Abstract
Objectives
Biocompatibility of dental materials has gained increasing interest during recent decades. Meanwhile, legal regulations and standard test procedures are available to evaluate biocompatibility. Herein, these developments will be exemplarily outlined and some considerations for the development of novel materials will be provided.
Methods
Different aspects including test selection, release of substances, barriers, tissue healing, antibacterial substances, nanoparticles and environmental aspects will be covered. The provided information is mainly based on a review of the relevant literature in international peer reviewed journals, on regulatory documents and on ISO standards.
Results
Today, a structured and systematic approach for demonstrating biocompatibility from both a scientific and regulatory point of view is based on a clinical risk assessment in an early stage of material development. This includes the analysis of eluted substances and relevant barriers like dentin or epithelium. ISO standards 14971, 10993, and 7405 specify the modes for clinical risk assessment, test selection and test performance. In contact with breached tissues, materials must not impair the healing process. Antibacterial effects should be based on timely controllable substances or on repellant surfaces. Nanoparticles are produced by intraoral grinding irrespective of the content of nanoparticles in the material, but apparently at low concentrations. Concerns regarding environmental aspects of mercury from amalgam can be met by amalgam separating devices. The status for other materials (e.g. bisphenol-A in resin composites) needs to be evaluated. Finally, the public interest for biocompatibility issues calls for a suitable strategy of risk communication.
Significance
A wise use of the new tools, especially the clinical risk assessment should aim at preventing the patients, professionals and the environment from harm but should not block the development of novel materials. However, biocompatibility must always be weighed against the beneficial effects of materials in curing/preventing oral diseases.
1
Introduction
Clinical reactions related to dental materials comprise local effects in the direct vicinity, such as inflammation of the dental pulp or the adjacent gingiva. Furthermore, gingivitis due to increased plaque accumulation e.g. on composite resin fillings has been observed . Allergic reactions to dental alloys occur in sensitized patients displaying both extra- and intraoral symptoms. Localized lichenoid reactions of the oral mucosa next to dental materials may also have an allergic background. Resins, e.g. from resin based composites and adhesives may elicit allergic reactions both in dental personnel and in patients. Finally, systemic reactions, where the site of effect is distant from the site of exposure, have been claimed for mercury from amalgam for many years. More recently, bisphenol A (BPA) from dental resin composites is subject of discussion (see below). Besides these effects, which are based on eluted substances or released particles, adverse reactions may be due to physical damage, e.g. tissue burns by light curing units . Thus, biocompatibility of dental materials and devices is a clinically relevant topic and has raised increased interest in the dental scientific community, the profession and in the public. Herein, the experiences and developments from the last four decades of biocompatibility evaluation of dental materials will be exemplarily delineated and conclusions will be provided with respect to the design of new materials.
2
From single tests to a systematic approach
Tests on material-related adverse biological reactions and attempts to prevent them could be found only sporadically in the literature from the beginning to the middle of last century . For instance, in 1924 the effect of silicate cement on the dental pulp of dogs had been evaluated , furthermore the use of amalgam was discussed . In the 60s/70s of last century the number of such reports increased and different cell culture or animal methods were used for testing different dental materials .
However, as outlined above, dental material-related, clinically observed adverse reactions are diverse in nature (local, allergic, systemic) with different reaction mechanisms behind them. Furthermore, dental materials comprise a wide variety of chemically different products including but not limited to aqueous based cements, resinous materials, mixtures of both, metals and ceramics. Additionally, the mode of body contact with dental materials is varying: related to time, it ranges from minutes to months and years; related to the site of exposure it can be the intact or breached skin/mucosa, it can penetrate the body surface or it can be placed inside the body. Therefore, a more structured and systematic approach was deemed necessary. In the 70s and 80s of last century a set of tests for demonstrating biocompatibility was issued by the American Dental Association (ADA) as Specification No. 41 and by the Fédération Dentaire Internationale (FDI) as Technical Report No. 9 . Later, different and more specified standards were developed (see below). Legal regulations defining dental materials as medical devices and implementing safety requirements were initiated 1976 in the US, followed by the EU in 1993 and are existent virtually worldwide today . In these legal regulations, the safety requirements are based on risk classes, usually ranging from I to III, where class I denominates low risk and class III high risk .
Eventually, it became clear that a set of tests with different endpoints needed to be performed in order to provide full information on the “biocompatibility” of a material . For dental materials the following endpoints are part of most of the test programs:
- a
Cytotoxicity (in vitro).
- b
Genotoxicity/Mutagenicity (mainly in vitro).
- c
Local reactions (experimental animals or in vitro simulation tests like the dentin barrier test, see below).
- d
Sensitization (mainly experimental animals).
Other endpoints such as systemic acute, subchronic or chronic toxicity, cancerogenicity or reproductive toxicity have to be considered . Some of these tests require a high number of, or large, test animals. According to the concept of Threshold of Toxicological Concern (TTC) such endpoints may also be evaluated based on the amount of eluted substances from a material . For cytotoxicity evaluation in this context, usually permanent cell lines like 3T3 or L-929 fibroblast are used together with unspecific cell viability endpoints (e.g. MTT, MTS or neutral red assays).
2.1
Considerations for novel materials
It is obvious that a systematic approach for the evaluation of the biocompatibility of novel materials/substances is necessary and that toxicological knowhow must be included already at an early stage of material development. The final choice on the tests that have to be performed is based on a so-called Clinical Risk Assessment as delineated in ISO 14971 and ISO 10993-1 .
The ISO 10993 series has been developed for all medical devices (including dental materials) and rules for the selection of the appropriate test methods as well as the methods themselves are described. By now, this Standard comprises 20 parts and additionally a number of informative Technical Specifications/Reports. ISO 7405 is designed especially for medical devices used in dentistry and should be applied together with the ISO 10993-1 . In conclusion, ISO standards play an important role today, both in risk assessment and implementation of testing as well as in the evaluation of test results, because they can be used to meet the legal requirements for premarket biocompatibility evaluation. Therefore, wherever feasible, standard tests should be applied in an early stage of material development, where the new material should best be tested against a market product (relative toxicity analysis). As all preclinical tests used for certification/market access have certain limitations, biocompatibity evaluation must finally be complemented by a post market information systems that require dentists to report adverse effects in their patients either to the manufacturer or to other bodies and which are an important part of the whole risk management system .
2
From single tests to a systematic approach
Tests on material-related adverse biological reactions and attempts to prevent them could be found only sporadically in the literature from the beginning to the middle of last century . For instance, in 1924 the effect of silicate cement on the dental pulp of dogs had been evaluated , furthermore the use of amalgam was discussed . In the 60s/70s of last century the number of such reports increased and different cell culture or animal methods were used for testing different dental materials .
However, as outlined above, dental material-related, clinically observed adverse reactions are diverse in nature (local, allergic, systemic) with different reaction mechanisms behind them. Furthermore, dental materials comprise a wide variety of chemically different products including but not limited to aqueous based cements, resinous materials, mixtures of both, metals and ceramics. Additionally, the mode of body contact with dental materials is varying: related to time, it ranges from minutes to months and years; related to the site of exposure it can be the intact or breached skin/mucosa, it can penetrate the body surface or it can be placed inside the body. Therefore, a more structured and systematic approach was deemed necessary. In the 70s and 80s of last century a set of tests for demonstrating biocompatibility was issued by the American Dental Association (ADA) as Specification No. 41 and by the Fédération Dentaire Internationale (FDI) as Technical Report No. 9 . Later, different and more specified standards were developed (see below). Legal regulations defining dental materials as medical devices and implementing safety requirements were initiated 1976 in the US, followed by the EU in 1993 and are existent virtually worldwide today . In these legal regulations, the safety requirements are based on risk classes, usually ranging from I to III, where class I denominates low risk and class III high risk .
Eventually, it became clear that a set of tests with different endpoints needed to be performed in order to provide full information on the “biocompatibility” of a material . For dental materials the following endpoints are part of most of the test programs:
- a
Cytotoxicity (in vitro).
- b
Genotoxicity/Mutagenicity (mainly in vitro).
- c
Local reactions (experimental animals or in vitro simulation tests like the dentin barrier test, see below).
- d
Sensitization (mainly experimental animals).
Other endpoints such as systemic acute, subchronic or chronic toxicity, cancerogenicity or reproductive toxicity have to be considered . Some of these tests require a high number of, or large, test animals. According to the concept of Threshold of Toxicological Concern (TTC) such endpoints may also be evaluated based on the amount of eluted substances from a material . For cytotoxicity evaluation in this context, usually permanent cell lines like 3T3 or L-929 fibroblast are used together with unspecific cell viability endpoints (e.g. MTT, MTS or neutral red assays).
2.1
Considerations for novel materials
It is obvious that a systematic approach for the evaluation of the biocompatibility of novel materials/substances is necessary and that toxicological knowhow must be included already at an early stage of material development. The final choice on the tests that have to be performed is based on a so-called Clinical Risk Assessment as delineated in ISO 14971 and ISO 10993-1 .
The ISO 10993 series has been developed for all medical devices (including dental materials) and rules for the selection of the appropriate test methods as well as the methods themselves are described. By now, this Standard comprises 20 parts and additionally a number of informative Technical Specifications/Reports. ISO 7405 is designed especially for medical devices used in dentistry and should be applied together with the ISO 10993-1 . In conclusion, ISO standards play an important role today, both in risk assessment and implementation of testing as well as in the evaluation of test results, because they can be used to meet the legal requirements for premarket biocompatibility evaluation. Therefore, wherever feasible, standard tests should be applied in an early stage of material development, where the new material should best be tested against a market product (relative toxicity analysis). As all preclinical tests used for certification/market access have certain limitations, biocompatibity evaluation must finally be complemented by a post market information systems that require dentists to report adverse effects in their patients either to the manufacturer or to other bodies and which are an important part of the whole risk management system .
3
From observation to analysis
3.1
Release of substances
As mentioned above, biocompatibility testing of dental materials in the past was focused on a description of observed effects on live tissues, such as cell death or inflammation. However, in the course of developing a more structured approach to biocompatibility evaluation and in order to improve material biocompatibility, an analysis of the causes of such reactions became necessary. It was generally accepted that the basis for biocompatibility evaluation is the analysis of released substances from a dental material (eluate). Both the total amount of released substances and the composition of the eluate should be evaluated . This release is, however, not directly related to the composition of the material. For example, for many resin composite materials the organic phase is largely composed of mixtures of bis-GMA and TEGDMA. Although the total amount of bis-GMA is higher, the more hydrophilic TEGDMA is generally eluted in greater amounts in aqueous solvents . Furthermore, the composition of a material may be different in the bulk compared to the surface. An example for an alloy is shown in Fig. 1 : the surface and bulk of the alloy reveal a significantly different composition. The surface layer contains relatively small amounts of gold, but a high percentage of copper and silver. This correlation is reflected by the elements that are released (primarily copper) after 24 h . Another example is that – after firing – alloys used for metal ceramic crowns change their surface composition, but not the bulk composition. The release of metal ions increased significantly, as does cytotoxicity . Therefore, actual data on the release of substances are necessary as a basis for the evaluation of biocompatibility of each material.
It remains a matter of discussion whether the amount of eluted substances from polymerized resin materials is correlated with the conversion rate. According to Ferracane, a weak correlation exists between conversion rates and eluted residual monomers, if water is used as extraction medium. A stronger correlation was found for a mixture of water with ethanol . Tanaka et al. asserted that a clear correlation exists between conversion rate and the release of TEGDMA and Bis-GMA into an aqueous medium. However, for bifunctional acrylates the inclusion of only one functionality into the network is sufficient to prevent the molecules from elution. Therefore, the terms “degree of conversion” and “eluted substances” should be used separately.
In this context it needs to be mentioned that the amount of eluted substances is also dependent upon the preparation of the test samples. Thus, surface morphology of test samples should be standardized, because the release of substances also depends on the amount of available surface. For resin based materials, the oxygen inhibition layer on the surface will influence the amount and composition of the eluate .
3.2
Considerations for novel materials
The basis for any evaluation of biocompatibility is the determination of substances liberated from a new material. In this case as well, relevant standards can be applied such as ISO 10993 parts 12 and 18 . The relation of sample surface to volume of solvent should comply with ISO 10993-12 and at least a hydrophilic (e.g. saline) and a lipophilic solvent like ethanol or methanol should be used. Additionally, mixtures of 75% ethanol and 25% water have been proposed .
Relevant methods of chemical analysis of materials and eluates are described in ISO 10993-18 . Details for sample preparation for dental materials are described in ISO 7405 and should be meticulously followed and reported. However, the users need to realize that although the release of substances from a biomaterial is the prerequisite for a biological reaction, this does not mean that the mere presence of eluted substances in the tissues makes the material non-biocompatible. Other factors, especially the concentrations are also important. Nevertheless, the reduction of eluted substances from a new material should be considered during the developmental process.
3.3
Influence of barriers
If a residual dentin layer of more than 0.5–1 mm was present, no apparent pulp damage was observed in pulp studies with resin composite materials both in experimental animals and in humans , although dentinal adhesives or some resin composites are cytotoxic in direct contact with cells . The same is true for zinc oxide and eugenol: it is strongly cytotoxic after direct contact with cells , but with a residual dentin layer of >0.5 mm no apparent pulp damage can be observed histologically , which justifies its use as a non-toxic reference material in ISO 7405 pulp studies .
In a number of studies it was shown that dentin has a protective effect. Dentin acts as a diffusion barrier for substances liberated from dental materials . Permeability of dentin has been proven to be low, but it increases exponentially with a residual dentin thickness of less than 0.5 mm ( Fig. 2 ). Especially lipophilic substances like eugenol liberated from a zinc oxide and eugenol mix diffuse at a very limited rate through the hydrophilic dentin; thus the concentration at the cavity floor is high enough to kill bacteria, but low enough in the pulp where no damage can be observed after histological analysis .
Furthermore, dentin was found to be able to bind eluted substances from restorative materials, e.g. eugenol, which is bound to dentin components like calcium apatite and to certain proteins, such as albumin . Discoloration of the dentin floor under unlined amalgam fillings shows that metal ions from amalgam become bound to dentin. Furthermore, dentin is able to consume protons when in contact with acids. Therefore, a time limited (30 s) application of (citric) acid on dentin of >0.5 mm thickness did not significantly increase dentin permeability ( Fig. 2 ), because after a limited surface demineralization the available protons were consumed and no deeper demineralization occurred. Interestingly, we found that the antibacterial effect of a selfetch dentin primer (here against Streptococcus mutans ) was blocked after contact with dentin (see Fig. 3 ), which may be attributed to the fact that antibacterial protons were consumed by dentin and then were no longer available for inhibiting/killing bacteria. Dentin also acts as a barrier against heat, which is produced e.g. by the exothermic reaction of resin composites and additionally by light curing units . Thermal transfer increased exponentially with decreasing dentin thickness .
On the other hand, dentin contains odontoblast processes in the dentinal tubules. During cavity preparation these cellular processes become injured. However, with a thickness of the dentin layer of >0.5 mm this cell injury apparently does not lead to further pulp damage beside the formation of some reactionary dentin in the pulp at the end of the severed tubules . Furthermore, dentin under a carious lesion undergoes sclerosis and thus it can be assumed that the number of severed odontoblast processes is reduced.
Interestingly, dentin does not seem to be an effective barrier against bacteria and bacterial products that are present between resin composite filling and the bottom of the cavity . Such bacteria have consistently been found in cases of pulp inflammation under resin composite fillings, also if the residual dentin layer was larger than 1 mm . This means that a tight seal of a cavity by an adhesive restoration is considered to be protective against pulp damage. Technical and biological effects are interrelated.
The oral mucosa is composed of keratinized or non-keratinized epithelial cells, a base membrane and fibroblasts and it acts as a barrier concerning the resorption e.g. of metal elements or nanoparticles. Several methods have been described to simulate this situation in vitro. A three dimensional culture of epithelial cells from an oral carcinoma grown on a polycarbonate filter was used as a reconstituted human epithelium model , a different study used co-cultures of human fibroblasts and keratinocytes . Other methods are based on immortalized epithelial cells and fibroblasts grown on a mesh . An animal test method using the hamster pouch has been described and included into the Annex of ISO 10993-10 . However, experience in the published literature with all these methods for dental materials is rather limited.
3.4
Considerations for novel materials
If a novel material is cytotoxic, this does not automatically mean that it is not suitable as a filling material. It must be analyzed further whether the toxic substances have the potential to diffuse through dentin in sufficient concentrations. In such cases, the indications should be restricted, e.g. with the exclusion of direct pup capping. This also means that if the biological properties of novel materials are tested, barriers between materials and target cells should be implemented; in case of filling materials this would be dentin.
In order to mimic this situation, we developed the dentin barrier test , which can be used as an in vitro simulation test for cytotoxicity evaluation of novel dental materials intended to be used for cavity filling. In this test, a split perfusion chamber is divided into two compartments by a defined dentin slice. On one side of the dentin slice the test material is applied, on the other side a three-dimensional cell culture is inserted ( Fig. 4 ). With this method, zinc oxide eugenol showed no cytotoxicity, which is in agreement with clinical experience . On the other side, a RMGIC elicited a toxic reaction , which shows that the system reacts. This RMGIC was found to damage the pulp tissue when applied in deep cavities of <0.3 mm residual dentin thicknesses . Furthermore, the protective effect of increasing dentin thickness was demonstrated for a RMGIC in this test system . This test method has meanwhile been included into the ISO 7405 (Annex) . Similar test methods need to be evaluated for measuring increases in temperature e.g. due to the setting reaction of resin composite materials and the additional effect of light curing units. Some in vitro test have been described , but information on the correlation to in vivo studies seems insufficient .
Concerning the epithelial barrier, the passage of molecules and nanoparticles (see below) through such barriers are a topic of interest as these particles from materials but also from the ingestion of tooth pastes get into contact with the oral mucosa. Interestingly, some dental alloys have been used successfully in contact with the oral epithelium, but they were not biocompatible when used as implants .
3.5
Influence on cell metabolism
As a further step toward a better understanding of material tissue interactions, the influence of substances from dental materials on cellular metabolism was investigated at concentrations of the toxicant that are low enough to not lead to cell death or gross growth inhibition. Apparently, at concentrations of acrylate monomers (in this case HEMA and TEGDMA) which do not impair gross cell vitality and cell growth, cellular glutathione is consumed in order to detoxify the monomers . After consumption, glutathione is no longer available to balance the production of reactive oxygen species (ROS) from normal cell metabolism, which results in a ROS imbalance . The increase of ROS leads to the oxidation of DNA (generation of 8-oxoguanine) and to genotoxic effects . Furthermore, the cell cycle is delayed/arrested and eventually, apoptosis is induced, if the DNA damage is beyond repair . Due to the increase of ROS, cellular enzyme systems are differentially up- or down regulated (adaptive cell response) in order to compensate for the imbalance of ROS . Such processes consume energy and may lead to early cell senescence. The clinical implications are described below.
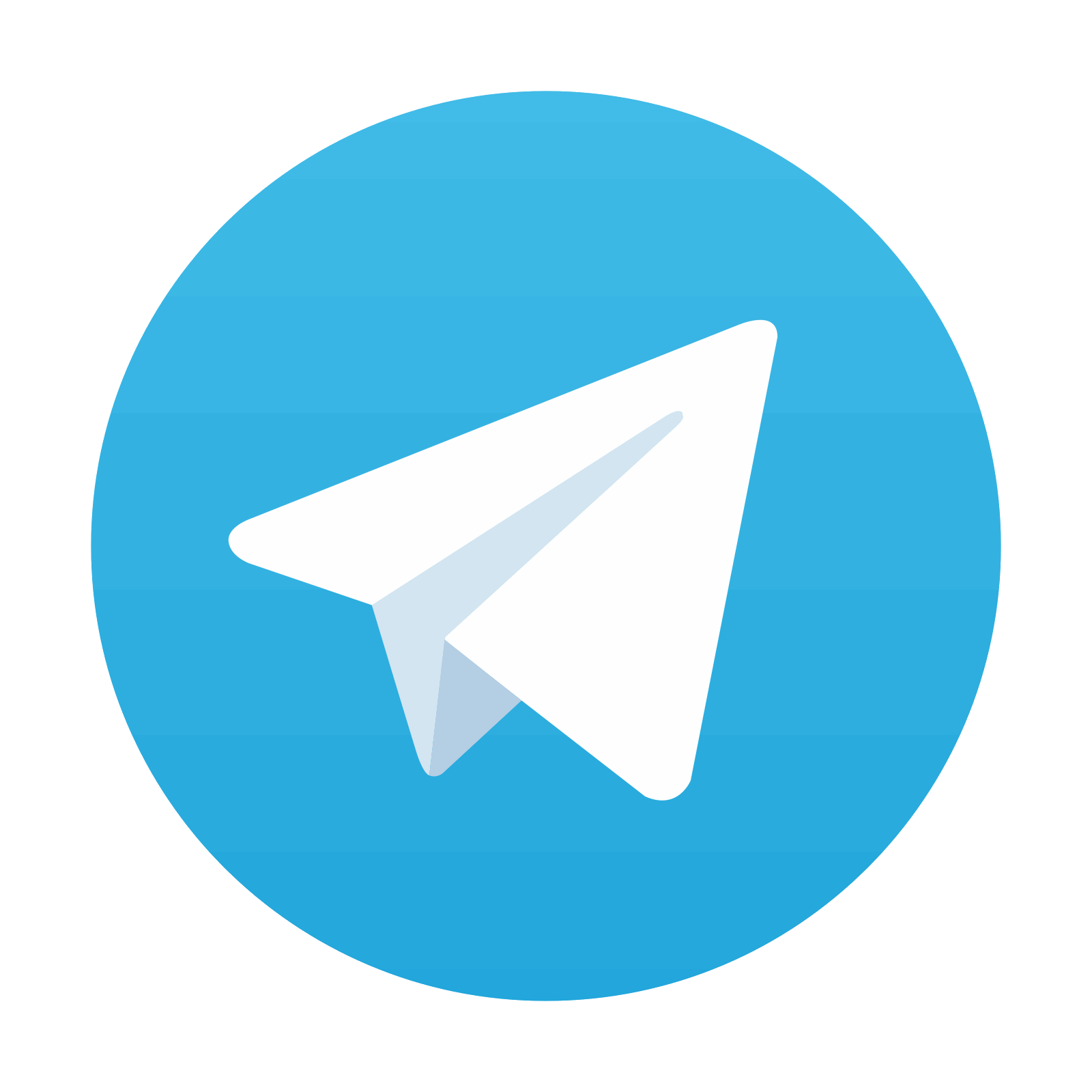
Stay updated, free dental videos. Join our Telegram channel

VIDEdental - Online dental courses
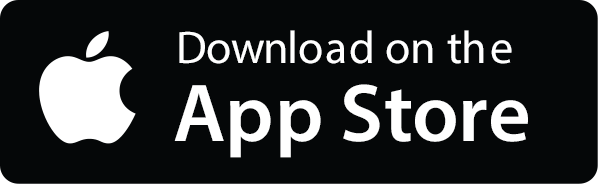
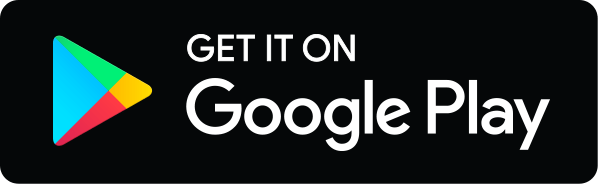