Abstract
Stem cell therapy for the treatment of bone defects is an alternative or adjunct to autologous bone grafting. This study assessed the efficacy of buccal fat pad-derived stem cells (BFPSCs) with iliac bone block grafting for the treatment of extensive human alveolar ridge defects. Eight patients with extensive jaw atrophy were selected for this study. The jaws were reconstructed with non-vascularized anterior iliac crest bone blocks. Gaps between the blocks were filled with freeze-dried bone granules and covered with a collagen membrane. In the test group ( n = 4), these granules were seeded with BFPSCs. Cone beam computed tomography scans were used to assess the amount of new bone formed at six sites in each patient. Trephine biopsies of 2-mm were also taken from the graft site during implant placement for histomorphometric analysis. The mean bone width change at the graft site was greater in the test group than in the control group (3.94 ± 1.62 mm vs. 3.01 ± 0.89 mm). New bone formation was 65.32% in the test group versus 49.21% in the control group. The application of BFPSCs in conjunction with iliac bone block grafts may increase the amount of new bone formation and decrease secondary bone resorption in extensively atrophic jaws.
The reconstruction of severely resorbed jaws is a challenging procedure, depending on the severity of bone atrophy. Bone defects in the maxillofacial region vary from small peri-implant defects to extensive bone atrophy (approximately equal to a span of six teeth). For extensive defects, autogenous bone grafting from an extraoral donor site, such as the anterior iliac crest, remains the workhorse technique for jaw reconstruction. Autografts concurrently provide osteogenic cells and a suitable scaffold for osteogenesis. However, donor site morbidity, prolonged hospitalization, and high costs are all limitations of this technique. Moreover, secondary bone resorption is a major shortcoming for dental implant success. It has been reported that the entire grafted iliac crest bone may be resorbed within 6 years post-surgery.
Tissue engineering is a new minimally invasive method to restore and reconstruct tissues or organs that involves the morphogenesis of new tissue using constructs formed from isolated cells, growth factors, and scaffolds. Mesenchymal stem cells (MSCs) form part of the tissue engineering triangle and are a novel modality for bone autografts. These cells may be obtained from several sources including the bone marrow, adipose tissue, dental pulp, peripheral blood, or periosteum. In the trial reported here, the buccal fat pad was used as a source of MSCs. Adipose stem cells (ASCs) – initially called processed lipoaspirate cells – were first discovered in 2001 by Zuk et al. They have been evaluated extensively for tissue engineering, and in particular for bone formation. Many studies have shown the excellent efficacy of MSCs in bone regeneration, but in larger and critical-sized defects, this stem cell treatment as the sole therapy has shown a lesser amount of new bone formation. Furthermore, onlay block grafts have shown reduced osteogenic activity and slow revascularization when compared to particulate bone marrow.
To increase the osteogenic activity of non-vascularized iliac bone block grafts, this study sought to assess the concomitant application of buccal fat pad (BFP)-derived MSCs (BFPSCs) and anterior iliac crest bone grafting in the reconstruction of extensive alveolar ridge defects.
Materials and methods
Patient selection
Eight patients presenting to the maxillofacial clinic of a university hospital in Tehran, Iran, were selected for this study. All of the patients enrolled in this study had extensive jaw atrophy (the need for augmentation to accommodate more than six teeth). The patients were aged between 25 and 60 years. They were non-smokers with no underlying systemic conditions. Of these eight patients, four were assigned to the control group. In these cases, reconstruction of the atrophic ridge was performed using onlay bone block grafting from the anterior iliac crest. Freeze-dried bone allograft (FDBA) granules (SureOss; Hans GBR Biomaterial, Seoul, Korea) were applied to fill the gaps, and collagen membranes (Jason membrane; Botiss Biomaterials GmbH, Zossen, Germany) were used to cover the defect. In the test group ( n = 4), the FDBA granules were loaded with autogenous MSCs harvested from the BFP.
The institutional ethics committee approved all of the studies, and informed consent was obtained from all donors. The isolation and cultivation of the MSCs was performed without xenogenic supplements such as foetal bovine serum (FBS). All methods used to cultivate and extract MSCs have been described in previous projects using human patients.
Buccal fat pad-derived stem cells (BFPSCs)
The BFP was harvested from healthy donors through a vestibular incision distal to the maxillary second molar ( Fig. 1 ). It was exposed by blunt dissection while preserving the thin covering membrane. Approximately 3–5 ml was excised and delivered to the laboratory in Dulbecco’s modified Eagle’s medium (DMEM). The dissected tissue was minced and incubated in 3 mg/ml type I collagenase and 4 mg/ml dispase in phosphate buffered saline (PBS) (Gibco Laboratories, Grand Island, NY, USA) at 37 °C for 30 min. The fragments were then immersed in minimum essential medium alpha (α-MEM) supplemented with 10% FBS and 1% antibiotics (Gibco), and placed in 25-cm 2 culture flasks, followed by incubation in a humidified atmosphere containing 5% CO 2 at 37 °C. The culture medium was changed twice weekly and after reaching the subconfluent stage, and cells were removed by enzymatic digestion (0.25% trypsin–ethylenediaminetetraacetic acid (EDTA)) and passaged. MSCs of the third to fourth passage were used in the experiments. Adherent cells were expanded as monolayer cultures in a 95/5 air/CO 2 (v/v) atmosphere at 37 °C, with medium changes every 3 days. Confluent cells were dissociated with trypsin and subcultured in new six-well culture dishes at a plating density of 6 × 10 4 cells/dish. The cells could be seen under light microscopy ( Fig. 2 ).


Preparation of human serum
Human autologous serum was used in this study. Twenty millilitres of whole blood was collected from each patient and drained into two 10-ml tubes without anticoagulants (BD, Plymouth, UK); this was allowed to clot for 4 h at 4–8 °C. Subsequently, the blood was centrifuged at 2800 × g at 4 °C for 15 min. Serum was collected and stored at −20 °C. FBS was omitted from the laboratory procedure for ethics committee considerations.
Evaluation of the mesenchymal stem cell nature of the isolated cells
Flow cytometry analysis
Fluorescence absorbance cell sorting (FACS) analysis was performed using standard protocols and quantification criteria to confirm the cell surface expression of CD44, CD90, CD73, CD105, CD45, and CD34. The gate used to distinguish positive from negative cells was set individually for each marker. Fluorescent isothiocyanate (FITC)-conjugated monoclonal antibodies and phycoerythrin (PE)-conjugated monoclonal antibodies were applied to the isolated cells using the flow cytometry device (BD FACSCalibur; BD Biosciences, Franklin Lakes, NJ, USA). Anti-CD44-FITC, anti-CD90-FITC, anti-CD73-PE, anti-CD105-PE, anti-CD45-FITC, and anti-CD34-PE (EXBIO Praha, a.s., Vestec, Czech Republic) were used at 2 μg/ml. Cells stained with FITC-labelled mouse immunoglobulin G (IgG) were used as negative controls. After washing twice with PBS, the cells were fixed with 1% paraformaldehyde. Flow cytometry standard files were analysed using FlowJo 7.6.1 software (FlowJo LLC, Ashland, OR, USA). Specimens containing at least 90% fluorescent-labelled cells were regarded as positive.
In vitro osteogenic differentiation
Cells from the third passage were placed in DMEM supplemented with 50 mg/ml ascorbic acid 2-phosphate (Sigma Aldrich, St. Louis, MO, USA), 10 nM dexamethasone (Sigma Aldrich), and 10 mM β-glycerol phosphate (Sigma Aldrich). After 3 weeks, the differentiated cells were fixed for 1 h in 4% formalin and rinsed with PBS (Gibco). Mineralization of the extracellular matrix was visualized by staining with 40 mM Alizarin Red S, pH 4.2, for 5 min.
Scaffolds and cell seeding
SureOss (Hans GBR Biomaterial) is a freeze-dried cortical bone allograft (FDBA). It is a granular biomaterial with a particle size of 200–850 μm. Approximately 5 mg of this biomaterial scaffold was placed into 96-well plates. BFPSCs were seeded onto the scaffolds at a cell density of 10 5 cells/well in osteogenic medium (Invitrogen, Grand Island, NY, USA). The cell scaffolds were maintained at 37 °C in a humidified 5% CO 2 atmosphere for 7 days.
Cell adhesion
BFPSC adhesion to the scaffolds was assessed using a scanning electron microscope. Three days after cell seeding, the samples were fixed in 2.5% glutaraldehyde solution (Merck KGaA, Darmstadt, Germany) for 30 min. The specimens were then fixed in 1% osmium tetroxide (Sigma Aldrich). After cell fixation, the specimens were dehydrated in an ascending series of ethanol concentrations (25%, 50%, 75%, 96%, and 100%) for 15 min each step. The specimens were then allowed to dry in air. After complete drying, they were sputtered with gold and analyzed using a scanning electron microscope (Hitachi, Tokyo, Japan).
Surgical procedure
A vestibular incision was made molar-to-molar, 10 mm away from the maxillary crest area, without any releasing incision. A buccal and lingual mucosal flap was elevated with gentle sub-periosteal dissection. Concurrently, a monocortical, autogenous bone graft was harvested from the medial surface of the anterior iliac crest. The iliac crest cortical block was cut into two or more pieces according to the characteristics of the recipient site. Adequate fixation was achieved with 1.2-mm microscrews. In the test group, FDBA loaded with BFPSCs was transferred to fill the gaps. In the control group, FDBA alone was used to fill the gaps ( Fig. 3 ). Concomitant injection of cefazolin (1 g) was performed during the perioperative period, as well as a 3-day course postoperatively.

Clinical evaluation
Soft tissue healing and the normal healing sequence of the grafted tissue were assessed every 2 weeks.
Radiographic assessment
Radiographic stents were made for all patients. Cone beam computed tomography (CBCT) images were obtained twice, once before bone grafting and once before dental implant placement. All patients wore their radiographic stent for the radiographic evaluations. The bone width was measured in six edentulous areas (10 mm apart) from anterior to posterior (A, B, C, D, E, and F) and at three levels (1, 3, and 6 mm depth) from the atrophic ridge crest (BW1). Thus, 72 sites were evaluated (6 × 3 × 4) in the test group and 72 sites in the control group. Five months after the surgical procedure and before dental implant placement, the second CBCT was obtained with the radiographic stent in place, in the same area. The bone width at the respective locations was measured (BW2). The changes in bone width values (BW2 − BW1) were analyzed ( Fig. 4 ).

Histological analysis
During implant placement, trephine biopsy samples of 2-mm in size were harvested from the gaps between the anterior iliac crest blocks that were filled with the granules ( Fig. 5 ). The specimens were fixed in 10% buffered formalin for 7 days and decalcified in formic acid and sodium citrate for 24 h. The specimens were washed with tap water, dehydrated with ascending concentrations of ethanol, cleared in xylene (Sigma Aldrich), and infiltrated with paraffin. Parallel sections (7 μm) from the central part of the specimens were cut using a microtome and stained with haematoxylin and eosin (H&E).

New bone formation, scaffold remnants, and inflammatory reactions were analyzed by an examiner using a light microscope (Olympus SZX9; Olympus, Tokyo, Japan). The percentage of new bone formation was analyzed using computerized image analysis software (Image-Pro Plus; Media Cybernetics, Silver Spring, MD, USA).
Data analysis
Data were analyzed using IBM SPSS Statistics version 20.0 (IBM Corp., Armonk, NY, USA). Descriptive statistics including tables and graphs were used for data description. The Mann–Whitney U -test was applied for analysis. P -values of <0.05 were considered to be statistically significant.
Results
In vitro differentiation
Flow cytometry
In order to confirm whether the isolated/cultured BFPSCs displayed the MSC phenotype, cells were subjected to flow cytometry analysis for the expression of positive (CD44, CD90, CD73, and CD105) and negative (CD45 and CD34) MSC markers. The results showed that more than 95% of this cell population were positive for CD44, CD90, CD73, and CD105 (the MSC surface markers), whereas they were negative for CD45 and CD34 (hematopoietic markers) ( Fig. 6 ).
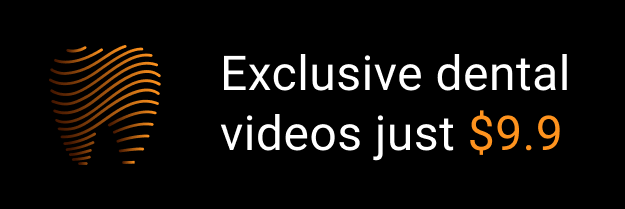