4
Augmentation Surgery Materials
The first successful cranioplasty in humans employed a piece of dog bone on an injured soldier and was published by van Meekeren in 1668.1 Transplantation of bones and soft tissues thus has a history of more than 300 years, with foreign materials from other humans, animals, or artificial materials being used from the beginning to reduce the trauma of graft harvest. Trauma related to graft harvest is highly dependent on the amount needed, with mostly small volumes needed in dentistry compared to most indications in orthopedic surgery. Therefore, in dentistry, one is not forced to take risks with foreign materials in dentistry.2
4.1 Properties of Foreign Materials
First of all, foreign materials should be biocompatible, ie, not enter into harmful chemical reactions with body molecules, eg, have a neutral pH, and not corrode to a significant extent. Furthermore, depending on the indication, it is important to be able to control the degradation and remodeling process, which can occur through solubility in water, chemically (eg, hydrolytically or enzymatically), and cellularly.
According to immunologic aspects, biomaterials are divided into autogenous (from the patient), isogenic (from identical twins), allogenic (from other people), xenogeneic (from other organisms), or alloplastic (technologically produced).
Chemistry and pH value
Some materials, such as bioglasses, release OH– ions and generate a strongly alkaline pH.3 Other materials, such as polylactides (polylactic acid), which are contained in some barrier membranes, resorbable films, or adhesives in bone graft substitutes, hydrolyze spontaneously in the body and release lactic acid. Acids are part of the proinflammatory tissue environment and trigger bone resorption rather than regeneration. Magnesium products corrode in the body and form hydrogen gas bubbles in the tissues. Even tissue-stable and normally corrosion-resistant materials, such as titanium, can corrode in the microenvironment of body cells or bacteria due to acid exposure,4 which is referred to as biocorrosion. On an even smaller scale, biomolecules such as albumin can create a corrosive nano-environment under the molecular body.5
Interface formation
Solid foreign materials that are not water soluble form permanent interfaces in the body. This is a problem with metallic implants such as hip replacements, which often have a limited life span and can become loose as a soft tissue layer develops between the bone and metal. Solid-liquid interfaces are also always susceptible to biofilm formation by bacteria if they are positioned close to the surfaces, because foreign materials have no defense of their own. One example is Teflon membranes for guided bone regeneration (GBR), which are prone to dehiscence or extrusion due to biofilms.
Particle-related sterile inflammation
Inorganic particles of tissue debris are removed in the body by patrolling macrophages by phagocytosis and digested intracellularly. These cells remove the material, break it down into smaller molecules in lysosomes, migrate to the lymph nodes, and present it in the lymph node to dendritic cells, which may prepare an immune response. Nondigestible metal particles migrate with the macrophages throughout the body. For example, titanium particles have been found in the liver after experimental implantation in the jaw.6 For these non-intracellularly digestible substances, particle size plays an important role.
Larger particles are not transportable at all and are encapsulated in place. In contrast, very small nanoparticles can pass through cell and cell nucleus membranes, in some cases without barriers, and cause changes in the cell nucleus. Therefore, caution is generally advised when using nanomaterials in wounds and defects.7 Medium-sized particles are problematic if they do not trigger encapsulation or clearance but frustrate phagocytosis. If the macrophage fails to internalize8 the particle, it can trigger sterile inflammation. For example, asbestos is dangerous not because of the chemistry but because of the size of the particles, because particle-induced inflammation can promote pulmonary fibrosis and eventually even tumors in the lungs. Substitute materials that contain or produce nonabsorbable particles of critical size in large quantities should be avoided.
Antigen structures through proteins
The organism distinguishes foreign from self on the basis of antigens that bind to receptors of immune cells. Full antigens are bound to structures of proteins and other organic molecules. Therefore, foreign materials containing organic materials should be considered with increased caution as transplants for tissue engineering. For example, an equine bone graft substitute (from horses) was known to cause a high rate of complications because the bone had not been deproteinized to reduce the brittleness of the material and improve its mechanical properties.9 Collagen is the main component of proteinaceous bone and soft tissue substitutes. The amino acid sequence of collagen is species specific and differs in pigs (porcine material), cattle (bovine material), horses (equine material), and humans.10 Our immune system reacts to molecules that were not in the body in the neonatal period when immune tolerance developed. In this respect, it is surprising that, in view of the mass use of proteinaceous foreign materials in dentistry, clinical adverse reactions are not greater.
Antigenic effect through haptens
Small organic molecules or certain metal ions (eg, nickel ions) alone cannot form a full antigen to trigger foreign recognition. They can, however, modify the tertiary structure of body proteins in such a way that they are interpreted as foreign by the immune system. Such substances are referred to as haptens or semi-antigens. Bone and soft tissue substitute materials should therefore not release any haptenic organic molecules (eg, plastic monomers) or metal ions into the tissue.
Cell-bound transplant antigens
Higher organisms had to defend themselves against parasites and tumors throughout their evolution. A foreign or degenerated cell is recognized by foreign antigens on its surface, which are presented by special proteins that are specific to a human. These are called human leukocyte antigens (HLAs) in humans and the major histocompatibility complex (MHC) in general. MHC class I presents intracellular antigens to the immune system and is used by all body cells except erythrocytes. MHC class II presents extracellular antigens and is produced only by certain cells, such as dendritic cells of the immune system. A foreign allogeneic cell (from another human) is recognized as foreign if it carries foreign MHC proteins, even if it has identical amino acid sequences at the protein level. This foreign recognition pathway leads to organ rejection in organ transplantation and is responsible for the necessary immunosuppressive medication of these patients. For this reason, manufacturers of allogeneic bone graft substitutes attempt to mitigate the cellular antigenicity of their products through purification processes, but this is not completely successful with block-shaped materials because residual foreign human cells remain in the marrow spaces and osteocyte cavities. Manufacturers of allogeneic soft tissue substitutes (eg, acellular dermal matrix) also try to banish cell-bound antigenicity from their materials.11
4.2 Bone Graft Substitutes
Certain biomaterials can replace the autologous bone graft and be incorporated by the bone without irritation, thus acting as passive defect fillers or active bone augmentation materials. In appropriate cases of well-healing bone defect types, the use of bone graft substitutes saves the patient from autogenous bone donation or reduces the need for autogenous or other bone grafts. The addition of bone graft substitutes can slow the uncontrolled resorption of autogenous grafts that sometimes occurs and reduce the amount of graft removed. Osteoblasts from the medullary cavity of the recipient bone grow appositionally along the surfaces of the bone substitutes (artificial extracellular matrix) into the defect. This process is called osteoconduction.
Bone substitute materials are usually passive fillers. The gradual appositional colonization of the bone substitute material with bone cells (osteoconduction) takes many months depending on the regeneration volume.
Micro- and nano-porosity
Successful bone graft substitutes exhibit micro- and macro-porosity and sometimes nano-porosity. Micro- and nano-porosity facilitates the attachment of matrix proteins such as fibronectin from the recipient wound, which in turn serve as a mediating layer to the bone cells.
Interconnecting macro-porosity
Macro-porosity of a bone graft substitute of about 200 to 600 µm12 is required to allow blood vessels to grow through an augmentation volume so that the necessary oxygen supply is still achieved even in the center of a large-volume defect. However, the pores must not be too large either, approximately 200 to 300 µm13; otherwise bone will not form on the surfaces of the bone graft substitute. The osteon with a diameter of 200 μm can serve as a model, the canal of which, starting from the central blood vessel, was circularly lined by bone at the time it was formed. No oxygen-dependent body cell such as osteoblasts can exist much further than 100 µm from the blood vessel.14 Tissue regeneration, especially bone regeneration, is always angiogenic. This means that bone formation is initially preceded by the formation of new blood vessels in the defect (Fig 4-1). To allow blood vessels to grow in, a bone graft substitute should have interconnecting porosity in addition to the above properties so that blood vessels not only find their way in but also find their way out as veins. Otherwise, there will be no blood flow. The requirement of interconnecting porosity is fulfilled by materials of natural origin (animals, algae, corals), while it is very difficult to produce artificially. If a material has already proven itself in the cancellous bone of an animal, then it can be assumed that, as a protein-free mineral framework, it has a macro-porosity that is favorable for the blood vessels.
Fig 4-1 Osteoinduction. A scaffold material (Bio-Oss Block, Geistlich) and recombinant human bone morphogenetic protein (rhBMP)-7 were implanted in a muscle. Macro-angiography with barium contrast on the left shows the connection of the graft to the blood supply. Micro-angiography on the upper right at ×50 magnification shows a newly formed blood vessel entering the block in longitudinal section from the left and many vessel cross-sections (white). It is striking that newly formed bone always appears at a constant distance from the vessels and only in the vicinity of a vessel. The image at bottom right shows the layer-by-layer deposition of new bone on a bone substitute surface within 6 weeks (Laboratory MKG Kiel, polychrome sequence labeling, pig, original magnification×100).
Degradability
A bone graft substitute should be as chemically inert as possible and produce a slightly basic pH in solution (blood = pH 7.4). Depending on the indication, resorption within months by spontaneous solubility (tricalcium phosphate [TCP]) or exclusive cellular degradation by acid (hydroxyapatite [HA]) with a correspondingly long service life is advantageous. There are also combinations of TCP and HA (biphasic calcium phosphate [BCP]). According to the German Society for Implantology (DGI) guidelines,15 a bone substitute material should be able to be degraded by the body in the long term, meaning that titanium metal particles and nondegradable polymers do not appear to be very suitable as biomaterials in implantology. For materials that are not water or acid soluble, the body generally has no degradation mechanism and its only response is encapsulation as a foreign body. Consider, in the case of peri-implant inflammation, that these encapsulated materials can come into contact with oral bacteria in the vicinity of dental implants and become infected.
4.3 Dentin as a Bone Graft Substitute
Dentin has a different structure than bone, but a very similar molecular composition. It also contains BMPs stored in the dentin matrix. It is therefore not surprising that dentin can be used to build bone. The observation already goes back to the Norwegian Gisle Bang, who published a study showing ectopic osteoinduction in guinea pig muscle by demineralized allogeneic dentin chips in a controlled experiment in 1972.16
In Korea, the method of using crushed allogeneic wisdom teeth was further developed into a commercial product and published in 1999.17 A first clinical study on 37 patients comparing this product to an alloplastic material was published in Korea in 2014,18 followed by a prospective randomized study on ridge preservation with autogenous demineralized dentin chips in comparison to xenogeneic bone mineral in 2017.19
In 2009, the first study on the use of human teeth as block grafts in bone defects was documented by Lars Andersson in Malmö.20 In Germany, Frank Schwarz and co-workers have addressed the issue and have already published a prospective controlled study on tooth block grafts compared to bone block grafts for alveolar ridge augmentation.21
Both methods, grinding the teeth and using them as block grafts, are still in the clinical experimental stage; severe complications have not been published so far.
4.4 Bone Products: Allogeneic and Xenogeneic Bone Grafts
Human (allogeneic) or animal (xenogeneic) bone can be used to produce bone regeneration products. Rejections of human allografts can occur due to the cell-bound MHC antigens, which in humans are also called HLAs and are commonly referred to as transplant antigens. Rejections of animal products can be based on both the cell-bound MHC antigens and additionally on the antigenicity of the foreign proteins.
The advantage of allogeneic and xenogeneic grafts in implant surgery is the avoidance of bone harvesting surgery, so that the patient does not have to endure the donor morbidity of bone harvesting, and so that even those surgeons who do not have the option of iliac bone harvesting can treat complex cases. It is even possible to order prefabricated allogeneic block grafts tailored to the patient’s individual defect via CAD/CAM using individual computed tomography (CT) data.
However, there is some evidence of healing problems with allograft blocks. A study from Germany on onlay allogeneic block grafts was terminated prematurely because healing complications occurred in over 80% of cases.22 A recent study from Israel on allogeneic block grafts showed dehiscence in 80% of cases and 25% block exposure as well as infections that required surgery and long-term antibiotics for resolution in 21% of cases.23
Allogeneic grafts are more commonly used as mineral bone substitutes in the United States, mainly in the form of demineralized freeze-dried bone allograft (DFDBA). In this case, freeze-drying serves to preserve the material, and partial decalcification breaks down the matrix so that the BMPs become more readily available for wound healing. In a direct comparison, allogeneic grafts performed worse than autogenous bone in various augmentation studies. For example, in a direct comparison of block bone grafts in humans, Spin-Neto and coworkers24 found 27.6% vital bone for the autogenous bone graft versus 8.6% for the allogeneic graft. In sinus floor augmentation, in a human study, the allogeneic graft was approximately halfway between autogenous bone and bone substitute in terms of bone density at 5-month biopsy.25
Microbiologic decontamination
Cadaver and animal products should be disinfected and preserved prior to sale, which competes with the attempt to preserve the activity of BMPs. Bacterial contamination of cadaver donations should not be underestimated. Before J. Choukroun became famous on the topic of L-PRF, he was known for his observations of gas-forming infections during sinus elevations with allogeneic grafts,26 which occurred because spore-forming germs had survived the disinfection process of the bone graft. A retrospective study showed 60% infections in the healing process after allogeneic graft transplantation in orthopedic indications, which healed without consequences in 92% of cases with antibiotic treatment.27 In a recent study, for example, 51% of allogeneic grafts from cadaver donors were contaminated with Klebsiella pneumoniae.28
Fig 4-2 Preparation classes of human bone allografts.
Preparation of human allografts
In addition to collagen, the protein fraction in bone also contains growth factors and BMPs. The art of industrial preparation of allografts is to remove the antigenic proteins and cellular components while preserving the desired BMP proteins (Fig 4-2). Bone contains the mineral scaffold, which can be rendered pure and used therapeutically by removing all proteins. However, such grafts should not be presented as osteoinductive. Allogeneic (human) bone products can be divided into three classes according to their preparation:
I. Fresh frozen human bone grafts: osteoinductive by BMP, MHC antigenicity, possibility of disease transmission from donor, only available through local bone banks at hospitals.
II. Gently disinfected human grafts: osteoinductive by BMP, limited MHC antigenicity, statistically low disease-transmission risk, durable commercially available industrial product.
III. Sterilized human grafts: only osteoconductive, no active BMPs but collagen proteins preserved, thus not osteoinductive; screwable, durable industrial product.
Fresh frozen allografts or xenografts (fresh frozen bone) of class I are critical because of their immunogenicity, risk of disease transmission, and lack of sterility (bacteria of deceased) and therefore should be viewed critically for dental indications (Fig 4-3). Most documented cases of HIV and hepatitis transmission to recipients through bone grafts fall into this category.29 In a prospective study of ridge augmentation in the maxilla, wound dehiscence and loss occurred with fresh frozen bone in 68% of patients,30 probably due to antigenicity.
Block versus particulate human allografts
A distinction should be made between block grafts and particulate grafts. In block grafts, it is not possible to completely remove the donor’s cells, leaving residual antigenicity of the MHC system. In particulate grafts, removal of cells and MHC antigenicity is technically possible according to a recent study.31 However, one of the particulate allograft products tested was pooled from different donor collectives. Unlike block allografts, these products lose traceability to a single body donor in the event of a problem.
Living, multi-organ, and cadaveric donors for human allografts
A distinction can be made between living donors and cadaveric donors according to the source of the allogeneic bone grafts. Living donor tissue comes from hospitals in cases of, eg, resected femoral heads during the insertion of hip joint prostheses. Living donors have the theoretical advantage that the window between viral infection (eg, with HIV) and seroconversion can be excluded by retesting 6 weeks after bone donation. Multi-organ donors are patients who are explanted within the organ transplant program in hospitals under sterile conditions and are tested multiple times. Cadaver bone also comes from distant countries. The extent to which these donors are tested should be clarified with the supplier of these products. It was reported in 1996 that HIV infection was established in 4 of 12 patients who had been treated with fresh bone grafts from an HIV-positive multi-organ donor.32 However, this occurred at a time when HIV testing could not be done routinely.
Fig 4-3 Transplantation of cryopreserved autogenous bone. a. Head of femur obtained during joint replacement surgery, cryopreserved for 2 years. b. Sinus floor defect and lateral ridge deficit. c. Cancellous bone and block material separated from the femoral head. d. Sinus lift and lateral ridge grafting. e. Osteosynthesis of the block material. f. Four months later implant placement into the successfully healed bone graft.
Preparation of animal xenografts
Xenogeneic bone products are commercially available in the following preparation levels:
I. Gently disinfected animal grafts: osteoinductive by BMP, protein antigenicity, HLA antigenicity, disease transmission risk from animal, durable commercially available industrial product.
II. Sterilized animal transplants: not osteoinductive (no active BMPs), protein antigenicity, limited HLA antigenicity, disease transmission risk from animal unlikely, shelf-stable commercially available industrial product.
III. Protein-free animal transplants: brittle and not screwable, durable industrial product, osteoconductive, no antigenicity and disease transmission.
Class I and II animal products are debatable because they can trigger immune reactions due to the foreign protein. For example, an equine block material, although very convenient to use with screws because of its collagen content, was withdrawn from the market because of antigenicity and high rates of wound healing disorders. In a prospective human study, wound dehiscence was observed in one-third of the patients and implant loss in another third.33 Antigenicity occurs in principle in all protein-containing animal products and is not linked to cells but to species-specific amino acid sequences, eg, of collagen. However, with minor species-specific differences in protein structure, this may often remain clinically inapparent.
Deproteinized xenogeneic bone chips
In the 1960s to 1990s, Kiel bone chip was known worldwide as “Kiel Graft.” It was the first industrially produced bone graft material, manufactured and distributed by the Braun Company (Melsungen, Germany).34 Through R. Maatz and A. Bauermeister, a method was developed at the University of Kiel in the 1950s to remove proteins from calf cancellous bone, thereby depriving the material of its antigenicity.35 In fact, however, the scaffold collagen was still present in the chip material, which gave the Kiel bone chip a certain strength but, according to experimental studies, nevertheless triggered considerable immunologic foreign body reactions. This was probably the reason why the “Kiel bone chip” was relatively rarely used, and autogenous bone material is preferred in many countries.34 The material looked like its market successor Bio-Oss (Geistlich, in collaboration with P.J. Boyne of Loma Linda University, California), in which it was possible for the first time to remove the protein completely. The exact preparation process remains a company secret to this day.
In 1980, J.F. Osborn then took an alternative successful path to bone replacement with synthetically produced hydroxyapatite.36
The problem of HLA sensitization by cell-containing human allografts
HLA sensitization can be induced by HLA antigens from cell surfaces of bone allografts, soft tissue substitutes, and platelet transfusions, as shown, for example, in a small case series of six patients from Brazil with sinus lifts using an allogeneic graft not approved in Europe and Germany.37 A meta-analysis found HLA sensitization in 48% of patients after bone allografts.38
This HLA sensitization may become problematic when a donor organ is needed later in life. However, it is still unclear whether this also applies to the rather small antigen quantities present in grafts for dental applications. Manufacturers of allogeneic bone substitute materials try to reduce the cell-bound antigen content of their products by purification processes. In the case of block-shaped bone pieces, however, this is not completely successful because residual foreign human cells remain in the marrow spaces and osteocyte cavities. According to a comparative study,39 the content of human MHC class I antigens (determined by enzyme-linked immunosorbent assay [ELISA]) in commercially available allogeneic grafts amounted to 0.037 to 0.04 ng per mg bone mass, compared to 0.084 ng in autogenous bone and 0 ng in protein-free xenogeneic materials. MHC class I is expressed by almost all nucleated cells. MHC class II molecules, normally expressed only by dendritic cells, were measured (using ELISA) at low levels of 0.004 ng per mg bone mass in the allograft osteograft, 0.189 ng per mg bone mass in autograft, and 0 µg each in allogeneic graft from a femoral head and protein-free xenogeneic materials, as expected.
HLA sensitization is one of the major organ rejection risks in kidney transplantation if antibodies against MHC class I or II are already present in the recipient’s blood before contact with the foreign organ.40
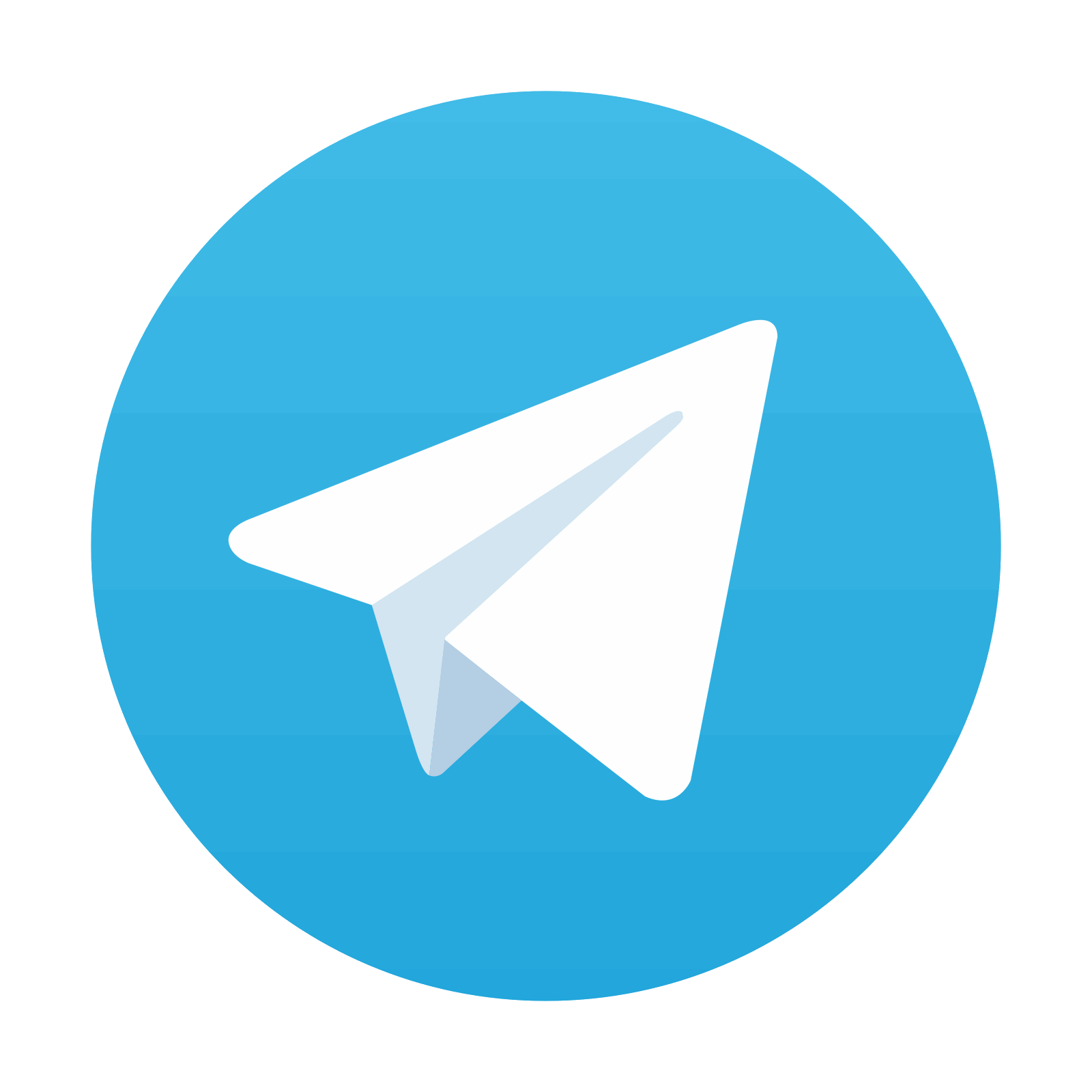
Stay updated, free dental videos. Join our Telegram channel

VIDEdental - Online dental courses
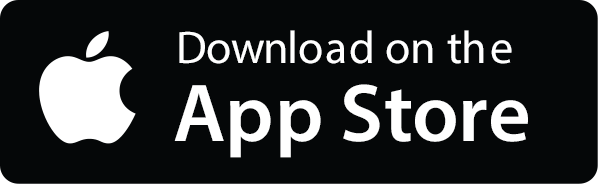
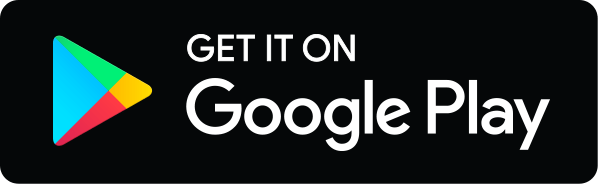