4
Bioceramics: Root‐End Filling Material
Sanjay Miglani1, Swadheena Patro2, Ankita Mohanty3, and Antarikshya Das4
1 Department of Conservative Dentistry & Endodontics, Faculty of Dentistry, Jamia Millia Islamia (A Central University), New Delhi, India
2 Department of Conservative and Endodontics, Kalinga Institute of Dental Sciences, Kiit University (deemed to be), Odisha, India
3 Conservative Dentistry and Endodontics Anew Cosmetics Centre, Bangalore, Karnataka, India
4 Department of Conservative Dentistry and Endodontics, Kalinga Institute of Dental Sciences, Kiit University (deemed to be), Odisha, India
4.1 Introduction
The primary goal of an endodontic treatment remains the chemomechanical disinfection of the root canal system, thereby leading to the removal of necrotic tissue and a decrease in bacterial load [1]. Although conventional treatment protocol must be considered first, considering it is more conservative, occasionally, the surgical approach proves to be inevitable [2]. Endodontic surgery typically entails resection of the apical portion of the root, followed by root‐end canal preparation and filling. The purpose of the retrograde filling is to create an apical seal around the canal, prohibiting microbes and related toxins from escaping into the periradicular tissues [3]. Therefore, it is safe to state that the objective of performing an endodontic surgery is to hermetically seal the root canal system and facilitate regeneration by eradicating bacterial contamination [4].
Endodontic materials in clinical use often have to meet certain requirements. They should be biocompatible, bioactive, should possess antimicrobial activity, be visible in radiographs, nonstaining to the tooth structures, dimensionally stable, should be easy to replace yet provide the best quality seal with hard tissues, and should have optimum mechanical strength [5–11]. It is evident that no material used in clinical dentistry, including endodontics, conforms with all the above‐mentioned criteria.
In the mid‐1990s, mineral trioxide aggregate (MTA) was the introductory bioceramic material which, on its clinical use for root‐end filling, aided in the development of numerous other hybrid or bioceramic materials. Essentially all bioceramic materials are biocompatible; they do differ in certain mechanical properties such as setting time, compressive strengths, etc. In conjunction to be used as retrograde fillings, these materials are utilized for pulp capping, repairing perforations, treatment of teeth with open apexes, and correction of defects caused by resorption. They are also employed as orthograde (apical) root fillings. Except for MTA, there isn’t much available literature on bioceramic materials, although it is expanding quickly.
This chapter emphasizes the properties, performance, application, and drawbacks of different bioceramics in endodontics when used in periradicular surgery as root‐end filling materials [12].
4.2 Microsurgical Endodontics
As quoted, Endodontic surgery is defined as a surgical procedure on exceptionally small and complex structures with an operating microscope. Modern techniques use ultrasonic devices that cut with almost negligent or no bevel, producing significantly better clinical results in contrast with carbide round burs that give a bevel of 45° when used for root‐end resection. The goal of a steep bevel is to facilitate more visibility of the root end during the procedure; however, this often leads to more damage leading to a large osteotomy.
In contemporary techniques, along with using ultrasonic devices, microscopes aid in performing the surgery with precision thereby providing a more predictable outcome of the procedure [13].
4.3 History
According to Saxena et al. almost every dental restorative material currently in use has been recommended to be used for root‐end filling [14]; among them are amalgam, glass ionomer cement, gold foil, super ethoxy‐benzoic acid (SuperEBA), gutta‐percha, and intermediate restorative material (IRM).
Farrar (1884), followed by Rhein (1897), placed amalgam as a root‐end filling material [15]. Abundant research supported the usage of amalgam for retrograde filling because of high success rates. However, it had its share of disadvantages such as corrosion, leakage, moisture sensitivity, tin and mercury contamination, preparation of retrograde cavities that require undercuts, etc.; therefore, amalgam is no more considered as the material of choice [16–18].
Schuster in 1913 introduced gold foil for root‐end filling; however, as a result of the complexities involving the placement, finishing, and high cost, it could not be used routinely [15].
In literature, there has been very little discussion about the utilization of GP as a root‐end filling material because it causes microleakage, owing to the porous nature of the material. However, Amagasa et al. in 1989 reported a good success rate with gutta‐percha being used for root‐end filling [19].
Zinc oxide eugenol (ZOE), an otherwise weak cement because of poor mechanical properties and high solubility, was modified to IRM and SuperEBA to revamp its mechanical properties. For its good sealing ability, Hendra in 1970 proposed SuperEBA as a root‐end filling material [20]. In a similar vein, a study conducted by Bondra et al. in 1989 concluded that IRM could be used as retrograde filling because it had remarkably less leakage as compared to amalgam. However, tissue irritation, difficult handling properties, sensitivity to moisture, and solubility are all characteristics that could not be ignored [21].
A composite resin developed by Bowen, stated to be hydrophobic in nature, limits its bonding to dentin (a wet substrate); therefore, a dry field and dentin bonding agents become indispensable for a composite to bond. Moisture control of the targeted area is required to allow predictable sealing and, as moisture is very often evident during the placement of a root‐end filling, the use of composite resin in periapical surgery for the root‐end filling was perhaps limited [13].
Among the major developments in reparative dentistry was the emergence of bioceramics as a new group of restorative materials in the early 1990s. Koch and Brave defined bioceramics as “ceramic products or components employed in medical and dental applications, mainly as implants and replacements that have osteoinductive properties.” [22]
Numerous authors have published studies comparing the characteristics of MTA as a retrograde material to those of other materials since 1993. The time frame required for Staphylococcus epidermidis to infiltrate a 3‐mm layer of retrograde fill made of amalgam, SuperEBA, IRM, or MTA was assessed in one study. Majorly, all samples containing amalgam, SuperEBA, or IRM showed leakage between 6 and 57 days, whereas the majority of samples containing MTA exhibited negligible leakage during the 90‐day study period [23].
Many such studies backed MTA as a root‐end filling material. Therefore, MTA along with other bioceramic cement will be discussed in detail in this chapter.
4.4 Properties of Root‐End Filling Material
The primary goal of root‐end filling material placement following root resection and root‐end preparation is to hermetically secure the root canal system, which will restrict either the microorganisms or their by‐products from leaving or entering the canal which may result in surgical failure.
Besides the obvious, there are certain other properties root‐end filling materials must possess to be a material of choice, as quoted by Shin et al. in 2017:
- Bacteriostatic or bactericidal
- Dimensionally stable
- Radiopaque
- Noncorrosive
- Resistant to dissolution
- Dentinogenic, osteogenic, cementogenic
- Adheres to tooth structure
- Nonstaining to the teeth or tissue
- Easy to manipulate [24]
Thus, the ideal properties should adhere to physical, biological, economic, and practical criteria.
4.5 Bioceramic Materials
Further in the chapter, we will discuss different bioceramic materials available, their composition, properties, contribution in periradicular surgery as root‐end filling material, and their impact on surrounding tissues (Table 4.1).
4.5.1 Mineral Trioxide Aggregate
Dr. Torabinejad developed MTA from Portland cement (gray powder) that was marketed as ProRoot MTA, following which, to resolve the aesthetic concerns, white MTA, a tooth‐colored formulation was introduced. Gray MTA comprises tricalcium silicate, tricalcium aluminate, tricalcium oxide silicate oxide, mineral oxide, and bismuth oxide (incorporated to make it more radiopaque). White MTA varies from gray MTA because of the lack of iron. The initial setting time is stated to be 4 hours; however, permanent setting requires at least 48 hours.
Table 4.1 Below is a table listing the most commonly available bioceramic cement for root‐end filling material.
Source: Abusrewil et al. [13]/with permission of Elsevier.
Product/manufacturer | Composition | Setting time |
---|---|---|
Grey ProRoot Mineral Trioxide Aggregate (G‐MTA) | Powder: tricalcium silicate, dicalcium silicate, bismuth oxide, tricalcium aluminate, calcium sulfate dihydrate or gypsum, calcium aluminoferrite | 165 min |
Dentsply Tulsa Dental Specialties, Johnson City, USA | Liquid: water | |
White ProRoot Mineral Trioxide Aggregate (W‐MTA) | Powder: tricalcium silicate, dicalcium silicate, bismuth oxide, tricalcium aluminate, calcium sulfate dihydrate or gypsum | 170 min |
Dentsply Tulsa Dental Specialties, Johnson City, USA | Liquid: water | |
Calcium‐enriched mixture cement (CEM) | Powder: different compounds of calcium, including oxide, sulfate, phosphate, carbonate, silicate, hydroxide, and chloride compounds | 50 min |
Bionique Dent, Tehran, Iran | Liquid: water‐based solution | |
Biodentine | Powder: tricalcium silicate, dicalcium silicate, calcium carbonate, zirconium oxide, calcium oxide, iron oxide | 45 min |
Septodont, Saint‐Maur‐des‐fossés, Cedex, France | Liquid: calcium chloride, a hydrosoluble (water‐soluble) polymer, water. | |
BioAggregate | Powder: tricalcium silicate, dicalcium silicate, tantalum pentoxide, calcium phosphate monobasic, amorphous silicon oxide | 1260 min |
Innovative Bioceramix, Vancouver, Canada | Liquid: deionized water | |
EndoSequence Root Repair Material Putty and Paste (ERRM) | Calcium silicates, zirconium oxide, tantalum oxide, calcium phosphate monobasic and filler agents | 4 h |
Brasseler USA, Savannah, GA, USA | ||
iRoot BP Plus Root Repair Material (BP‐RRM) | Calcium silicates, zirconium oxide, tantalum oxide/pentoxide, calcium phosphate monobasic | 2 h (The manufacturer) |
Innovative Bioceramix, Vancouver, Canada |
As mentioned earlier, MTA in comparison to amalgam, SuperEBA, and IRM is more resistant to bacterial contamination and fluid penetration. Recent studies reveal that when MTA comes into contact with tissue fluid, a layer of hydroxyapatite (HA) forms on the surface (also known as biomineralization) during its setting period, which is responsible for a biological seal between MTA and the dentin interface. According to histological studies, it was observed that MTA showed nearly negligible signs of inflammation when tested as a root‐end filling material in an animal model. As per a study conducted at the University of Pennsylvania on a dog model, new cementum‐like and bone‐like growth was observed atop an MTA root‐end filling (Figure 4.1). Varied in vitro cell cultures exhibited significant cell growth and attachments of several cells such as DPSC, human cementum‐derived cells, mouse primary osteoblasts, MDPC23, etc., thereby showcasing a positive biocompatibility profile (Figure 4.2). The high pH of MTA facilitates hard tissue formation or, more specifically, aids in tertiary dentin formation; thus, it suffices the criteria of being dentinogenic, osteogenic, and cementogenic. However, MTA comes with its share of drawbacks as well; for example, the probability of staining the remaining tooth structure, prolonged setting time, difficulty in the placement of MTA after retrograde cavity preparation, and so on. [24].

Figure 4.1 The pictures below demonstrate the root apex of a dog with ProRoot MTA. (a) Micro Computer Tomography. (b) Histological section.
Source: Shin et al. [24]/with permission from John Wiley & Sons.

Figure 4.2 Pictures under the scanning electron microscope of MDPC23 cells on (a) a plastic plate. (b) and (c) ProRoot MTA; showcasing the cell growth on the same under high magnification.
Source: Shin et al. [24]/with permission from John Wiley & Sons.
Several modifications of MTA have been brought into the scenario such as MTABio, MTA Angelus, OrthoMTA, and EndoCem MTA. However, more research is required to substantiate their uses in clinical practice [24].
4.5.2 Bioaggregate
MTA monopolized the research into root‐end filling materials for almost a decade until a new bioceramic cement called BioAggregate (calcium silicate–based cement). It was introduced by Bioceramics Inc. in 2006 and was promoted by DiaDent as DiaRoot. Leal et al. in a study concluded that there was no difference, when it came to leakage in retrograde cavities, between MTA and BioAggregate; both materials are equally noncytotoxic [25]. Like MTA, BioAggregate is also mixed from powder and liquid, deionized water is also provided with the same.
Personal preference dictates the preferred root‐end filling material between MTA or BioAggregate because both are expected to perform well. Although in the root tip, aesthetics is not an area of concern, in regions where it is, BioAggregate or Biodentine can be used as opposed to MTA. Keskin et al. deduced that the color stability of different calcium silicate‐based materials, when exposed to different irrigating solutions, was better in materials free of bismuth oxide [26].
4.5.3 Biodentine
Septodont introduced Biodentine in 2011 as a material that could be a replacement for dentin. In contrast to MTA, which showed mild to negligent reaction, Mori et al. stated that Biodentine exhibited moderated inflammation of the tissue on the seventh day. However, on the 14th day, both materials exhibited the same level of mild inflammation [27]. A lot of research proves microleakage with Biodentine is much less as compared to other materials. According to Grech et al., when compared to BioAggregate, Biodentine exhibited a high washout, thereby inferring that Biodentine filling must be protected while rinsing the retrograde cavity [28].
4.5.4 iRoot BP Plus Bioceramic Putty
This material is a water‐based bioceramic synthesized purely in the laboratory. Because it is available in a premixed hydraulic formula, it is fairly easy and convenient to use. A recent study on the cytotoxic profile of iRoot BP concluded that it was as biocompatible as MTA and does not incite critical cytotoxicity [14].
4.5.5 Capasio
Capasio (Primus Consulting, Bradenton, FL), a recently developed calcium aluminosilicate‐based material has been established in an attempt to enhance the shortcomings of preceding materials. Primarily composed of dental glass, bismuth oxide, and calcium aluminosilicate with a water‐based gel, its powder is mixed with its gel and liquid in a 4 : 1 ratio by weight [29]. This is moderately more acidic (pH = 10.9) than WMTA (pH = 11.6) when finally set at nine minutes. Besides, it has exhibited analogous or improved physical characteristics including radiopacity, compressive strength, setting time, washout resistance, and pH [29]. It has been noted that Capasio is more likely to enter dentinal tubules when used as a root‐end filling material because all its particles are less than 20 mm, with a mean particle size of 5.3 mm and, much like MTA, on exposure to synthetic tissue fluid it encouraged apatite deposition [30]. However, in contrast to MTA and Generex A, Capasio has not been demonstrated to boost primary osteoblast growth or promote nodule formation in a comparative analysis [31].
4.5.6 Calcium‐Enriched Material
Calcium‐enriched material (CEM) cement was first established in 2006 by Asgary et al. and is regarded to have great similarity to MTA, besides improved physical properties. The major and minor components of the powder in the descending order of their percentage weight include 51.75% wt. calcium oxide (CaO), 9.53% wt. sulfur trioxide (SO3), 8.49% wt. phosphorous pentoxide (P2O5), 6.32% wt. silicon dioxide (SiO2), aluminum trioxide (Al2O3), sodium aluminum oxide (Na2O), magnesium oxide (MgO), and chloride (Cl), 23.91% wt. respectively [32]. It creates a bioactive calcium and phosphate‐enhanced mixture when combined with a water‐based solution showing alkaline pH of 10.71 ± 0.19 and a setting time of about 50 minutes [33]. In addition to creating HA in simulated bodily tissue fluid, mixed CEM cement also does so in ordinary saline solution, the latter of which differs from MTA [34]. Additionally, the calcium and phosphorus ions that are released by this innovative cement from indigenous sources create a rich pool of hydroxyl (OH), phosphate (PO4) ions, and calcium (Ca2+), which are ultimately utilized in the synthesis of HA [35]. It should be noted that other biomaterials in normal saline solution do not exhibit this effect. When CEM is established, its small expansion, suitable flow, and film thickness (increased flow and decreased film thickness) can guarantee a strong seal and minimize any subsequent leakage, as well as having the capacity to encourage cementogenesis over both the material and dentinal surface at the root‐end. A noteworthy characteristic is the presence of entrapped cementocytes and insertions of periodontal ligament fibers in the newly produced eosinophilic cementum [36]
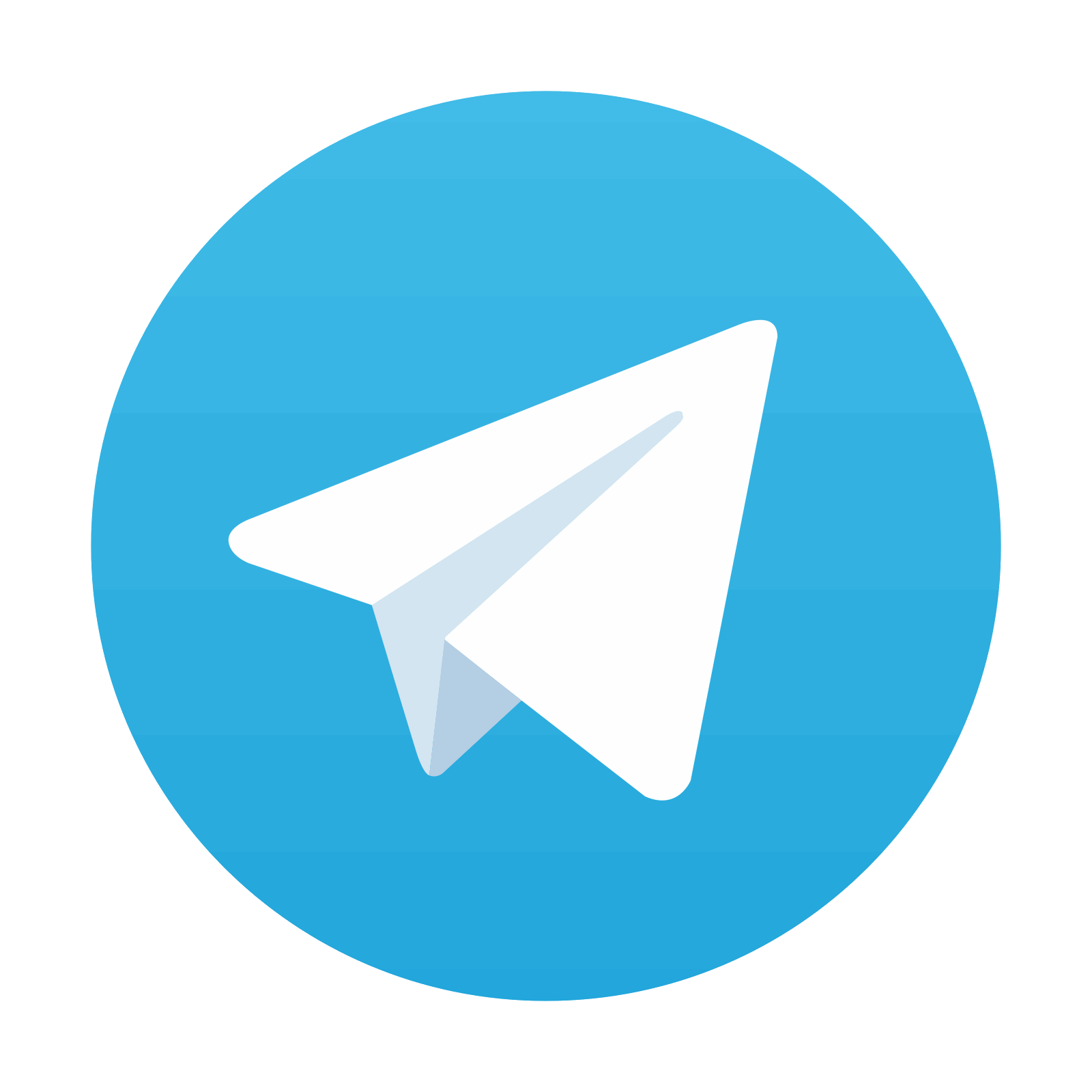
Stay updated, free dental videos. Join our Telegram channel

VIDEdental - Online dental courses
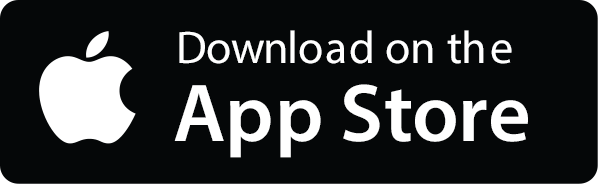
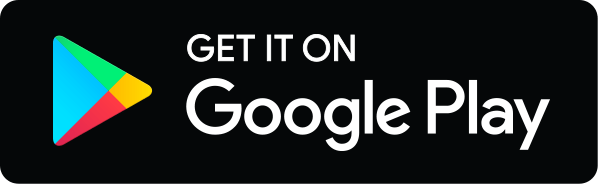