Abstract
The aim of this study was to assess the effects of low-level laser therapy (LLLT) applied to a dental extraction socket on thyroid gland function in a rabbit model, based on serum triiodothyronine and thyroxine levels. Sixteen male New Zealand rabbits were randomly distributed into two groups: a control group (non-irradiated animals) and an experimental group (irradiated animals: one irradiation point in the extraction socket of the lower incisor). Animals in the experimental group were irradiated with an aluminium gallium arsenide diode laser (AlGaAs; wavelength 830 nm, 40 mW, CW laser), for 13 days, every 48 h, at a dose of 6 J/cm 2 per session, resulting in a total dose of 42 J/cm 2 . Serum triiodothyronine and thyroxine levels were measured in both groups before extraction and on the last day of observation (day 15). There were no statistically significant differences between the groups in pre- and post-irradiation triiodothyronine and thyroxine values. With the irradiation protocol used in this study, LLLT did not affect thyroid function in rabbits as assessed by circulating serum triiodothyronine and thyroxine levels.
The rationale for the use of low-level laser therapy (LLLT) in clinical practice is supported by the ability of laser to stimulate molecular and biochemical processes involved in tissue repair at the cellular level. LLLT stimulates tissue repair and thus improves tissue regeneration and healing by increasing cell proliferation, accelerating the formation of granulation tissue, stimulating collagen synthesis, increasing the potential for remodelling and bone repair, restoring nerve function after injury, reestablishing normal hormone function, modulating and regulating the immune system, reducing inflammation and oedema, modulating and attenuating pain, and providing analgesia. Postoperative LLLT also promotes metabolic, energetic and functional changes to the irradiated surfaces, accelerating the healing process, allowing the evolution to cure in a short period of time. The potential use of laser therapy in the biomodulation of bone repair, made possible by its photochemical and photobiological properties, has been studied by several investigators as a means of providing surgical patients with faster bone regeneration, reduced postoperative discomfort and oedema, and improved tissue healing. Many in vivo and in vitro studies have reported beneficial effects of LLLT on the healing process in animal models and in tissue culture.
The first law of photochemistry (and photophysics) states that light must be absorbed for photochemistry (or photophysics) to occur. This simple concept is the basis for the success of photobiological experiments. Photobiological and phototherapeutic effects are initiated by photochemistry (or photophysics); therefore, unless light of a particular wavelength is absorbed by the system, no photochemistry (or photophysics) will occur, and no photobiological effects will be observed, independently of the time of irradiation.
The photochemical, photophysical, and photobiological effects of laser radiation affect deeper structures as well as the irradiated area. The biomodulating effects exerted by laser on cells at the irradiation site may also extend to tissues that are distant from the point of application. LLLT may therefore affect organs located far from the irradiation site.
After the penetration of photons in the tissue, some isolated laser light photons go through the tissue without producing any reaction (process known as transmission). This capacity of energy to diffuse through tissue without producing any effect ensures the light is transmitted until it reaches a specific tissue where the absorption characteristics are found. Then an effect may be observed. In a clinical situation, during a LLLT protocol following tooth extraction the laser beam can pass through the point of interest (extraction tooth socket) and reach the thyroid gland tissue, affecting the production of the thyroid hormones and, consequently, indirectly affecting bone healing as a side effect.
Although the use of laser has become common in the biomedical sciences, its possible side effects have yet to be clearly understood. Studies of the effects of infrared laser irradiation on the thyroid gland have shown increased mitotic activity in thyroid epithelial cells, transient hyperactivity in some follicles, and changes in circulating serum levels of triiodothyronine (T 3 ) and thyroxine (T 4 ). Moreover, structural or functional abnormalities in the thyroid gland may lead to deficient production of thyroid hormones (T 4 and T 3 ), affecting metabolism in all organs and systems of the body.
Dose is the most important parameter of laser therapy, but a definitive protocol for different clinical situations is still a matter of discussion in the literature ( Table 1 ).
Authors | Year | Wavelength (nm) | Power (mW) | Dose per session (J/cm 2 ) | No. irradiation sites |
---|---|---|---|---|---|
Pinheiro et al. | 2001 | 830 | 40 | 4.8 | 4 |
Weber et al. | 2006 | 830 | 50 | 10 | 4 |
Lopes et al. | 2007 | 830 | 10 | 21.5 | 4 |
Pozza et al. | 2008 | 830 | 30 | 10 | – |
Pinheiro | 2008 | 830 | 40 | 16 | 4 |
AboElsaad et al. | 2009 | 830 | 40 | 16 | 4 |
Rodrigo et al. | 2009 | 830 | 50 | 20 | 1 |
Kreisner et al. | 2009 | 830 | 40 | 10 | – |
Freddo et al. | 2009 | 830 | 50 | 10 | 2 |
Nascimento et al. | 2010 | 830 | 10 | 20 | – |
Hubler et al. | 2010 | 830 | 40 | 10 | – |
According to AlGhamdi et al. LLLT has shown a variety of effects and the stimulation of cells with LLLT must be examined in numerous contexts. It has been reported that irradiation of cells at certain wavelengths can activate specific biochemical reactions and alter the whole cellular metabolism. It is not possible to define the exact depth that the laser beam should reach, nor the dose that reaches the targets below the incidence point. It is possible that cells in different tissues may not respond to LLLT in exactly the same way and that similar parameters can have different effects on different cells.
The present study uses a wavelength of 830 nm, an output power of 40 mW, and an energy density of 6 J/cm 2 per session, which is the maximum dosage recommended by the manufacturer for irradiation of the dental extraction socket.
The objective of the present study is to assess the probable systemic effects of LLLT applied to a dental extraction socket on thyroid gland function by measuring circulating serum levels of T 3 and T 4 in rabbits.
Materials and methods
This study was approved by the Research Ethics Committee of the Pontifícia Universidade Católica do Rio Grande do Sul (PUCRS), Porto Alegre, Brazil (protocol no. 06/03417), and was conducted in accordance with the Ethical Principles for Experimental Research set forth by the Brazilian College of Animal Experimentation ( Colégio Brasileiro de Experimentação Animal , COBEA).
The study sample comprised 16 male New Zealand white rabbits ( Oryctolagus cuniculus ) aged 3 months and weighing 3–4 kg. Animals were randomly assigned to two different groups of 8 rabbits each: one experimental group (irradiated animals) and one control group (non-irradiated animals). Animals were fed according to the standard protocol of the authors’ animal hospital, namely solid diet (Purina ® , Nestlé Purina Petcare, St. Louis, MO, USA) and water ad libitum , and kept in a climate-controlled environment, under normal lighting, humidity, and temperature conditions. All animals underwent surgical extraction of the left mandibular incisor, thus creating equal baseline clinical conditions for both groups. The aim of tooth extraction was to simulate a common clinical situation, where LLLT therapy is used following tooth extraction with the aim of improving postoperative conditions and accelerating alveolar bone tissue repair at the extraction site.
Animals were anesthetized with an intramuscular injection of ketamine hydrochloride (Dopalen ® , Ceva Santé Animale, Libourne, France), 20 mg/kg, and xylazine hydrochloride (Anasedan ® , Ceva Santé Animale, France), 3 mg/kg. The area around the left mandibular incisor was disinfected with chlorhexidine digluconate 2% (FGM Produtos Odontológicos, Joinvile, SC, Brazil), and the tooth was extracted with the aid of #5 paediatric extraction forceps (Edlo S/A, Canoas, RS, Brazil) ( Fig. 1 A, B ). The socket was sutured with 4-0 nylon monofilament (Ethicon ® , Johnson & Johnson, Somerville, NJ, USA) ( Fig. 1 C).

An AlGaAs infrared laser (Thera Lase ® , DMC Equipamentos, São Carlos, SP, Brazil) was used at a wavelength of 830 nm, with 40 mW output power, and continuous spot irradiations for a total application time of 00:02:31 (151 s, automatically controlled by the laser device as determined by other parameters). The irradiation protocol was instituted immediately after surgical extraction in all animals in the experimental group. The irradiated animals received a single spot dose of 6 J/cm 2 directly on the centre of the extraction socket. The laser was applied with the irradiation tip placed perpendicularly over the socket ( Fig. 2 ). The irradiation was performed every 48 h, to a total of 7 applications over a 13-day period, giving a total dose of 42 J/cm 2 . Non-irradiated animals (control group) underwent simulated irradiation, which followed the same procedure used in the experimental animals but was performed with the device turned off ( Table 2 ).

Control group ( N = 8) |
Experimental group (LASER) ( N = 8) |
|
---|---|---|
Light source type | – | Laser (AlGaAs) |
Average power (mW) | – | 40 |
Wavelength (nm) | – | 830 |
Pulse parameters | – | CW |
Irradiation points | 0 | 1 |
No. applications per point | 0 | 1 |
Energy per point (J/cm ) | 0 | 6 |
Total energy density (J/cm ) | 0 | 6 |
Irradiation time per point (s) | 0 | 151 |
Total dose (J/cm ) | 0 | 42 |
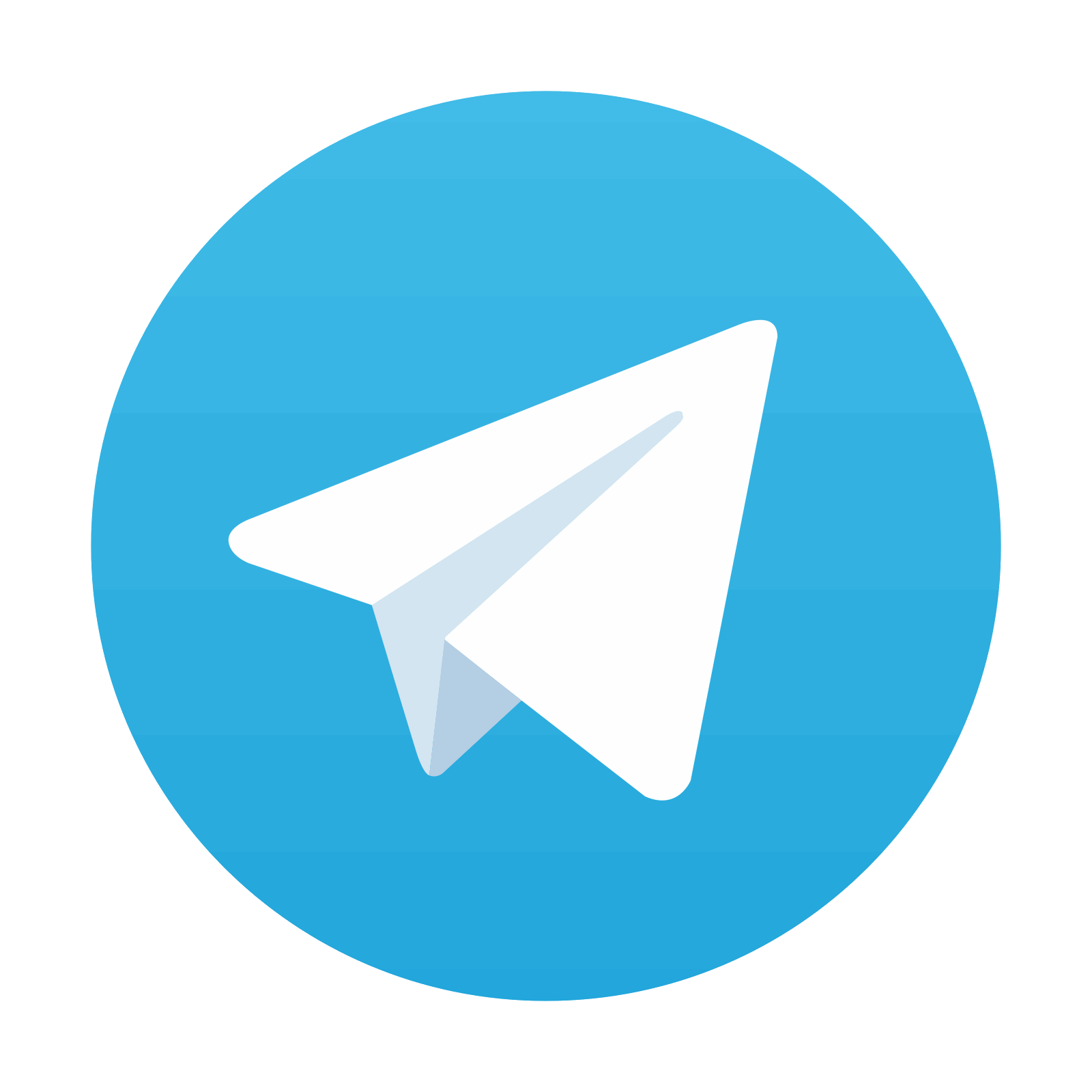
Stay updated, free dental videos. Join our Telegram channel

VIDEdental - Online dental courses
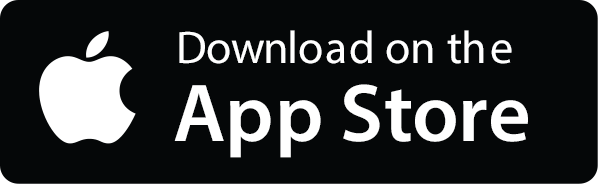
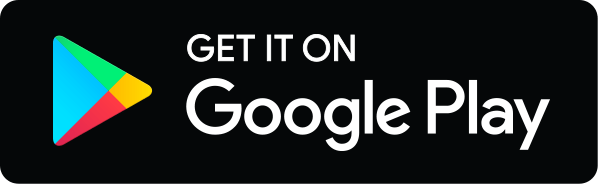
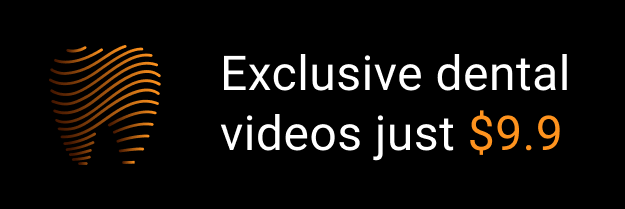