MORA appliance. MORA (mandibular orthopedic repositioning appliance) appliance with hard acrylic covering the posterior teeth designed to reposition the mandible in the anterior direction
Consequently, in order to assess differing impacts of vertical dimensions and subsequent effects on performance, researchers employed various methods to measure impact on performance. Greenberg and colleagues conducted a study by creating a placebo appliance (no vertical dimension) versus a MORA/Gelb device, testing strength differences in a university basketball team (N = 14). The authors were not clear on how much vertical dimension the appliance provided but did cite that the placebo appliance did not provide any resin on the occlusal services, resulting in no change in vertical dimension for this appliance. In testing shoulder abduction and adduction on the dynamometer, there were no differences between the placebo appliance, the no appliance, and the MORA device [33, 34]. However, the sample size was small, no vertical dimensions were reported, and the authors noted that these athletes were not involved in any strengthening program at the time of testing. Therefore, in order to address some of these issues, Yates and colleagues set the vertical dimensions to a specific 2–3 mm for 14 college football player subjects studying the effect of the MORA on isokinetic and isokinetic upright rower, isometric deadlift, or isometric two-arm pull. They too found no significant positive or negative outcomes in muscular strength with this position at 2–3 mm vertical dimension [28]. However, more recently, Lee et al. [35] utilized a MORA device which was designed using a precise protocol (see paper for all aspects of the protocol) that provided 3 mm vertical dimension at the centric occlusion and adjusted such that all teeth would come in contact evenly with the MORA device [35]. Their findings revealed significant EMG outcomes with isometric improvements in the following muscle groups: sternocleidomastoid muscles, cervical and lumbar erector spinae, upper trapezius, biceps, triceps, rectus abdominis, and internal and external oblique [35].
It is evident in many of the earlier studies that a few methodological issues were apparent as it relates to the vertical dimensions, sample size, types of athletes and sports studied, and design of the mouthguards. However, despite these issues, each study added valuable knowledge to the understanding of the effect of MORA and/or mouthguard use during exercise performance. Yet the question still remained on how the MORA and/or mouthguard use during exercise performance elicited performance outcomes, if any. Were any positive performance outcomes due to the placebo effect, or was there a link to whole-body physiology with the use of such a device as described by Stenger and Kaufman [23, 27]? Thus, due to the complexities of the issues, the difficulties involved for practicing dental professionals in conducting research, and the lack of interest by researchers in the field of exercise physiology, investigation of this issue remained stagnant for several years.
13.2.2 Recent Research on the Effect of Mouthguard Use on Performance

Performance-enhancing mouthguards. Two styles of performance mouthguards Under Armour MG that relies on wedges to move the mandible in an anterior inferior direction. The pure power MG utilizes neuromuscular dentistry to locate the desired bite to build into the appliance
13.2.3 Mouthguard Effect on Anaerobic Performance
There have been a number of current studies published which cite improvements with mouthguard use during anaerobic performance which includes varying mouthguards and methodology [37–41]. Ebben and colleagues using a vinyl mouthpiece found significant improvements during knee extensions, with an 11% increased average torque and 10% increase in peak torque with subjects clenching on a mouthpiece versus no-mouthpiece condition [40]. Dunn-Lewis and colleagues also cited improvements with a Pure Balance mouthguard made of an EVA material which incorporates pronounced bite pads on both sides of the maxillary mouthguard. Among their findings, they cited a significant increase in bench throw power and force, increased rate of power production in the vertical jump for the Pure Balance mouthguard versus no mouthguard and an over-the-counter mouthguard. Yet no differences were seen in the Pure Balance mouthguard between conditions as it related to 10 m sprint time, sit and reach distance, a visual reaction test, and balance [38]. Utilizing a TMJ repositioning mouthguard, Arnet and colleagues gauged the effect on physical performance parameters in collegiate and professional athletes using neuromuscular dentistry (a method in which TENS surface electromyography is applied to the jaw to facilitate muscular relaxation resulting in a neuromuscular optimal bite position, with a mouthguard fabricated based on this position). They found that when subjects wore a TMJ repositioning device, there was a 3% improvement in vertical jump and average mean power for the Wingate anaerobic test versus a standard custom-fit mouthpiece designed to protect the teeth [37]. These findings as it relates to the Wingate protocol were later substantiated using a maxillary mouthguard (CleverBite®, CleverBite SL, Terrassa, Spain) fabricated using digital scans of the maxillary and mandibular dental arches as well as a recording of the interocclusal relation of the rest position of the mandible. they found a 4% improvement in peak power and a 1% improvement with mean power during the Wingate anaerobic test in the mouthguard condition, these being the same findings (4% and 1%, respectively) by Arnet and colleagues using the same protocol [37, 41]. In addition, researchers cited an 8% improvement in lactate measures with the mouthguard use and significant improvements in all variables associated with anaerobic testing with mouthguard use compared to no-mouthguard condition [41]. Durante-Pereira and colleagues also cited significant improvements with a maxillary custom mouthguard (described as pressure laminated with gum shield consisting of ethylene vinyl acetate) in testing countermovement jumps (CMJ) in 10 rugby players. Yet they found no differences in a 15 second rebound jump nor in the spirometer data with each of these tests. Yet, the improvement in the CMJ test should be viewed with caution due to the small sample size [39]. Using the same CMJ test, Busca and colleagues utilized a larger sample size (N = 28) and measured vertical CMJ and found significant improvements in mean power and height in the mouthguard condition versus a clenching no-mouthguard condition and a no-clenching no-mouthguard condition. The mouthguard used in this study was the CleverBite (as described earlier in this review) which relies on digital scans of the maxilla and mandible with a resultant maxillary mouthguard of 1.4 mm EVA overlaid with 4 mm polyethylenterephthalat-1. In addition, they cited significant improvements during the hand-grip test and the back-row isometric force test (force development and peak force) in the mouthguard condition versus the other two conditions (clenching no mouthguard and no clenching no mouthguard) [42].
While there are many studies which have cited improvements in performance with mouthguard use during exercise, there are numerous others which have cited no difference in anaerobic performance with mouthguard use. A study by Allen and colleagues examined recreationally trained individuals and found no differences in countermovement vertical jump (CMJ) using a force plate and Vertec device, one-repetition bench press, and measurements of peak force or rate of force development. However, the caveat of this study was that the authors admitted they had not informed the subjects to clench or not to clench but to try to perform each exercise as “normally as possible” [43]. Thus, the difference between those articles finding improvements with mouthpiece use and those which did not cite differences may be due to the clenching effect. Goelm and Arent also found no differences with a maxillary mouthpiece in assessing measures of vertical jump and power output, balance, flexibility, range of motion, strength (though trended to significance with p = 0.06), and agility. They cited that this lack of evidence in any of these parameters may be due to the fact that they used a basic custom mouthpiece that may not have provided optimal jaw-repositioning versus a custom dental appliance used in a previous study done in their lab which found differences using a jaw-repositioning mouthpiece [37, 44]. Drum and colleagues also noted no differences in anaerobic, aerobic, reaction time, and flexibility measures between a custom-fit maxillary mouthguard, boil and bite mouthguard, and a no-mouthguard condition. Their study utilized a collegiate football team, highly conditioned athletes, yet sample size (N = 10) was small and would be an impetus for more studies with a larger sample size [45].
13.2.4 Oxygen Uptake and Ventilation
While much of the research has focused on anaerobic performance outcomes with mouthguard use, other research has focused on objective measures assessing differences in oxygen uptake , heart rate, and ventilation and mouthguard use [46–55]. In a study examining impact of exercise between no-mouthguard condition, commercially available maxillary mouthguard, and custom maxillary mouthguard in 19 trained males, Bourdin and colleagues cited no differences in respiratory parameters as well as no differences in visual reaction time and explosive power at rest and during exercise [47]. Yet interestingly, as it relates to respiratory parameters, the commercially available mouthguard showed differences in respiratory rate during stages of incremental exercise on the cycle ergometer. the use of this mouthguard resulted in a 9% decrease in respiratory rate during stage 1 of the incremental protocol using the commercially available mouthguard as compared to the no-mouthguard condition. These appear important in light of later research by Garner and colleagues which found significantly lowered respiratory rates with various mouthpieces utilized in their studies [47–50]. Gebauer and colleagues also found no differences in respiratory function (ventilation, oxygen uptake, and heart rate) during a graded exercise test (two 5 min stages at 6.2 and 7.5 mph) between no mouthguard, normal-palate maxillary mouthguard, and an open-palate maxillary mouthguard, with N = 27 [53]. However, they cited a 3.9% change between no-mouthguard condition and palate-free condition and a 2.1% change between no mouthguard and normal-palate mouthguard in comparing maximum oxygen uptake (ml/kg/min) during each stage [53]. During an incremental exercise protocol in which the workload was increased each minute by 30 W, von Arx and colleagues showed that subjects experienced no difference between no mouthguard and custom mouthguard as it relates to peak oxygen uptake, breathing frequency, and peak minute ventilation. However, von Arx and colleagues did note a 5% improvement in workload scores with the mouthguard versus no mouthguard [55]. In another graded exercise protocol in which subjects were asked to cycle for 5 min during four stages which increased by 50 W for each stage, Bailey and colleagues cited a significant difference in ventilation in the vented moldable maxillary mouthguard versus the no mouthguard and standard boil and bite maxillary mouthguard. Specifically, the vented mouthguard ventilation was 9% lowered at maximum workload as compared to the no-mouthguard condition and was 6% lower at 200 W in vented versus no mouthguard. In addition, they cited a significant reduction in blood lactate levels with the vented mouthguard as compared to the no mouthguard and standard boil and bite mouthguard at both the 200 W and maximum workloads [46]. Finally, while most studies cited involve males, Rapisura and colleagues using an all-female population (N = 11) cited no significant differences in heart rate, oxygen consumption, and minute ventilation between women with a self-adapted mandibular mouthguard, boil and bite maxillary mouthguard, and no-mouthguard condition during 2 min incremental exercise on the cycle ergometer [54]. Yet issues with this study were small sample size and potential differences in the use of the maxillary versus mandibular mouthguard as well as inability to compare respiratory parameters due to the duration of incremental exercise chosen for this study (2 min stages). While these studies have utilized various sample sizes and protocols, what appears to be apparent is that a trend or a significant difference occurs with respiratory parameters with mouthguard use during higher-intensity exercise. Yet why would such changes in respiratory parameters during exercise be important to individuals during exercise?
An earlier study by Francis and Brasher helps shed light on possible mechanisms and impact on exercise performance with mouthguard use [56]. In this study, they had subjects perform 20 min of continuous exercise with varying intensities with the following conditions: no mouthguard (No), unfitted upper mouthguard (MG1), unfitted bimaxillary mouthguard (MG2), and a bimaxillary guard with a breathing hole (MG3). In comparing all conditions for the subjects with conditions randomly assigned, they found that during heavy-intensity exercise, subjects had significantly lower ventilation with the mouthguard conditions as compared to the no-mouthguard condition, with expired volume of gas being higher in the mouthguard condition. They then concluded that the use of the mouthguard may actually result in an improved breathing pattern that would enhance alveolar ventilation. They cited that this could be due to a type of pursed-lip breathing that would enable subjects to take in less air with a given amount of oxygen thereby affecting ventilation and expired gas [56]. In their protocol they examined effects during both light and maximum exercise on a cycle ergometer, with only the maximum exercise demonstrating differences. Similar to this protocol, Garner and colleagues utilized mandibular mouthpieces against a no-mouthpiece condition in assessing effects on respiratory parameters [48–50]. In one study, they found significant decreases in respiratory rate with both the boil and bite and the custom mouthpiece, specifically a 3% reduction in respiratory rate during the first 5 min of moderate-intensity activity [49, 50]. Then in comparing the boil and bite to the custom, the boil and bite had lowered respiratory rate versus the custom, with a 9% reduction in the respiratory rate with the mandibular boil and bite mouthpiece [48–50]. As noted earlier, comparing these outcomes is difficult to relate to other studies as none have provided steady-state exercise parameters. However, the study by Bourdin and colleagues was the most similar with an incremental exercise protocol of 4 min. Bourdin and colleagues found a 2% difference in ventilation (L/min) with the custom upper mouthpiece versus the no-mouthguard condition during the first 4 min of the exercise, with Garner finding 1.4% difference in ventilation between their custom lower mouthpiece and the no-mouthpiece condition during the first 5 minutes of steady-state exercise [49, 50]. In addition, Bourdin and colleagues found a 5% difference in breaths per minute between the boil and bite mouthguard and no-mouthguard condition, while Garner found a 5% decrease in respiratory rate (similar to breaths per minute) between custom mouthpiece and no mouthpiece and a 9.7% decrease in respiratory rate between a boil and bite and the no-mouthpiece condition during 10 min of steady-state exercise [47–50]. Although the protocols are different, cycling versus running and steady state versus graded, they are the most similar and enable a greater understanding the effect of various mouthguards/mouthpieces versus a no-mouthguard/no-mouthpiece condition. A potential explanation on the differences may be due to the amount of material with boil and bite mouthguards/mouthpieces versus the custom devices as it relates to the tongue placement (to be discussed in a later section). Thus in these studies, the objective measures of respiratory function demonstrated trending or significant improvements in ventilation and or breathing rate with mouthguard use during maximal exercise. This is critical as it shows that breathing rate and ventilation is slower while taking in the required oxygen needed for exercise with mouthguard use, potentially resulting in improved alveolar ventilation and reduced workload as seen in the study by Bailey and von Arx studies [46, 55, 56].
13.2.5 Lactate and Cortisol

CBCT scans airway. CBCT scans of subject airways with and without oral appliance. Appliance used was Under Armour mouthpiece (lower arch) seen in ◘ Fig. 13.4. a shows the no appliance airway and b shows the airway while wearing the appliance. There was consistent significant improvement in airway volume while wearing the appliances
In conclusion, although these recent studies have cited improvements, of importance is understanding if there is an impact of the placebo effect with any of these studies, i.e., if a person is told he/she will or won’t improve performance outcomes with an appliance, this is likely to affect results. It is unclear whether researchers informed subjects of a potential effect in studies that found improvements. In studies within the Garner laboratory, subjects were not told whether the mouthpiece would or would not affect their performance [59]. However, due to the popularity of such products, it still may be a factor in influencing subjects. Thus, research which is less subjective is needed to support or refute the positive physiological findings with mouthpiece use. Much of the evidence citing positive physiological performance effects leads this author to believe that there is a physiological mechanism resulting from mouthguard use during exercise. Thus, the next sections of this review will delve into these theories of the mouthguard effect which have been substantiated in other fields of research, thus providing a greater understanding and knowledge of how to better study this area of sport dentistry.
13.3 Literature Review of Theories to Support Performance Enhancement
13.3.1 Genioglossus and Tongue Position
It has been cited that the “the tongue is a small member and has dominion ” (James 3:5, Aramaic Bible in Plain English). Although this statement was not in reference to the tongue being a physiologic marvel, it cannot be overstated the importance and involvement of the tongue within several physiologic functions. The complexity of the organ can be found in studying its involvement in respiration, swallowing, speech, and mastication [66–72]. The tongue muscle, specifically the genioglossus, is the main protruding muscle. The genioglossus is innervated by the hypoglossal (cranial nerve XII) which, along with the hypoglossus, causes a pressing down of the tongue base [66, 69]. The importance of the genioglossus is its role in increasing muscular tone during the inspiratory phase of breathing [73, 74] which in turn is important for dilating of the pharyngeal area. The importance of the genioglossus’ role in dilating the airway has been extensively researched in the area of sleep apnea and will be discussed later in this chapter [75–79].
In addition to the genioglossus’s role in dilating the airway, there has been a body of literature which states the tongue’s role in temporomandibular (TMJ) disorders , serving as a way to mediate or reduce the severity of the disorder. Schmidt and colleagues cite the use of the tongue as a treatment option as minimizing muscle activity and thereby reducing pain in the orofacial area; specifically by placing the tongue in a position of “rest” will maximize relaxation and subsequently reduce muscle-related pain in the TMJ [80]. Optimal tongue placement providing a “rest” position suggests that it should be positioned on the floor of the mouth. Evidence of this optimal tongue “rest” position cites decreased EMG activity in the right masseter, suprahyoid, right temporalis, and left temporalis with the tongue on the floor of the mouth versus against the hard palate [80]. To a degree, others have supported this, finding decreased activity in the anterior temporalis and suprahyoid with the tongue on the floor of the mouth versus on the hard palate yet with an increase in masseter EMG activity with the tongue on the floor versus the hard palate [81]. Yet, before the research of how the genioglossus elicits effects in the body, there must be an understanding of mechanisms involved with innervating the tongue muscle and its reflex response.
Miller [69] states that the tongue, in order to operate optimally, receives complex somatosensory input via the central nervous system, resulting in both complex and simple reflex actions [69]. Initial animal and human research to more recent research supports this hypothesis [69, 82–87]. With the animal model, Lowe and Sessle cite the interaction between the jaw and tongue when they opened the cat jaw as little as 4 mm, resulting in genioglossus activity. This outcome led to their conclusion that the temporomandibular joint significantly affects the activity of the tongue due to reflexes originating in orofacial regions [88]. In earlier human research, Weber and Smith [87] stated a reflex exists between the jaw, tongue, and lip by demonstrating increased EMG activity of the masseter, orbicularis oris inferior, and the genioglossus with mechanical stimulation [87]. In the human model, Takata and colleagues found genioglossus and orbicularis oris EMG activity increased with jaw opening and ceased with jaw closing during gum chewing, suggesting the link between the tongue, lip, and jaw [89]. Hiyama’s laboratory also found similar outcomes with EMG activity of the genioglossus, with EMG increasing during jaw opening. Yet, they also found increases during jaw closing. They state that this may be due to the hypoglossal nerve which innervates the tongue protruding muscle, thus resulting in EMG activity in both the opening and closing phases of the jaw. They hypothesize that this collaboration of activity between the jaw and tongue would not be explained by a sequential reflex response but possibly preset into the central nervous system within the lower brain stem [67]. Miller [69] explains these complex oral reflexes as it relates to the genioglossus, stating that either via the lingual nerve or mechanical stimulation of the tongue will result in a potential excitation or inhibition in various tongue muscles [69]. In addition, Miller cites that this interaction between the tongue and jaw plays a critical role in the function of the pharyngeal pathway during respiration; specifically that protrusion of the tongue muscle will function to open in the pharyngeal airway [69].
The importance of tongue muscle placement has been cited as playing a key role in the opening of the pharyngeal area, with sleep apneic studies citing a forward shift of the mandible and subsequent forward protrusion of the tongue using sleep apneic mouthpieces designed to promote enhanced breathing mechanics [75, 76, 90, 91]. these devices have been shown to increase the pharyngeal area, with Kyung and colleagues citing a 19% improvement in cross-sectional area of the retroglossal (defined as the back of the tongue to the wall of the pharynx) area of the pharynx using a 75% mandibular advancement mouthpiece [91]. Mann and colleagues cited increases in the diameter of the hypopharyngeal area with genioglossal stimulation, resulting in a mean 133% increase from baseline [68]. Earlier research cited that contracting the genioglossus results in pulling the base of the tongue down and forward, with later researcher citing that this occurs with the help of the protruder muscles, which will subsequently open the pharyngeal area [92, 93]. To clarify how this occurs, Saboisky et al. [71] cited a complexity of networks linking the hypoglossal motoneurons which innervate the genioglossus. they cite increased genioglossus discharge rates during both inspiration and expiration thereby leading to tongue protrusion [71]. In addition, research has shown that the number of hypoglossal motoneurons will also be affected by exercise, citing an increased number of these motoneurons activated with increased exercise intensity, resulting in increased EMG activity of the genioglossus [94].
13.3.2 Role of Clenching
In addition to the important role the genioglossus plays in dilating the airway as innervated by the hypoglossal motoneurons, research has also examined the effect that clenching has on the EMG activity of this muscle. Firstly, researchers have cited an increase in EMG activity in the genioglossus with mild to maximal clenching during non-exercise protocols [86, 95]. Valdés and colleagues cite a link between the masseter while clenching and its effect on the tongue, noting the interaction using 30 healthy subjects with no current or past pain in the TMJ, mouth, or tongue. In measuring the EMG activity of the masseter and temporalis during clenching and swallowing, they cited significantly lower EMG activity in the masseter during clenching with the tongue on the floor of the mouth versus on the hard palate, this being explained by the effect the tongue creates when placed on the floor of the mouth, against the mandibular, lingual side of the incisors, which consequently linked to the masticatory muscles [86]. Igarashi then demonstrated outcomes in the genioglossus during clenching, finding an increase in EMG activity of the genioglossus during clenching [96]. Finally, as it relates to force production, placement of the tongue has also been cited as an important factor. Saboisky et al. [97] found that optimal position for the tongue resulting in the greatest tongue force production is when it is retracted between 12 and 32 mm, with the mean maximal force being 28.3 N at 24 mm and the lowest forces (14.9 N) produced with tongue protrusion at 12 mm [97]. Not only did they find increased force production but also cited in a significant decrease in breathing rate with the tongue on the floor of the mouth (15.47 BPM) versus the tongue on the roof of the mouth (16.15 BPM, p = 0.023). These findings support observational studies in our laboratory with various mouthguard/mouthpieces utilized during exercise (versus at rest as in the Saboisky et al. study) and will be discussed later in this chapter.
Not only has clenching been cited to effect genioglossus activity and masticatory muscles, clenching has also been shown to affect cerebral activity in activation of the cortical areas in the brain, thereby affecting the hormone response [60, 61, 98–100]. As cited earlier, studies have cited decreases in cortisol levels with both clenching and chewing, with and without physical activity [49, 50, 100]. Yet what mechanism can explain the purported improvements in hormone levels with clenching? A rat model may explain the potential mechanisms that occur with a reduced stress response during clenching. Researchers have cited that restrained and stressed rats, when biting on a stick, had reductions in corticotrophin releasing factor and c-Fos in the hypothalamus which may be modulated by suppression of extracellular signal-regulated protein kinase 1/2 (pERK 1/2) in the paraventricular nucleus [60, 61, 101]. This link between the hypothalamus and the involvement in the jaw muscle via clenching may be explained by neuronal projections from the lateral hypothalamic connecting to the trigeminal motor nucleus in the rat model [102]. In addition, it was observed that the trigeminal motor nucleus is innervated by corticotropin-releasing factor-immunoreactive fibers within the amygdala, providing another explanation of effects on hormonal response during clenching [102].
Yet rat models cannot completely explain the stress response mechanisms involved in humans during chewing and clenching; thus, researchers use functional magnetic resonance imaging or positron-emission tomography to assess cortical activity and blood flow dynamics during clenching and chewing which have been cited to be a valid measures of assessing these tasks [98, 99, 103–106]. Momose et al. [105] demonstrated mastication increased cerebral blood flow in the sensorimotor cortex by approximately 26.5% during clenching [105]. Later studies cited significantly increased middle cerebral blood flow and significant activation of the sensorimotor cortex with clenching versus other tasks such as gum chewing and a hand motor task [98, 103]. Research also cites that activation within the dorsolateral prefrontal cortex (DLPFC, an area in the cerebral cortex) is most likely dependent on continuous teeth contact as occurs during clenching, and that intensity of the clenching most likely influences that magnitude of the cerebral activity within the sensorimotor cortex (area in cerebral cortex responsible for motor function) [99, 107]. Qin et al. [108] cited that the function of the DLPFC is likely affected by the HPA axis by decreasing levels of the catecholamines [108]. These findings are significant as it relates to mouthpiece use during exercise as they provide potential explanations for the cited decreases in cortisol and lactate with mouthpiece use during exercise [49–51, 58, 59]. Thus, enhanced cerebral blood flow may be a key piece in understanding these effects, with researchers citing improved cerebral blood flow rate when subjects are in a mandibular physiologic rest position [109]. Research by Otsuka and colleagues demonstrated how an experimentally induced retrusive mandibular position using a splint (defined as placing the mandible in a more backward position) resulted in an activation of the hypothalamus during clenching in two of eight subjects [110]. Though this evidence is not sufficient to make any definitive links between malocclusion and activation of the hypothalamus and subsequent stress response, it is another step in understanding a mechanism that could explain the cortisol response during exercise with a mouthpiece, a mouthpiece which has been cited as placing the mandible in a more forward mandibular position [48–50, 58]. In closing, more recent research aims to elucidate how increased cerebral blood flow could affect the hypothalamic response from stress with subsequent hormonal production such as cortisol. Miyake et al. [104] demonstrate that biting during stress and its effect on the hypothalamic response appears to be mediated by nitric oxide levels, specifically with biting resulting in decreased levels of nitric oxide versus not biting which leads to elevated nitric oxide levels [104]. They surmise that masticatory activity (biting down) during physiological stress results in an anti-stress response that may be facilitated by nitric oxide in the brain in which nitric oxide acts as an amplifier or feedback mechanism for neuronal activity during stress [104].
Although the interaction between the mandible and tongue has been clearly established in the literature, the relationship between such a reflex and effect on whole-body movement is sparsely cited. However, some research suggests that concurrent activation potentiation is the result of the jaw-repositioning and subsequent mandibular muscle contraction which thereby affect neuromuscular outcomes during exercise [42, 91]. Ebben first defined this phenomenon in his review of concurrent activation potentiation which he defined as an interaction between the Jendrassik maneuver, a type of remote voluntary contraction, in which individuals can increase the strength of reflexes by clenching their teeth and motor overflow, referring to communication between cortical areas through various parts of the brain [111]. In essence, Ebben cites literature suggesting that remote voluntary contractions acting through the H reflex will positively affect lower body musculature [111]. Examples of this phenomenon can be found in studies that show an increase in the soleus H reflex, which is described as a measure of the excitation of the spinal monosynaptic reflex in humans, during clenching, with a strong correlation in the increase of EMG activity of the masseter [112, 113]. The conclusion is that such a clenching response would be beneficial during stabilizing posture and improving fluidity of movement during muscular contraction, this being due to enhanced H reflex of the leg muscles with a concurrent reduction of the reciprocal Ia inhibition [113].
13.3.3 Genioglossus and Clenching and Involvement of the Mouthguard
Although there is substantial evidence of the importance of the genioglossus, as well as the effect of clenching, how does this relate to mouthguard use during exercise? Firstly, an appliance provides some type of stimulus to the tongue muscle as well as an increased opportunity for the individual to clench during exercise. Hidaka and colleagues found that with increased clenching, there was a resulting shift on the bite force such that there was a more balanced bite force (with balance bite force being defined as force placed on all occlusal contacts). They hypothesized that this outcome may be a mechanism which prevents damage to teeth and to the temporomandibular joint [114]. Thus, using a mouthguard may improve clenching capacity thereby resulting in changes of cerebral blood flow and hypothalamic response as noted earlier. Secondly, the design of the mouthguard is important to understand in light of its potential effect on the tongue muscle, i.e., a mandibular mouthguard versus a maxillary mouthguard differs in its impact on the tongue and may thereby affect outcomes associated with the genioglossus.
Accordingly, reviewing what is known will enable researchers to clarify the precise mechanism(s) that may be occurring with mouthguard/mouthpiece use during exercise. With research as it relates to the genioglossus and clenching effect, there is compelling evidence that a few aspects of mouthguard/mouthpiece use should be more carefully studied. Firstly, understanding the types of oral appliances used in previous and current studies should be evaluated for potential effect on vertical displacement, then understanding how the angle of the bite created by the mouthpieces affects vertical displacement as it relates to the TMJ should be reviewed. Murakami and colleagues who used magnetic resonance imaging (MRI) with and without a mouthguard made of a EVA material (Erkosoft Erkodent, Pfalzgrafenweiler, Germany) studied 26 healthy subjects wearing two different EVA mouthguards; MG1 created a 3 mm vertical displacement, while MG2 created a 6 mm vertical displacement [115]. It was cited in both mouthguard conditions that the condyle moved backward and upward in all subjects during clenching yet to a lesser extent than the non-clenching condition. During the non-clenching, MG1 condition, the condyle moved 1.15 mm downward and 2.10 mm forward, with MG2 moving 2.10 mm downward and 2.33 forward, a statistical difference between MG1 and MG2 [115]. Thus vertical displacement alone seems to create varying degrees of condylar distance from the mandibular fossa. A case study assessing the positioning of the temporomandibular joint between the boil and bite UA mouthguard, the custom mouthguard, and no-mouthpiece condition showed that the boil and bite provided greater distance between the condyle and the mandibular fossa, the custom provided lesser distance, and the no-mouthpiece condition resulted in the least distance created between the condyle and fossa, which may be due to the type of vertical displacement created by the two mouthpieces and no-mouthpiece conditions (unpublished data).

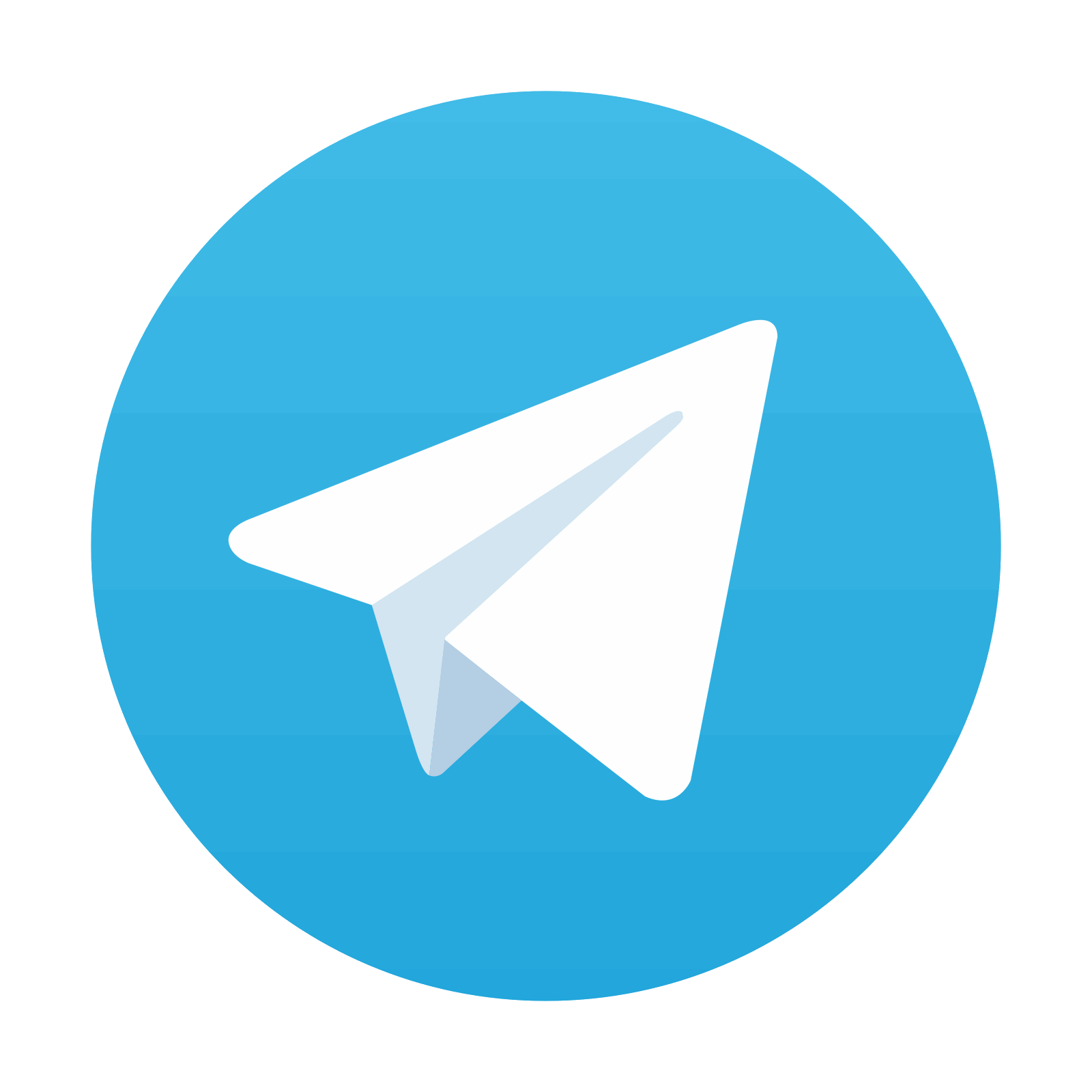
Stay updated, free dental videos. Join our Telegram channel

VIDEdental - Online dental courses
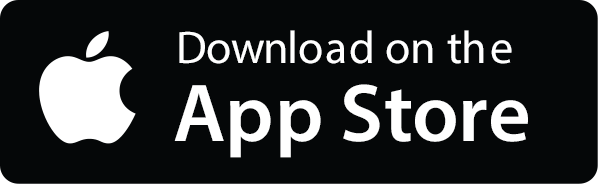
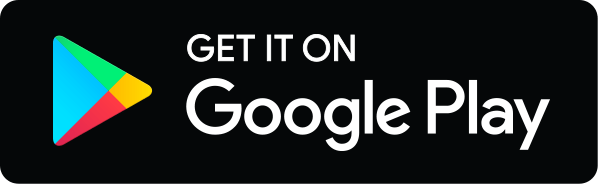