Introduction
The purpose of this study is to explore the feasibility of yttria-stabilized zirconia (Y-TZP) in fixed lingual retention as an alternative to stainless steel.
Methods
Exploratory Y-TZP specimens were milled to establish design parameters. Next, the specimens were milled according to ASTM standard C1161-13 and subjected to 4-point flexural tests to determine material properties. Finite element analysis was used to evaluate 9 novel cross-sectional designs, which were compared with stainless steel wire. Each design was analyzed under loading conditions to determine von Mises and bond stresses. The most promising design was fabricated to assess the accuracy and precision of current CAD/CAM milling technology.
Results
The superior design had a 1.0 × 0.5 mm semielliptical cross-section and was shown to be fabricated reliably. Overall, the milling indicated a maximum percent standard deviation of 9.3 and maximum percent error of 13.5 with a cost of $30 per specimen.
Conclusions
Y-TZP can be reliably milled to dimensions comparable with currently available metallic retainer wires. Further research is necessary to determine the success of the bonding protocol and the clinical longevity of Y-TZP fixed retainers. Advanced technology is necessary to connect the intraoral scan to an esthetic and patient-specific Y-TZP fixed retainer.
Highlights
- •
Yttria-stabilized zirconia is an esthetic alternative for stainless steel retainers.
- •
Y-TZP can be reliably milled to 0.48 mm in a curved geometry for a mandibular retainer.
- •
More research is needed to determine the bonding protocol and clinical longevity.
- •
Advanced technology is needed to fully personalize a milled retainer.
- •
A milled Y-TZP retainer is more expensive than stainless steel but has advantages.
Norman Kingsley is said to have claimed over 100 years ago that, “the success of orthodontia as a science and an art now lies in the retainer.” As clinicians, we must consider the stability of our result that relies on retention as the long-term goal of treatment. As excited as patients are on debond day, we should ensure that our retention protocol can maintain the desired finish and prevent the patient from having unnecessary retreatment. Treated patients are more cognizant than their untreated peers when assessing crowding, incisor irregularity, and alignment. Crowding and incisor irregularity are the most cited reasons for seeking orthodontic treatment. It seems to follow that postorthodontic retention would account for the mandibular anterior, a common site of crowding, as an esthetic priority for patients and subsequently affect the orthodontist’s retention design and protocol.
Relapse can be defined as any undesired movement away from an ideal finish after initiation of a retention protocol after orthodontic treatment, particularly in the mandibular anterior teeth. Various postretention studies have reported movement toward arch constriction, decreases in intercanine width, and increases in incisor irregularity and crowding with time. To counter relapse, fixed lingual retainers are the most commonly used appliance for mandibular retention in the United States among active members of the American Association of Orthodontists as of 2010. Fixed retention may be achieved by bonding round stainless steel wire, multistrand flexible braided steel wire, rectangular steel wire, or dead soft wire. Once a retention protocol and material are chosen, they are used for as long as the patient is compliant and desires to maintain the orthodontic finish. Of current American Association of Orthodontists members and 658 completed surveys, most orthodontists (75.9%) did not instruct patients to have fixed lingual retainers removed at a specific time.
The motivation to explore novel retainer designs and materials stems from the desire to improve the current retention protocol in terms of accuracy of fit, esthetics, bonding, and failure rate. Two recent articles in the American Journal of Orthodontics and Dentofacial Orthopedics have highlighted the complications that can arise from improper fabrication, placement, and irregular monitoring of fixed retention appliances. The overall failure rate of fixed retainers has been reported to be as high as 53% depending on placement technique and observation period. Failure of a bonded retainer can include debond, poor adaptation, or inadvertent activation, which may lead to crowding relapse, incisor irregularity, torque differences, bodily movement, or root dehiscence. Kucera and Marek reported a “twist effect,” where the contralateral canines had opposite buccolingual inclinations that were not categorized as relapse, as the most common complication. Although serious complications may be rare—up to 5% of patients—50% of them require retreatment. Thus, a new fixed retainer design and bonding protocol may mitigate the risks and better retain orthodontically positioned teeth when compared with stainless steel.
For our novel retention protocol, we considered both material and design changes beyond round and flexible braided stainless steel. Yttria-stabilized zirconia (Y-TZP) is an inert metal oxide ceramic that has been used in dentistry as an esthetic alternative to metallic alloys. Zirconia possesses ideal properties for dental use, including high flexural strength, high fracture toughness, fatigue resistance, excellent wear properties, resistance to chemical corrosion, biocompatibility, and esthetic appearance. We chose Y-TZP because, although it possesses some resiliency, it does not have the ability to store activation and enact orthodontic movement as stainless steel does. We also considered the current bonding protocol for Y-TZP involving 10-methyacryloyloxydecyl dihydrogen phosphate-containing luting agents to be clinically superior to current stainless steel bonding.
In addition to an improved bonding technique, another advantage of a zirconia retentive device is its ability to be milled to very specific dimensions and geometries. The advent of computer-aided design and computer-aided machine or manufacturing (CAD/CAM) systems has greatly improved the accessibility, design possibilities, accuracy of fit, sintering, and milling of restorations with materials such as Y-TZP. The level of customization that may be achieved by intraoral scanning could impart an individualized retention appliance that theoretically can be adapted much more precisely and deliberately to the lingual surfaces of teeth with appropriate technology. A personalized and adapted fixed retainer could increase the stability of anterior mandibular orthodontic results by minimizing the degrees of freedom of a tooth for incisor relapse and irregularity. Personalization is further advanced with the ability to shade-match the Y-TZP to the patient’s dental shade, imparting an esthetic advantage over metallic options. As of a current literature search, zirconia has not been used in a CAD/CAM-fabricated fixed retainer after orthodontic treatment. The purpose of this study was to explore the feasibility of Y-TZP as mandibular anterior fixed retention by assessing milling capabilities, material properties, and various designs with finite element (FE) analysis followed by analyzing the cost and practicality of potential clinical use. See Supplemental Materials for a short video presentation about this study.
Material and methods
To establish the parameters of current milling capabilities, 10 straight and 5 curved exploratory specimens were milled (B&B Dental Ceramic Arts, Renton, Wash). These specimens shared the same rectangular cross-section and 1.0 × 0.9 × 36.0 mm dimensions. Standardized descriptive measurements were completed to ensure sufficient milling accuracy and precision before progressing to more refined designs. Descriptive measurements for the straight specimens were made at even intervals across each, and the curved specimens were placed on a cast and marked at the mesiodistal midpoint of each crown. The initial exploratory curved specimens were based on the geometric measurements of a well-aligned postretention model, whereas all subsequent curved dimensions were based on a well-aligned dentoform. We defined 0.018-in and 0.036-in type 304 18-8 austenitic stainless steel as our controls. The 0.9-mm dimension was chosen as the closest millimetric measurement to 0.036-in diameter round wire. The 1.0-mm dimension was chosen to define a different measurement from 0.9 mm and to test the milling capabilities within 0.1 mm. All measurements were made with the same digital caliper (Precision 4-in Digital Caliper Alloy; EA Beck and Co, Santa Ana, Ca.) with the caliper arms placed perpendicular to the specimen’s surface where the measurement was being recorded.
Twelve Y-TZP specimens were fabricated (B&B Dental Ceramic Arts) according to ASTM standard C1161-13 , which defines the Standard Test Method for Flexural Strength of Advanced Ceramics at Ambient Temperature with a minimum requirement of 10 specimens. Dimensions for each specimen were 2.0 mm width by 1.5 mm height by 25 mm length. Standardized descriptive measurements were completed for each specimen to assess the milling capabilities. Height and width were measured at 5 evenly distributed areas on each specimen. Overall specimen length was also measured. All measurements were made with the same digital caliper (Precision 6-in Digital Caliper Alloy) with the caliper arms placed perpendicular to the specimen’s surface where the measurement was being recorded.
After the FE analysis, the design with superior results in the shear bond strength model was sent to the dental laboratory (B&B Dental Ceramic Arts) for fabrication. Ten specimens were fabricated and measured following this same descriptive measurement protocol.
All 12 ASTM-standard specimens were loaded into a 4-point flexural test attachment, placed in a testing machine (Instron, High Wycombe, United Kingdom), and loaded at a cross-head speed of 0.2 mm per minute in accordance with ASTM C1161-13 . Extension at failure was recorded, and maximum stress (MPa) and flexural modulus (GPa) were calculated for each specimen.
A straight specimen with ASTM-specified dimensions of 2.0 × 1.5 × 25.0 mm in rectangular cross-section was modeled in FE software (ANSYS, Canonsburg, Pa.). The specimen was subjected to a 4-point bend test in accordance with ASTM C1161-13 . This FE model was used to evaluate the maximum stress as a comparison with the experimental values observed and to validate the FE model use for Y-TZP in the project.
Nine novel Y-TZP designs varying in cross-sectional geometry and dimensions as well as 0.018-in and 0.036-in round stainless steel wires were modeled using the ANSYS software. Optimal mesh density was determined by a mesh convergence study. The overall length of each specimen and the defined height and width of each bonding area were defined according to tooth dimensions in Wheeler’s Dental Anatomy, Physiology, and Occlusion . A standard dentoform with comparable tooth measurements to those was used to further define the geometric parameters of each design. The bonding area was measured as the flat lingual surface between marginal ridges at the inciso-gingival midway point of each crown. The dentoform was used to determine the depth, arch width, and curvature of the lingual surfaces of the teeth for each design.
Eight of the 9 different Y-TZP and 2 stainless steel designs were included in our model, which assessed the bond strength to the tooth and the stress in the retainer resulting from an occlusal load. Design 2 was not included because it was judged not to be clinically applicable due to its large size. The model incorporated the materials’ properties determined from the experimental 4-point flexural test for Y-TZP and published values for stainless steel. The bonding areas of all 6 mandibular anterior teeth were defined as fixed points. An anterior occlusal force of 285.3 N was directed evenly across the superior-lingual aspect of each design. This represented the occlusal force being transmitted directly to the retainer, a common mode of debond failure seen in anterior mandibular retainers. The reaction force at each fixed bonding site was determined, and the shear stress at each bond was calculated for all 6 teeth using the bond areas for each design. The maximum von Mises stress in the retainer was compared with both the experimental and FE-determined flexural strength values. If the von Mises stress is below the strength of the material, then it is reasonable that the design will not fail. If the von Mises stress is above the material strength value, then it follows that the design is inadequate and will fail under the defined load. Furthermore, the safety factor was calculated for each design. Bond strength values were compared with literature values for Panavia F and Clearfil SE (Kururay, New York, NY) with accepted bonding protocol to assess expected clinical bonding performance under the simulated occlusal force. The maximum shear stress at any bonding point was determined for each design, and using 17.4 MPa as a reference, the safety factor was calculated and compared. Using this information, we were able to determine the best design to pursue for milling. Once milled, the average dimensions of the milled specimens were modeled with the same cross-section as the corresponding design and run in the bonding model to determine the same values.
Results
Figure 1 shows the 5 exploratory curved specimens that were milled to assess the initial capabilities for current CAD/CAM technology for curved retainers at this dimension. The average, standard deviation, percent standard deviation, and percent error were calculated for all specimens in each milled group—exploratory straight, exploratory curved, ASTM-standardized, and design 4 ( Table I ). From the data, we recorded the maximum percent standard deviation and percent error for any specimen in each group to determine the limit of milling capabilities. When we assessed the milling for the initial exploratory straight Y-TZP specimens with a rectangular cross-section (1.0 × 0.9 × 36.0 mm), the maximum percent standard deviation for any 1 specimen was 5.2, and the maximum percent error was 7.3. When we assessed the milling for the initial exploratory curved Y-TZP specimens with rectangular cross-section (1.0 × 0.9 × 36.0 mm), the maximum percent standard deviation for any specimen was 3.2, and the maximum percent error was 10.8. For ASTM-standard specimens, the maximum percent standard deviation was 4.2, and the maximum percent error was 4.8 (same specimen). The maximum percent standard deviation and maximum percent error calculated for any 1 specimen of design 4 were 9.3 and 13.5, respectively. Figure 2 shows the final 10 specimens of design 4 including its fit on the dentoform to highlight the adaptation and scale.
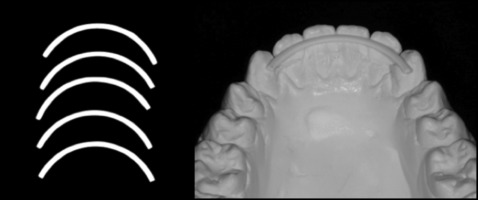
Wire | Nominal measurement (mm) | Average (mm) | SD | % SD | % error |
---|---|---|---|---|---|
Exploratory straight (1.0 × 0.9 × 36.0 mm) | |||||
Width | 1.0 | 0.96 | 0.01 | 1.0 | 4.0 |
Height | 0.9 | 0.87 | 0.03 | 3.8 | 3.3 |
Length | 36.0 | 36 | 0.03 | 0.1 | 0.0 |
Exploratory curved (1.0 × 0.9 × 36.0 mm) | |||||
Width | 1.0 | 1.05 | 0.02 | 2.1 | 5.0 |
Height | 0.9 | 0.98 | 0.01 | 1.0 | 8.9 |
Length | 36.0 | Not measured | |||
ASTM (1.5 × 2.0 × 25.0 mm) | |||||
Width | 1.5 | 1.45 | 0.03 | 1.9 | 3.3 |
Height | 2.0 | 2.04 | 0.02 | 1.0 | 2.0 |
Length | 25.0 | 25 | 0.02 | 0.1 | 0.0 |
Design 4 (1.0 × 0.5 × 27.75 mm) | |||||
Width | 1.0 | 0.48 | 0.01 | 2.6 | 4.0 |
Height | 0.5 | 1.02 | 0.08 | 8.1 | 2.0 |
Length | 27.75 | 28.06 | 0.15 | 0.5 | 1.1 |
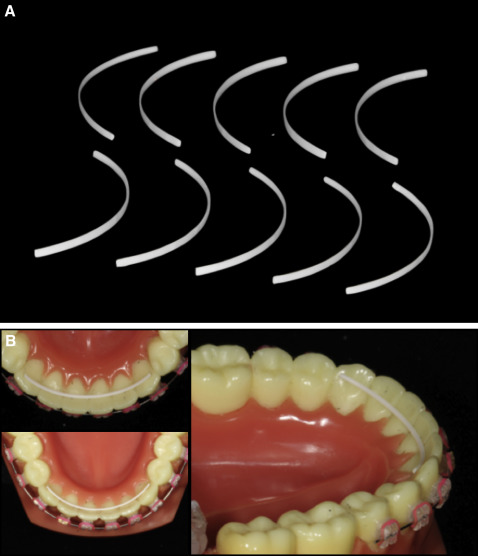
Table II summarizes the data obtained from the 4-point bend tests. The average and standard deviation are highlighted—556 ± 34 MPa for the experimentally determined data and 565 ± 35 MPa for data determined by FE modeling. The percent difference is listed for each specimen. The average percent difference was 1.7%, with values ranging between 1.6% and 2.7%. The extension at failure ranged from 0.2 to 0.31 mm in the experimental 4-point bend test, with an average of 0.24 mm and a standard deviation of 0.03.
Specimen | Experimental maximum stress (MPa) | FE maximum stress (MPa) | Difference (%) |
---|---|---|---|
1 | 547 | 556 | 1.7 |
2 | 530 | 539 | 1.6 |
3 | 591 | 600 | 1.7 |
4 | 506 | 514 | 1.6 |
5 | 534 | 543 | 1.6 |
6 | 563 | 572 | 1.6 |
7 | 519 | 528 | 1.6 |
8 | 556 | 565 | 1.7 |
9 | 601 | 617 | 2.7 |
10 | 574 | 583 | 1.7 |
11 | 534 | 542 | 1.7 |
12 | 613 | 623 | 1.6 |
Average | 556 | 565 | 1.7 |
Figure 3 summarizes the 9 Y-TZP designs and 2 stainless steel controls. Dimensions, cross-sectional geometry, and pictures of each are included. Table III summarizes the results for the mesh convergence study used to determine mesh size for each design. A prescribed element size of 0.05 mm was selected as appropriate for our modeling based on this analysis, which resulted in approximately 40,000 elements in each design. Figure 4 shows the schematic of the bonding model. Bonding areas are highlighted as well as the superior-lingual aspect of the retainer where the occlusal force was applied. Tables IV and V summarize the data obtained from the bonding model. The safety factors were calculated using both the experimentally and FE-determined stresses for the material. The values for both calculations are included in Table IV and used to determine the best designs. Designs 1, 3, 4, 4 milled, and 9 as well as the 0.036-in stainless steel control had calculated safety factors greater than 1.0 and are highlighted. Designs 1, 3, 4, 4 milled, and 9 as well as the 0.036-in stainless steel control had maximum bond stress values less than the literature-reported value of 17.4 MPa and calculated safety factors greater than 1.0.
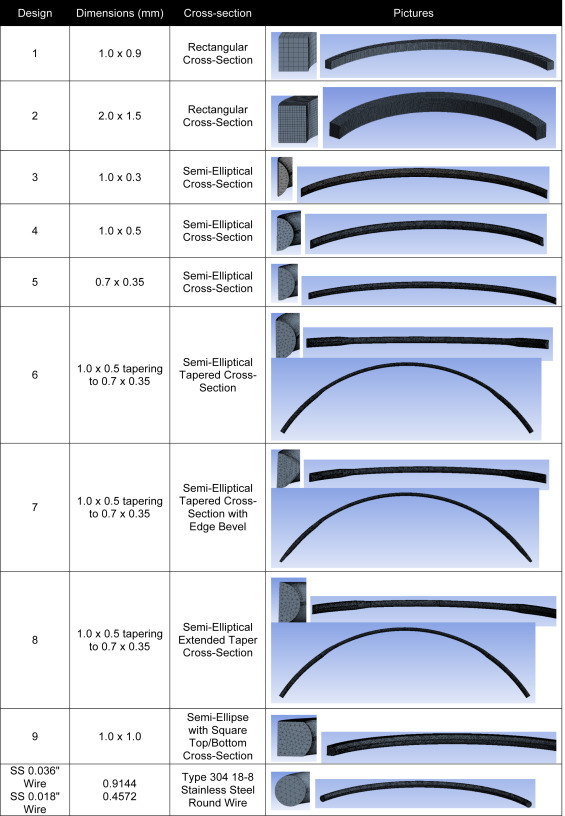
Mesh size (mm) | Maximum von Mises stress (MPa) | Percent difference | Nodes | Elements |
---|---|---|---|---|
0.5 | 92.222 | NA | 820 | 205 |
0.2 | 63.501 | 36.9 | 4417 | 1759 |
0.1 | 62.622 | 1.4 | 19349 | 9638 |
0.075 | 61.109 | 2.4 | 32894 | 16933 |
0.05 | 60.669 | 0.7 | 79742 | 43272 |
0.04 | 60.577 | 0.2 | 119969 | 66129 |
0.03 | 60.501 | 0.1 | 222236 | 124971 |
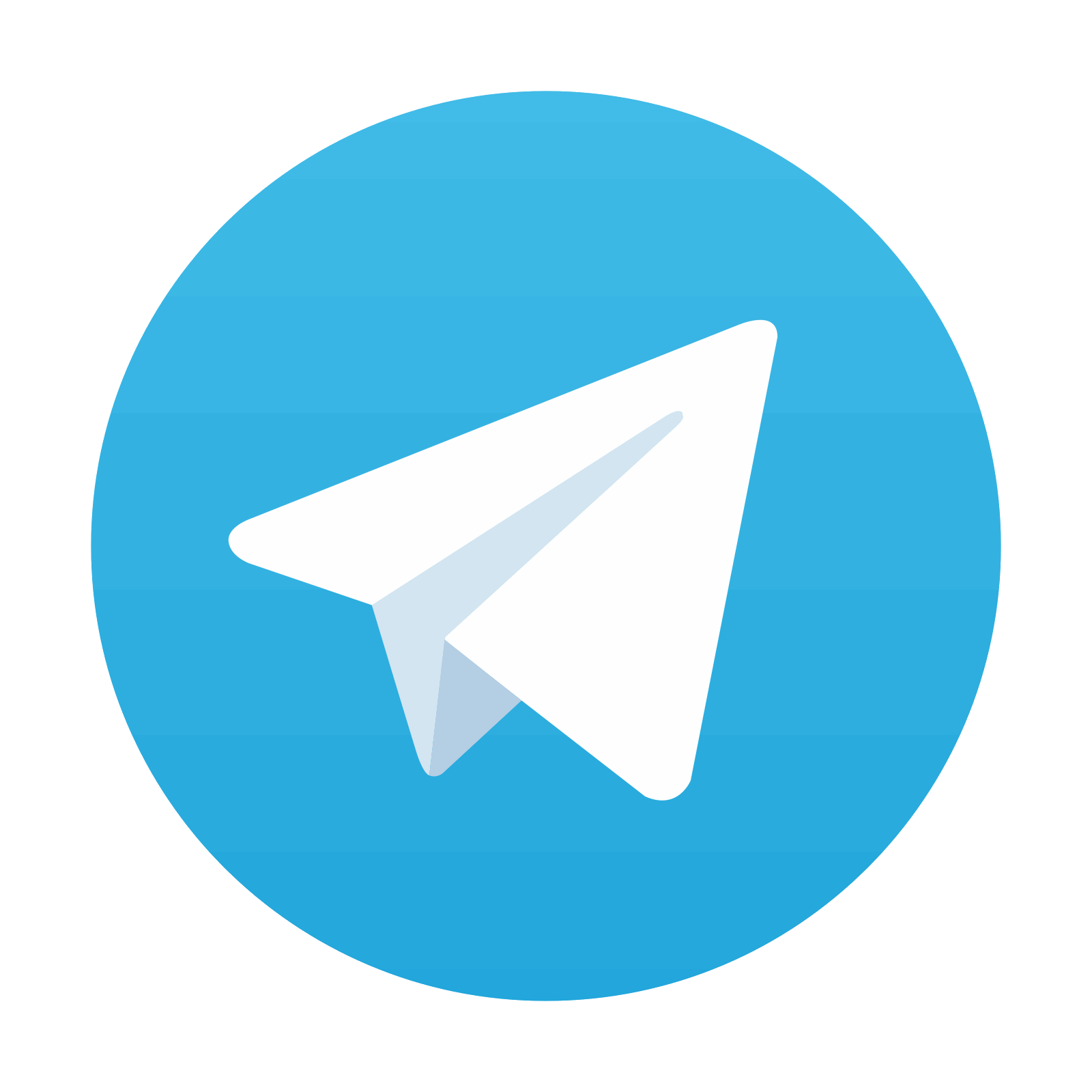
Stay updated, free dental videos. Join our Telegram channel

VIDEdental - Online dental courses
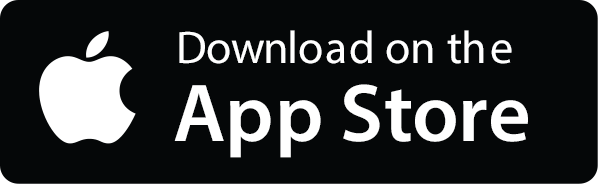
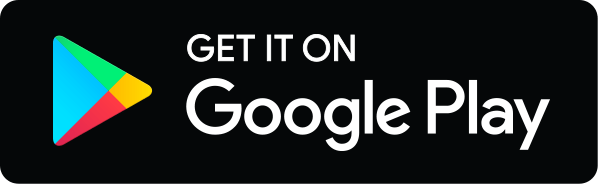
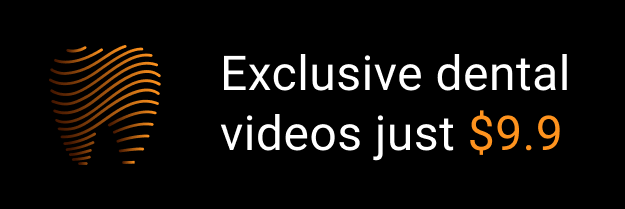