environments, macrophages play the leading role; they can determine the further fate of the wound with their polarization (M1 or M2). M1 polarization promotes the inflammatory milieu by secretion of proinflammatory cytokines.4 The M2 polarized macrophages promote angiogenesis, oxygenation, and extracellular matrix assembly through secretion of growth factors.5
From what has been said, it is clear that tissue regeneration and thus alveolar ridge augmentation and osseointegration of an implant are tied to an antiinflammatory environment. Anything that lowers the pH (eg, decay products of polylactide materials), stimulates the release of proinflammatory cytokines such as IL-1b or tumor necrosis factor-α (TNF-α) (eg, macrophages upon antigen contact), or degrades extracellular matrix (eg, fibrinolysis) stands in the way of bone formation. So the physician should try to shift the wound balance to the anti-inflammatory side. An example of weighing the balance on the anti-inflammatory side is the introduction of extracellular matrix into the wound in the form of collagen membranes, collagen fleece, or collagen powder.6 This acts like a magnet on the hypervalent proteases of the inflammatory environment and causes a substrate inhibition of this enzyme so that the endogenous collagens of the connective tissue are spared.7 This is an additional explanation for the effectiveness of the collagen membrane in guided bone regeneration (see chapter 6).
Fig 2-7 Diagram of wound equilibrium intended to illustrate wound healing as a quantitative process. A non-healing wound contains M1 polarized macrophages of the proinflammatory environment; a healing wound contains M2 polarized macrophages of the anti-inflammatory wound environment. The macrophages are the switch point of the transition from the inflammatory to the regenerative phase. IL-1, interleukin-1; TNF-α, tumor necrosis factor-α.
Fig 2-8 a. The anti-inflammatory wound environment of a healing wound is characterized by a high content of extracellular matrix (fibrin, collagen, fibronectin) and growth factors (VEGF; TGF; FGF, fibroblast growth factor; KGF, keratinocyte growth factor; PDGF, platelet-derived growth factor) and by neoangiogenesis. Tissue inhibitors of metalloproteinases (TIMPs) prevent collagen degradation by inhibiting proteases. b. The proinflammatory or toxic wound environment of a non-healing wound contains cytokines such as TNF-α, many granulocytes, and a weak extracellular matrix with few blood vessels. In the marginal area, hyperemia is present; centrally there is a lack of blood vessels. The toxic wound environment contains oxygen radicals that kill bacteria and somatic cells. Aggressive proteases are also found, which break down collagen and ultimately liquefy the tissue to form pus.
2.4 Bone Remodeling
In the body of young healthy patients there is usually no bone older than 3 to 4 years; later in life the renewal period extends to about 10 years.8 Bone tissue is continuously and abundantly rebuilt. The continuous remodeling explains how, some time after a bone injury, the bone can regain its anatomically correct shape without a visible scar; how bone grafts heal; and how the bone can functionally adapt to the occlusal forces of a dental implant.
The balance between the responsible cells, the osteoclasts and the osteoblasts, is ensured by their molecular coupling, among other things, via the BMPs stored and chemically bound in the bone matrix. The cell complex consisting of degrading and remodeling cells is referred to as the bone multicellular unit (BMU) (Fig 2-9) and is the biologic basis of the equilibrium that keeps bone mass constant despite constant remodeling. At any given time, about 1 to 2 million BMUs are active in the body under healthy conditions.8
In the healing process of a bone block for augmentation, a distinction must be made between resorption from the surface, which costs augmentation height and volume, and internal resorption. Sometimes too much surface resorption of the bone is undesirable, eg, over the facial implant surface or after bone grafting. In contrast, rapid internal resorption with rapid graft remodeling is desirable. The resorption phase in the BMU lasts about 30 to 40 days, followed by reconstruction over approximately 150 days.9 After the bone is degraded by osteoclasts, a rest period of several days occurs during the reversal phase.10 The growth and differentiation factors (BMPs) bound in the bone are exposed on the surface. So-called reversal cells11 clean the cavity and line it with a protein film. This cement line serves as an attachment point for the osteoblasts that subsequently move in. The reversal cells can enzymatically detach the superficial fringe-like proteins protruding from the bone, including the growth factors, by serine proteases.12 The coupling of osteoclasts and osteoblasts, ie, the balance of degradation and attachment, is thought to be regulated by the matrix-bound cytokines such as IGF and BMP, among others.13,14
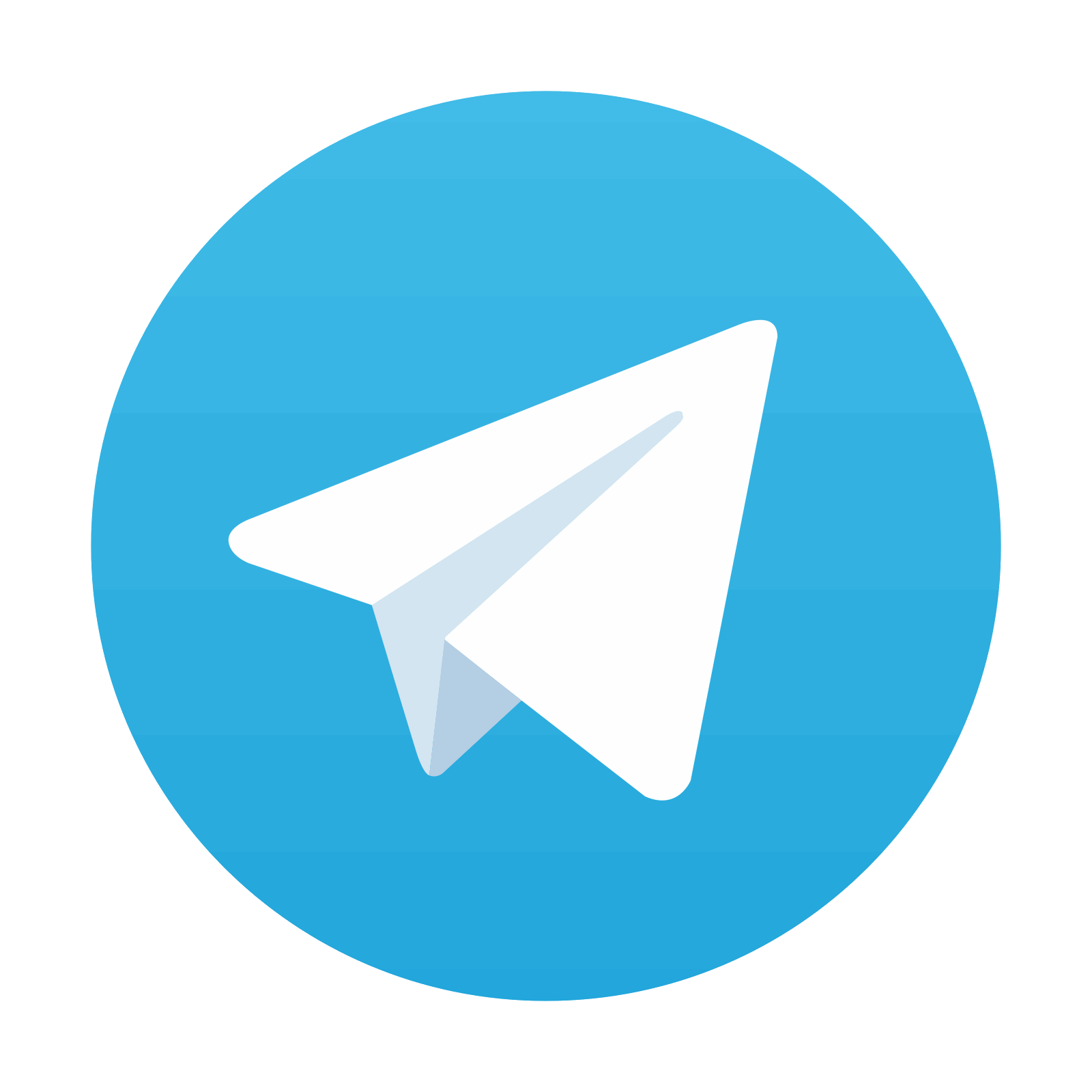
Stay updated, free dental videos. Join our Telegram channel

VIDEdental - Online dental courses
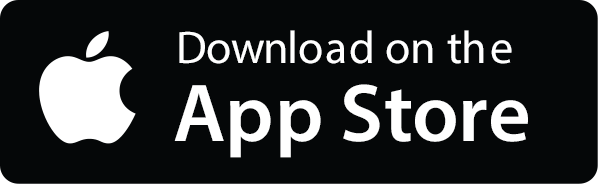
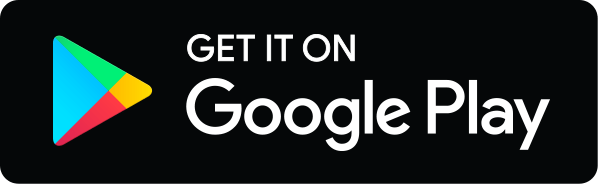