Dental caries is closely related to a dysbiosis of the microbial consortia of supragingival oral biofilms driven by a sugar-frequent and acidic-pH environment. The pH is a key factor affecting the homeostasis of supragingival biofilms seen in health. There is increasing interest on the ecological dynamics of the oral microbiome and how a dysbiotic microbiota can be successfully replaced by health-beneficial flora. The concept of preventing the microbial dysbiosis related to caries through modulation of sugar intake and pH has fully emerged.
Key points
- •
The pH and carbohydrate availability are key environmental factors affecting the physiology, ecology, and pathogenicity of oral biofilms colonizing teeth.
- •
Maintenance of oral health depends on the homeostasis of a consortium of commensal bacteria, such as that found in healthy-associated supragingival oral biofilms.
- •
Novel anticaries strategies include the modulation of the biofilm pH via arginine metabolism, nanotechnologies using acid-activated agents, oral probiotics and prebiotics, species-specific antimicrobial peptides targeting cariogenic pathogens, and other antibiofilm-specific approaches.
Introduction
After several decades of oral microbiology research, the scientific community has come to a simple conclusion – that a healthy mouth is a happy place to live if you are an oral commensal organism. In this context, the pH of supragingival oral biofilms is a key factor affecting the ecological “harmony” or homeostasis seen in oral heath. Oral commensals thrive at pH range between 6.0 and 7.0, which is the typical pH of resting biofilms colonizing healthy tooth surfaces. Advances in genome sequencing platforms, coupled with traditional microbiological studies, for analysis of the composition and function of the oral microbiome, have provided new insights in the etiology of oral diseses, and in particular, dental caries. Dental caries is closely related to a dysbiosis of the microbial consortia of supragingival biofilms driven by environmental changes, such as a sugar-frequent and acidic-pH environment. The concept of preventing the microbial dysbiosis related to caries through modulation of sugar intake and pH has fully emerged. Novel anti-caries strategies have been developed in recent years including modulation of the biofilm pH via arginine metabolism, nano technologies using acidic-triggered anti-caries agents, oral probiotics and prebiotics, species-specific antimicrobial peptides targeting cariogenic pathogens, and synergistic combination of bacterial killing and extra polysaccharides (EPS) matrix digestion. Yet, deeper understanding of the mechanisms of action and toxicity of these new technologies using in vivo models and further efficacy validation in clinical studies are required. This chapter summarizes our current knowledge of the healthy oral microbiome and these exciting new technologies being developed to promote dental health.
The oral microbial ecosystem plays an essential role in human health. This is because the oral cavity is a major portal of microbial entry to the human body, and the altered oral microbiota has been intimately associated with oral and systemic diseases. The potential link between oral diseases (eg, dental caries and severe periodontitis) ( Figs. 1 and 2 ) and systemic diseases (eg, cardiovascular disease, rheumatoid arthritis, and diabetes) has prompted great interest in the oral microbiome. There is also an evolving trend for dental and medical research to share knowledge on the etiology and pathogenicity of human diseases. Such interest follows insights provided from the Human Microbiome Project revealing that ecological balance in biofilms plays a significant role in health.


Nowadays, ecologists and data scientists are beginning to collaborate with clinical scientists, and this teamwork is crucial to understand the potential of microbiome-informed and microbiome-based medicine and why not microbiome-based dentistry? Improvements in the throughput and accuracy of DNA sequencing of the genomes of microbial communities of human samples, accompanied by analysis of transcriptomes, proteomes, metabolomes, and immunomes, and by functional experiments, have vastly improved the ability to understand the structure and function of the microbiome associated with disease and health. Our understanding of the link between the oral and the human microbiome is rapidly expanding. In the era of applied meta-omics and personalized medicine, the oral microbiome is a valuable asset. The oral microbiome encompasses the ecological community of commensal, symbiotic, and pathogenic microorganisms that share the mouth space. This community forms a dynamic and complex microbial ecosystem that usually exists in homeostasis or symbiotic state with the host, such as that found in healthy-associated supragingival oral biofilms.
The ecological complexity of the human mouth
From the time of birth, the human mouth is a primary point of entry of microorganisms into the human body. Microorganisms in the air food and those that are transferred through oral fluids (mainly saliva) from family members, caregivers, and others, can either just pass through the mouth or they can colonize the several oral niches. In fact, the complex ecosystem of the mouth supports the growth of diverse microbial communities comprising bacteria, viruses, mycoplasmas, archaea, fungi and protozoa that coinhabit and functionally interact in oral biofilms. The environmental factors that make the mouth such an attractive place for microorganisms include the: moisture and warmth (35°-37°C), presence of a variety of microbial niches, and abundance and continual influx of nutrients for microbial growth, such as salivary proteins, glycoproteins, and dietary components such as carbohydrates. As a result, the human mouth is home to over 800 bacterial species organized into microbial communities that occupy multiple niches, including saliva, dental plaque, gingival crevice, and various soft tissue surfaces that compose the buccal mucosa and the tongue.
The most abundant members of the oral microbiota are commensal organisms beneficial for oral health, but pathogens responsible for oral disease also exist. Commensal communities function to maintain the normal development of host tissues and defenses, by providing colonization resistance and down-regulation of damaging host inflammatory responses. However, the homeostasis or symbiotic relationship between the oral microbiome and the host is highly dynamic, as the composition and metabolic activities of microbial communities fluctuate according to the environmental changes in pH, nutrient availability, oxygen tension and redox environment, shedding effects of oral surfaces, and composition of salivary and crevicular fluids.
The pH and carbohydrate availability are key environmental factors affecting the physiology, ecology, and pathogenicity of the oral biofilms colonizing the teeth. Many beneficial oral bacteria can tolerate short periods of low pH, but their growth is inhibited by prolonged or frequent exposures to acidic conditions. In this context, the buffering activity of saliva plays a major role in maintaining the intraoral pH at around neutrality, which is optimal for the growth of most members of the oral microbiome. Changes in environmental pH occur following consumption of dietary sugar. Specifically, organic acids produced by the fermentation of dietary carbohydrates by cariogenic bacteria elicit demineralization of tooth enamel. These periods of acid challenge to the tooth are followed by periods of alkalization, which neutralizes plaque pH and promotes remineralization of tooth enamel. Whereas many factors contribute to the alkalization of oral biofilms (eg, buffers in saliva or diffusion of acids out of biofilms), alkali generation by oral bacteria plays a major role in pH homeostasis in oral biofilms and inhibits the initiation and progression of dental caries.
Oral microorganisms gain substantial advantages by growing as a biofilm, and by functioning as a microbial community. Biofilms are inherently more tolerant to environmental stresses, host defenses, and antimicrobial agents, compared with growth as single microbial cells. The complex spatial organization or biogeography of supragingival oral biofilms was demonstrated by spectral imaging fluorescence in situ hybridization (CLASI-FISH) and metagenomic sequence analysis. Corynebacterium was shown as the cornerstone of supragingival plaque architecture with long filaments that serve as anchor sites for many other microorganisms. This study also revealed that individual taxa are localized at the micron scale, in ways suggestive of their functional niche in the biofilm consortium. For example, anaerobic taxa tend to be in the interior, whereas facultative or obligate aerobes tend to be at the periphery of the consortium. Consumers and producers of certain metabolites such as lactate (the main acid dissolving tooth tissues) tend to be near each other.
The architecture of oral biofilms allows for synergetic and antagonistic interactions among different microbial species, and these interactions are central to homeostasis. Genome sequencing techniques have identified some of these oral microorganisms, but several others – about one-third of oral bacteria – are yet to be isolated from oral samples and cultivated in the laboratory by using conventional microbiological methods. Bacterial cultivation of certain strains may be challenging, because of their specific requirements for nutrients, while others may be inhibited by substances in the culture media, or produced by other bacteria. However, recent progress has been made on cultivating or cocultivating the so-called uncultivable bacteria, and greater knowledge about the ecological role of these “hard-to-grow bugs is being acquired.
The caries microbiome
The microbiome that naturally colonizes teeth in health is a biofilm community that can counterbalance acid production from dietary intake of carbohydrates to maintain an intact tooth surface (eg, by ammonia production from arginine or urea). As proposed by Marsh, excessive and frequent intake of carbohydrates exceeds the buffering capacity of the healthy microbiome and leads to dysbiosis of the biofilm with change of the bacterial composition ( Fig. 3 ). Current knowledge points to a symbiotic relationship between the oral microbiome and the host in health, contrasting with a dysbiosis of the microbial consortia driven by a sugar-frequent and acidic -pH environment in dental caries. In caries, continuous acid production from the metabolism of dietary carbohydrates favors the emergence of a highly acidogenic and aciduric microflora, enriched for Streptococcus mutans , other acid-tolerant streptococci, and Lactobacillus species. This selective process alters the pH homeostasis of supragingival biofilms and shifts the demineralization-remineralization equilibrium toward loss of tooth minerals.

Several studies support the observations that human supragingival dental plaque harbors a highly diverse bacterial community and that the microbiome of healthy tooth surfaces differs substantially from that found when there is evidence of caries activity. Changes in the microbial profile with the progressive stages of early childhood caries were observed when supragingival plaque of healthy tooth surfaces was compared with those of enamel and dentin carious lesions. In particular, plaque communities from dentin carious lesions of caries-active children showed a distinctive bacterial profile compared with the other communities. Moreover, communities from healthy tooth surfaces of caries-active children (CA-PF) were shown to be more similar to those from enamel carious lesions (CAE-PE and CA-PE) than to those of healthy teeth from caries-free children (CF-PF); suggesting that CA-PF sites appear to be at greater risk of caries development than CF-PF sites. This finding is concordant with previous risk factor studies for childhood caries and highlights the interconnectedness of plaque communities, in which these communities are part of a larger ecosystem where changes in the structure of 1 community may eventually affect others.
Modulation of biofilm pH
Carious lesions develop in tooth surfaces where there is an imbalance of the processes of acid and alkali production by supragingival biofilms. Because low pH is the main driving factor in the development of carious lesions, most efforts to identify an effective anticaries therapy have focused on targeting the acid-producing bacteria and their mechanisms of acid production. An expanding area of oral microbiology has been devoted to exploring microbial metabolic activities that help to neutralize biofilm pH, and thus inhibit the caries process. One way to restore biofilm homeostasis is to equilibrate the acidity and alkalinity processes in order to maintain a neutral biofilm pH. Physiologic factors that can counterbalance the acidification of biofilms include the clearance and buffering capacity of saliva and the metabolism of salivary substrates, such as urea and arginine, which generate alkali in the form of ammonia. In particular, ammonia production from arginine metabolism of oral bacteria inhibits tooth demineralization by neutralizing glycolytic acids and by favoring the growth of a desirable microflora that is compatible with dental health.
Arginine in caries prevention: prebiotics, probiotics, and oral care formulations
l -arginine was identified as the main component responsible for the pH-raising effect of saliva by early in vitro studies, and this amino acid is now receiving great attention because of its potential health benefits. Arginine is found free in saliva in micromolar concentrations, and is also abundant in salivary peptides and proteins. Arginine enters the mouth through dietary components but is also naturally produced by the human body via protein turnover and de novo arginine synthesis from citrulline. Multiple pathways for arginine degradation have been described in microorganisms, and occasionally several of them are simultaneously present in the same organism. Among these pathways, the arginine deiminase system (ADS) is the most widespread anaerobic route for arginine degradation.
In supragingival biofilms, arginine is metabolized mainly by ADS of certain oral bacteria to produce citrulline, ornithine, CO 2 , ATP, and ammonia ( Fig. 4 ). Ammonia production via ADS results in cytoplasmic and environment pH rises and serves as a mechanism used by oral bacteria for
- •
Protection against acid killing
- •
Bioenergetic advantages, including the increase of ΔpH and synthesis of ATP
- •
Maintaining a neutral environmental pH that favors the persistence of ADS-positive (ADS+) bacteria while being competitive against caries pathogens

The potential for arginine metabolism to prevent caries has been supported by compelling evidence from in vitro studies and clinical observations. Salivary levels of free arginine are strongly correlated with caries resistance. Plaque of caries-free individuals has higher pH values compared with plaque from caries-active individuals, and this difference has been correlated with elevated ammonia levels in caries-free subjects. The author’s clinical studies revealed a positive correlation between caries activity and low arginolytic capacity of the supragingival microbial populations of adults and children. In brief, the author and colleagues measured the ADS activity of plaque collected from caries-free tooth surfaces, enamel carious lesions, and dentin carious lesions of children who were either caries free or caries active. Mixed-model analysis evaluated if age, type of dentition, children’s caries status, and plaque caries status could be used as predictors of ADS activity. A recent cohort study revealed the arginolytic potential of supragingival plaque populations of children over time in the context of caries status. As observed in previous cross-sectional studies, plaque bacteria from caries-free children and caries-free tooth surfaces had consistently higher ADS activity compared with those from caries-active children and carious tooth surfaces during the 18 months of the study ( P <.0001).
Numerous therapies have been proposed to target specific caries pathogens or indiscriminately eliminate oral biofilms (eg, xylitol, chlorhexidine, immunization, and bacterial replacement therapy). However, the effectiveness of these methods is yet to be recognized, and safety concerns have been raised with regards to their negative impact in the ecology of the oral microbiota. Novel therapies that seek to provide arginine as a substrate for ammonia production in oral biofilms may have high cost-effective potential for at-risk populations facing challenges accessing dental care. These promising approaches may include the use of arginine as prebiotic, selected ADS + strains as probiotic, and/or introduction of arginine in oral care formulations.
A technology designed to deliver arginine for ammonia production by plaque bacteria has been incorporated into toothpastes, mints, and chews. Over the last 10 years, clinical trials have been conducted to evaluate the anticaries efficacy of products containing this original arginine technology or other optimized arginine formulations with or without fluoride. A toothpaste containing 1.5% arginine, an insoluble calcium compound, and 1450 ppm of fluoride was also shown to reduce caries increments in low- and moderate-risk children, to arrest and reverse carious lesions in children and adults, and to have superior caries benefits compared with a regular toothpaste containing 1450 ppm of fluoride alone. Thus, the mechanisms of action of arginine appear to complement those of fluoride by directly influencing biofilm pH while impacting the composition of oral biofilms. Even though the supplementation of arginine to oral biofilms can be effective against caries, the long-term impact of these novel arginine-based technologies on oral health and etiology of other oral diseases such as periodontal disease remains to be investigated.
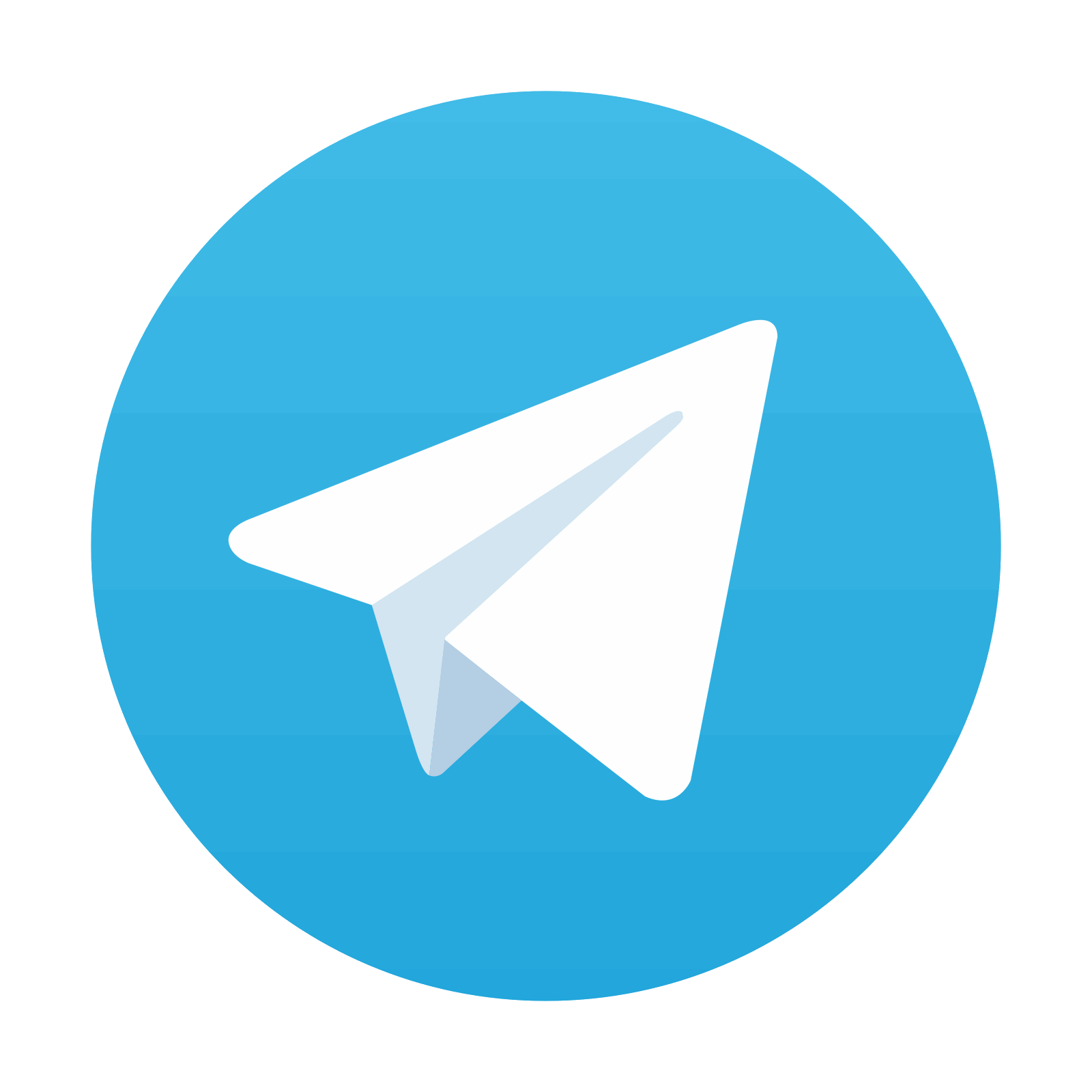
Stay updated, free dental videos. Join our Telegram channel

VIDEdental - Online dental courses
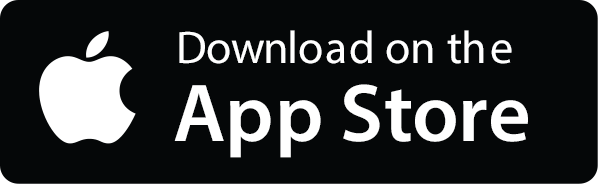
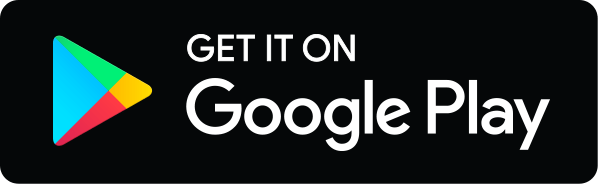