5.13
Antidysrhythmics
- Esmolol and metoprolol discussed on page 143
- See Figure 4.12 for antidysrhythmics classifications
Adenosine
- Mechanism of Action
- Adenosine receptor agonist
- Clinical Indications
- Stable AVNRT/AVRT
- First dose
- Adult: 6 mg IV
- Peds: 0.1 mg/kg IV
- Second dose
- Adult: 12 mg IV
- Peds: 0.2 mg/kg IV
- First dose
- Stable AVNRT/AVRT
- Cerebral
- Minimal
- Cardiovascular
- Transient conduction cessation at the SA and AV node
- ↓ MAP
- ↓ HR
- ↓ CO
- Pulmonary
- Bronchoconstriction
- Renal
- Minimal
- Hepatic
- Minimal
- Contraindications
- Any rhythm that is not AVRT/AVNRT
- WPW syndrome
- Asthma
- Board Facts
- Rapid injection following by a saline flush
- Raise limb to facilitate adenosine movement into central circulation
- If AVRT/AVNRT rhythm does not terminate after second dose of adenosine, consider monomorphic ventricular tachycardia
- Methylxanthines antagonize adenosine requiring an increased dose
- Carbamazepine and dipyridamole potentiate adenosine action and thus require a reduced dose [108]
- Transplanted hearts/denervated hearts require a reduced dose [109]
Amiodarone
- Mechanism of Action
- Na+, K+, and Ca2+ channel antagonist (Figure 4.12)
- Clinical Indications
- VF/pulseless VT
- First dose
- Adult: 300 mg IV
- Peds: 5 mg/kg IV
- Second dose
- Adult: 150 mg IV
- Peds 2.5 mg/kg IV
- First dose
- Stable wide QRS tachycardia with a pulse
- Adult: 150 mg IV over 10 minutes
- Peds: 5 mg/kg IV over 20–60 minutes
- Atrial fibrillation
- VF/pulseless VT
- Cerebral
- Minimal
- Cardiovascular [110]
- ↓ MAP
- Minimal change in HR
- ↑ CO
- Pulmonary
- Pulmonary fibrosis (in chronic use)
- Renal
- Hepatic
- Primary site of metabolism
- Hepatic toxicity (primarily with chronic use) [113]
- Contraindications
- Pregnancy
- Renal pathology
- Pulmonary pathology
- Hepatic pathology
- Thyroid pathology
- Board Facts
Atropine
- Mechanism of Action
- Muscarinic receptor antagonist
- Clinical Indications
- Bradycardia with a pulse
- Adult: 0.5 mg IV (Max 3 mg)
- Peds: 0.02 mg/kg IV (Max 1 mg)
- Peds: 0.02 mg/kg IM
- Coadministration with neuromuscular reversal
- Neuromuscular reversal covered on page 138
- Bradycardia with a pulse
- Cerebral
- May present as excitation or sedation in high doses
- Cardiovascular
- ↑ CO
- ↑ HR
- Low‐dose atropine may cause bradycardia [75]
- Pulmonary
- Bronchodilator
- ↓ Secretions and thicken them
- Renal
- Minimal
- Hepatic
- Primary site of metabolism
- Contraindications
- Acute angle glaucoma
- Cardiac transplantation due to denervation
- Paradoxical bradycardia can occur [76]
- Board Facts
- Crosses placenta and BBB
Diltiazem
- Mechanism of Action
- Non‐dihydropyridine calcium channel antagonist (Figure 4.12)
- Clinical Indications
- Stable atrial flutter
- 5–10 mg IV; Titrate, Max 50 mg
- 0.25 mg/kg initial; 0.35 mg/kg next
- 5–10 mg IV; Titrate, Max 50 mg
- Stable atrial fibrillation
- 5–10 mg IV; Titrate, Max 50 mg
- 0.25 mg/kg initial; 0.35 mg/kg next
- 5–10 mg IV; Titrate, Max 50 mg
- Stable AVNRT/AVRT
- 5–10 mg IV; Titrate, Max 50 mg
- 0.25 mg/kg initial; 0.35 mg/kg next
- 5–10 mg IV; Titrate, Max 50 mg
- Stable atrial flutter
- Cerebral
- ↑ CBF
- Cardiovascular
- ↑ CO
- ↓ HR
- ↓ MAP
- Pulmonary
- Minimal
- Renal
- Minimal
- Hepatic
- Primary site of metabolism
- Contraindications
- WPW syndrome
- Bradycardia
- Cardiac conduction delays
- Board Facts
Verapamil
- Mechanism of Action
- Non‐dihydropyridine calcium channel antagonist (Figure 4.12)
- Clinical Indications
- Stable atrial flutter
- 2.5–5 mg IV; Titrate to max 20 mg
- Stable AVNRT/AVRT
- 2.5–5 mg IV; Titrate to max 20 mg
- Stable atrial flutter
- Cerebral
- ↑ CBF
- Cardiovascular [118]
- ↓ CO
- ↓ HR
- ↓ MAP
- Pulmonary
- Minimal
- Renal
- Minimal
- Hepatic
- ↑ HBF [119]
- Contraindications
- WPW syndrome
- Bradycardia
- Cardiac conduction delays
- Board Facts
References
- 1 Peck, T.E. and Harris, B. (2021). Pharmacology for Anaesthesia and Intensive Care, 5e. Cambridge University Press.
- 2 Aranake, A., Mashour, G.A., and Avidan, M.S. (2013). Minimum alveolar concentration: ongoing relevance and clinical utility. Anaesthesia 68 (5): 512–522. https://doi.org/10.1111/anae.12168.
- 3 Cameron, C.B., Robinson, S., and Gregory, G.A. (1984). The minimum anesthetic concentration of isoflurane in children. Anesth. Analg. 63 (4): 418–420.
- 4 Ekberg, E., Nilsson, I.M., Michelotti, A. et al. (2023). Diagnostic criteria for temporomandibular disorders – INfORM recommendations: comprehensive and short‐form adaptations for adolescents. J. Oral Rehabil. https://doi.org/10.1111/joor.13488.
- 5 Fu, A.D., McDonald, H.R., Eliott, D. et al. (2002). Complications of general anesthesia using nitrous oxide in eyes with preexisting gas bubbles. Retina 22 (5): 569–574. https://doi.org/10.1097/00006982‐200210000‐00006.
- 6 Nagele, P., Brown, F., Francis, A. et al. (2013). Influence of nitrous oxide anesthesia, B‐vitamins, and MTHFR gene polymorphisms on perioperative cardiac events: the vitamins in nitrous oxide (VINO) randomized trial. Anesthesiology 119 (1): 19–28. https://doi.org/10.1097/ALN.0b013e31829761e3.
- 7 Hathout, L. and El‐Saden, S. (2011). Nitrous oxide‐induced B₁₂ deficiency myelopathy: perspectives on the clinical biochemistry of vitamin B₁₂. J. Neurol. Sci. 301 (1–2): 1–8. https://doi.org/10.1016/j.jns.2010.10.033.
- 8 Divatia, J.V., Vaidya, J.S., Badwe, R.A., and Hawaldar, R.W. (1996). Omission of nitrous oxide during anesthesia reduces the incidence of postoperative nausea and vomiting. A meta‐analysis. Anesthesiology 85 (5): 1055–1062. https://doi.org/10.1097/00000542‐199611000‐00014.
- 9 Combes, X., Schauvliege, F., Peyrouset, O. et al. (2001). Intracuff pressure and tracheal morbidity: influence of filling with saline during nitrous oxide anesthesia. Anesthesiology 95 (5): 1120–1124. https://doi.org/10.1097/00000542‐200111000‐00015.
- 10 Brioni, J.D., Varughese, S., Ahmed, R., and Bein, B. (2017). A clinical review of inhalation anesthesia with sevoflurane: from early research to emerging topics. J. Anesth. 31 (5): 764–778. https://doi.org/10.1007/s00540‐017‐2375‐6.
- 11 Torri, G. (2010). Inhalation anesthetics: a review. Minerva Anestesiol. 76 (3): 215–228.
- 12 Tanaka, S., Tsuchida, H., Nakabayashi, K. et al. (1996). The effects of sevoflurane, isoflurane, halothane, and enflurane on hemodynamic responses during an inhaled induction of anesthesia via a mask in humans. Anesth. Analg. 82 (4): 821–826. https://doi.org/10.1097/00000539‐199604000‐00025.
- 13 Tateishi, T., Asoh, M., Nakura, H. et al. (1999). Carbamazepine induces multiple cytochrome P450 subfamilies in rats. Chem. Biol. Interact. 117 (3): 257–268. https://doi.org/10.1016/s0009‐2797(98)00110‐0.
- 14 Frink, E.J., Morgan, S.E., Coetzee, A. et al. (1992). The effects of sevoflurane, halothane, enflurane, and isoflurane on hepatic blood flow and oxygenation in chronically instrumented greyhound dogs. Anesthesiology 76 (1): 85–90. https://doi.org/10.1097/00000542‐199201000‐00013.
- 15 Agnew, N.M., Pennefather, S.H., and Russell, G.N. (2002). Isoflurane and coronary heart disease. Anaesthesia 57 (4): 338–347. https://doi.org/10.1046/j.1365‐2044.2002.02469.x.
- 16 Burchardi, H. and Kaczmarczyk, G. (1994). The effect of anaesthesia on renal function. Eur. J. Anaesthesiol. 11 (3): 163–168.
- 17 Merin, R.G., Bernard, J.M., Doursout, M.F. et al. (1991). Comparison of the effects of isoflurane and desflurane on cardiovascular dynamics and regional blood flow in the chronically instrumented dog. Anesthesiology 74 (3): 568–574. https://doi.org/10.1097/00000542‐199103000‐00027.
- 18
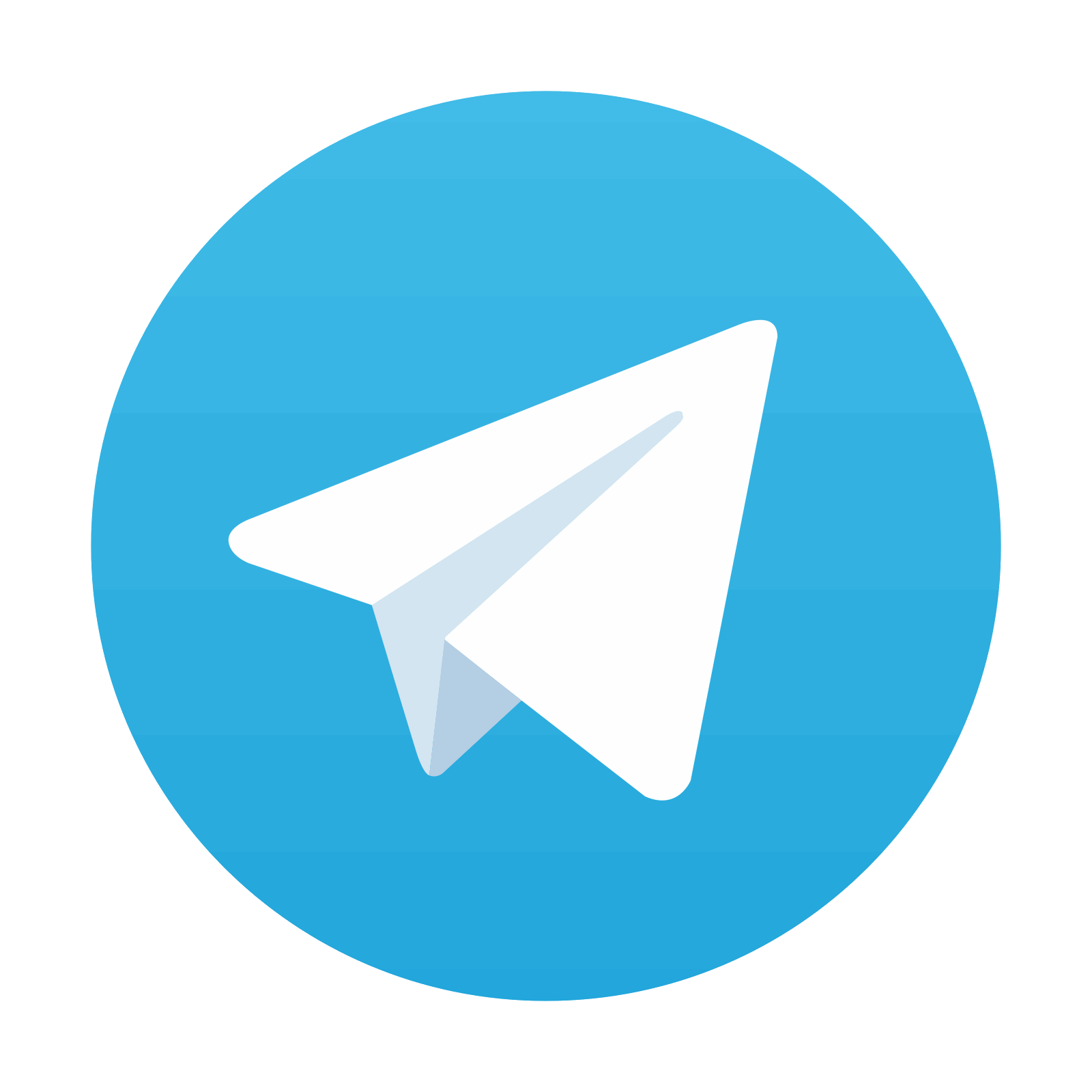
Stay updated, free dental videos. Join our Telegram channel

VIDEdental - Online dental courses
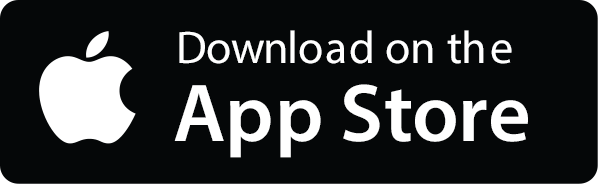
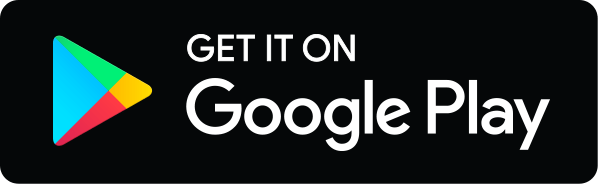