div epub:type=”chapter” role=”doc-chapter”>
P. Jain, M. Gupta (eds.)Digitization in Dentistryhttps://doi.org/10.1007/978-3-030-65169-5_5
5. Digitization and Dental Lasers
LaserRoot canalOral surgeryBoneTissueTechnology
5.1 Introduction
Lasers have gained immense use due to the optimization of the wavelength for human tissue interactions. Initially, dental lasers were primarily used for soft tissue surgical procedures, such as excisions and incisions. Instruments then evolved for use on both hard and soft oral structures [1, 2]. Photobiomodulation (PBM) is now universally used as a substitute for low-level laser therapy, has been shown to have a positive effect on living tissues, and as such have been used as a noninvasive alternative in the treatment of various pathological conditions [3, 4].
The biomedical and therapeutic application of the photonic energy is based on the light-tissue interactions leading to laser absorption by cellular components such as mitochondria cytochromes and endogenous chromospheres, which give rise to phenomena such as fluorescence, chemical reactions, and thermal effects [5].
PBM is NOT a thermal interaction in the sense of significantly raising the tissue temperature. These laser therapies often involve red blood cells due to the high optical absorbance of hemoglobin; thus, have proved to be the “most versatile” [6, 7]. The red blood cells are also found in many organs in high concentration. Therefore, the damaging effect of these irradiations occurs at the cell level since hemoglobin is located in red blood cells [8]. The damage is thermal and is related to mechanical stress, vapor bubbles, and overheating because of irradiation [9]. These physiological phenomena stimulate other processes in the cell such as enzyme inactivation, alteration of metabolic rate, and coagulation among other structural changes in the cell [10].
Important effects of low-energy laser light have been highlighted in the scientific works of literature, which are tissue regeneration, reduction of inflammation, pain relief, and immune system enhancement [11]. The low-energy intensity lasers have in recent years being used in the noninvasive treatment of musculoskeletal and cutaneous complications and have also gained increased use in wound healing, nerve repair, and pain management, among other clinical applications. In diabetic conditions where wound healing is compromised, low-energy intensity lasers have shown beneficial effects of healing impaired wounds [12, 13].
Laser used in clinical dentistry has spanned over 30 years. Prior to that, carbon dioxide lasers (CO2, wavelength 10.6 μm) were used in general medical surgeries and subsequently were applied to the soft tissue surgical procedures within the mouth [14]. Neodymium YAG (1.064 μm) was the first dental laser which was launched in 1989, and from there the next 10 years witnessed the emergence of different major wavelengths Er:YAG and Er,Cr:YSGG and diode semiconductor-based technology [15, 16].
5.2 Laser and its Components
LASER is an acronym for Light Amplification by Stimulated Emission of Radiation, which is based on theories and principles first put forth by Einstein in the early 1900s.The first actual laser system was introduced by Maiman in 1960 [17].
Laser energy is a man-made product and consists of photons of a single wavelength. The process of lasing occurs when an excited atom is stimulated to remit a photon before it occurs spontaneously; spontaneous emission of light results in unorganized light waves similar to light emitted by a light bulb [18]. Stimulated emission of photons generates a very coherent, collimated, monochromatic ray of light that is found nowhere else in nature. As that light is so concentrated and focused, it can have a decided effect on target tissue at a much lower energy level than natural light. The effect of laser energy on target tissue is dependent on its wavelength, which is determined by the lasing medium inside the laser device [19].
Laser irradiation is a type of electromagnetic radiation which exhibits the properties of a wave, and discrete energy packages referred to as photons. The short wavelengths such as high-energy ionizing irradiation cause the ionization of molecules in an indiscriminate manner with the far-infrared heating biological tissues [20, 21].
-
Gas discharge lasers.
-
Semiconductor diode lasers.
-
Optically pumped laser.
-
The final group that consists of X-ray lasers, combustion lasers, chemical lasers, and gas dynamic lasers.

A representational graphic of the basic components of a dental laser. The optical cavity contains the active medium and reflective surfaces. Initial energy is provided by an excitation source. Photons within the active medium are reflected, amplified, and then collimated. Those are emitted into a lens assembly, which then produces the useful laser beam. In a diode laser, the semiconductor medium is a wafer that contains layers of positively and negatively charged compounds, bounded by reflective coating. Several of these wafers are ganged together to produce a useful laser beam
The optical cavity contains the active medium and reflective surfaces. Initial energy is provided by an excitation source. Photons within the active medium are reflected, amplified, and then collimated. Those are emitted into a lens assembly, which then produces the useful laser beam [24].
In a diode laser, the semiconductor medium is a wafer that contains layers of positively and negatively charged compounds, bounded by reflective coating. Several of these wafers are grouped together to produce a useful laser beam [25, 26].

Approximate absorption curves of the currently available dental laser wavelengths. The curves represent hemoglobin, melanin, water, and tooth enamel (carbonated hydroxyapatite.) Above the light green line, there is absorption, and below it is transmission of the photonic energy
5.3 Laser Delivery Systems and Laser Emission Modes

(a) A small diameter glass fiber is paired with a disposable tip, used in contact with the target tissue. (b) A fiber optically delivered laser with a handpiece and a reusable tip, employed slightly of contact with the target tissue

(a) An optic fiber. (b) A hollow waveguide. (c) An articulated arm assembly. Usually, with an optic fiber, the laser beam is emitted with a contact tip; conversely with a hollow waveguide or an articulated arm, the beam is delivered without a tip in a non-contact mode
Lasers in the visible (445 and 532 nm) and near-infrared (from 810 nm to 1064 nm) range use fiber-optic strands by means of a handpiece with straight and precise tips that deliver the laser energy to the target tissue. This type of delivery system can degrade with time but is lightweight with a good tactile sensation and is easy to use and sterilize. Some newer models have more rugged fibers [31].
Erbium and CO2 devices are lasers with more rigid glass fibers, semiflexible hollow waveguides, or articulated arms. However, there is a loss of energy over time with lack of control due to internal reflection, with these systems [5]. For hard tissue procedures, a water spray is used for cooling, and that can be switched off for soft tissue surgery. In addition, lasers with articulated arm delivery systems utilize a progression of verbalized mirrors (generally 7) associated one to each other, prompting transmission of vitality [32, 33].
-
1.
Continuous wave mode where the laser energy/beam is transmitted continuously as long as the laser is activated. Carbon dioxide, argon, and diode lasers operate in this manner. Variations in this mode include the “gated emissions” where there is a periodic alteration of the laser energy being on or off (similar to blinking), thereby preventing laser light transmission. This design helps minimize some of the undesirable residual thermal damage associated with continuous wave devices. It is very important to pay attention to this effect during laser use in order to protect damage to the surrounding tissues. Some of the recent models of “gated” lasers feature pulse durations in the microsecond range.
-
2.
Free-running pulse lasers only operate in a unique pulsed mode, never continuously, where emission is in very short pulses in the microsecond range followed by a long time when the laser is off. Nd:YAG and Er:YAG as well as Er,Cr:YSGG devices operate as free-running pulsed lasers.
5.4 Types of Tissue Interactions

Approximate absorption curves of the currently available dental laser wavelengths
The hydroxyapatite crystals of teeth and bone absorb carbon dioxide, and the erbium family’s photons. The visible and near-infrared wavelengths have virtually no interaction with either enamel or water. The erbium family is very highly absorbed in water, while carbon dioxide is about 10 times less absorbed [21]. All wavelengths have different depths of penetration through soft tissue because of the different water absorption characteristics. Diode lasers can reach deeper into the tissue approximately several thousand layers, whereas the erbium lasers are absorbed on the surface [33, 36].
Lasers can have various effects on the target tissue. One effect is photothermal: simply stated, light is converted to heat. When the tissue absorbs the photonic energy used during surgical procedure, it elevates the temperature. When a temperature of 100 °C is reached, vaporization of the water within the tissue occurs. This process is called ablation. At temperatures between 60 and 100 °C, denaturization of the proteins starts occurring, without dehydrated and then burned, resulting in an undesirable effect called carbonization or vaporization [37, 38].
Nonsurgical, low-level power laser applications include photo-bio modulation (PBM), diagnostics, photo-activated antibacterial processes, laser tooth whitening, and laser scanning of tooth cavity preparations [39]. Another effect is photochemical effects such as curing of the composite resin [40]. Lastly, some lasers can also produce tissue fluorescence, which is used as a diagnostic method for caries detection [41, 42].
5.5 Lasers in Dentistry

How the laser works on the tooth
lists the currently available dental lasers whose emission is on the visible spectrum. Column 1 lists the generic name of the laser and the color of light emitted; column 2 describes the typical uses of that laser; column 3 lists the elements of the active medium; column 5 lists the emission wavelength; and column 5 shows abbreviations for the emission modes
Generic name of laser |
Typical uses |
Active medium |
Emission wavelength |
Emission mode |
---|---|---|---|---|
Semiconductor diode, visible blue |
Soft tissue procedures, tooth whitening |
Indium gallium nitride |
445 nm |
CW, GP |
KTP solid state visible green |
Soft tissue procedures, tooth whitening |
Neodymium-doped yttrium aluminum garnet (Nd:YAG) and potassium titanyl phosphate (KTP) |
532 nm |
CW, GP |
Low-level lasers, visible red semiconductor, or gas lasers |
Photobiomodulation therapy (PBM), photodynamic therapy (PDT), or carious lesion detection. |
Variations of gallium arsenide or indium gallium arsenide phosphorus diodes Helium neon gas |
600–670 nm 632 nm |
CW, GP |
This table lists the currently available dental lasers whose emission is on the invisible spectrum. Column 1 lists the generic name of the laser; Column 2 describes the typical uses of that laser; Column 3 lists the elements of the active medium; Column 4 lists the emission wavelength or range of wavelengths; and Column 5 shows abbreviations for the emission modes
Generic name of laser |
Typical uses |
Active medium |
Emission wavelength (nm) |
Emission mode |
---|---|---|---|---|
Low-level diode lasers, near infrared |
Photobiomodulation therapy (PBM), photodynamic therapy (PDT) |
Variations of aluminum gallium arsenide diodes |
800–900 |
CW, GP |
Semiconductor diode, near-infrared |
Soft tissue procedures |
Aluminum gallium arsenide |
800–830 |
CW, GP |
Semiconductor diode, near-infrared |
Soft tissue procedures |
Aluminum/indium gallium arsenide |
940 |
CW, GP |
Semiconductor diode, near-infrared |
Soft tissue procedures |
Indium gallium arsenide |
980 |
CW, GP |
Semiconductor diode, near-infrared |
Soft tissue procedures |
Indium gallium arsenide, phosphorus |
1064 |
CW, GP |
Solid state, near-infrared |
Soft tissue procedures |
Neodymium-doped yttrium aluminum garnet (Nd:YAG) |
1064 |
FRP |
Solid state, near-infrared |
Soft tissue procedures, endoscopic procedures |
Neodymium-doped yttrium aluminum perovskite (Nd:YAP) |
1340 |
FRP |
Solid state, mid-infrared |
Soft tissue procedures, hard tissue procedures |
Erbium, chromium-doped yttrium scandium gallium garnet (Er,Cr:YSGG) |
2780 |
FRP |
Solid state, mid-infrared |
Soft tissue procedures, hard tissue procedures |
Erbium-doped yttrium aluminum garnet (Er:YAG) |
2940 |
FRP |
Gas, far infrared |
Soft tissue procedures, hard tissue procedures |
Carbon dioxide (CO2) laser, with an active medium isotopic gas |
9300 |
FRP |
Gas, far-infrared |
Soft tissue procedures |
Carbon dioxide (CO2) laser with an active medium of a mixture of gases |
10,600 |
CW, GP, FRP |
5.6 Lasers Generally Practiced in Dentistry
5.6.1 Carbon Dioxide Laser
CO2 laser’s photonic energy has a very high affinity for water; it causes rapid soft tissue removal and hemostasis. The penetration of depth is relatively shallow. A newly available model of CO2 with ultrashort pulse duration can be used for hard and soft dental tissue.
5.6.2 Neodymium Yttrium Aluminum Garnet Lasers
Absorption of this wavelength is high in pigmented tissue; therefore, it is sufficient for cutting and coagulating dental soft tissues, with high-grade hemostasis. Other uses include nonsurgical periodontal therapy.
5.6.3 Erbium Laser
The family of erbium lasers has two distinct wavelengths, Er, Cr: YSGG lasers and Er: YAG lasers. Erbium wavelengths have a secondary affinity for hydroxyapatite crystals and the most powerful absorption of water in any dental laser wavelength. It is the laser of selection for treatment of dental hard tissues, and can be used for soft tissue ablation because the dental soft tissue comprises a large percentage of water.
5.6.4 Diode Laser
The diode laser is a solid-state semiconductor containing various combinations of aluminum, gallium, arsenide, and occasionally indium producing different laser wavelengths, varying from approximately 445 to 1064 nm. These wavelengths are absorbed principally by tissue pigment melanin and hemoglobin, and inadequately absorbed by hydroxyapatite and water.
Uses of lasers in dentistry
Specialty |
Uses |
---|---|
Oral surgery |
Major or minor surgical procedures such as flap surgeries, frenectomies, removal of hyperplastic tissues, operculectomy, excisional biopsy, root end resection, gingivectomy procedures, exposure of impacted teeth, and vestibuloplasty Treatment of abscess, aphthous ulcer, granuloma, epulis, irritation fibroma Hemangioma and curettage |
Periodontics |
Flap surgery, frenectomy, gingival contouring/gingivectomy, pocket treatment |
Orthodontics |
Post orthodontic removal of residual cement Exposure of impacted teeth |
Endodontics |
Bleaching Canal irrigation Root resection in endodontic surgeries |
Prosthetic and restorative dentistry |
Caries removal Curing of material Removal of fractured restorations Etching of the tooth Sulcus deepening Crown contouring and lengthening Smile design |
Pediatric dentistry |
Removal of caries in deciduous teeth Pulpotomy and pulpectomy procedures |
5.7 Soft Tissue Application
5.7.1 Photobiomodulation
It is also known as “soft laser therapy” and is based on the concept that low level of doses of specific coherent wavelengths can turn on or turn off certain cellular components or functions. At low laser doses (2 J/cm2), laser utilization stimulates proliferation, while at large doses (16 J/cm2) it is suppressive [43, 44]. This technique induces analgesic, anti-inflammatory, and biomodulation effects at molecular level improving tissue healing processes and less postoperative discomfort for patients, without any negative effects [10, 45]. This technique is most useful in medically compromised patients where wound closure and tissue healing is of prime importance.
LLLT (low level laser) results in vasodilation of the cells, which causes increase in local blood flow, relaxation of smooth muscles, thus bringing in oxygen and further migration of immune cells to the targeted tissue. This in turn results in rapid maturation and regeneration [9, 46]. Accurate effects of PBM on the healing of lesions of recurrent aphthous stomatitis in humans have been recorded. Research has shown that LPL encourages healing and dentinogenesis following pulpotomy. This makes it useful in the healing of mucositis and oropharyngeal ulceration in patients enduring radiotherapy for head or neck cancer [47, 48].

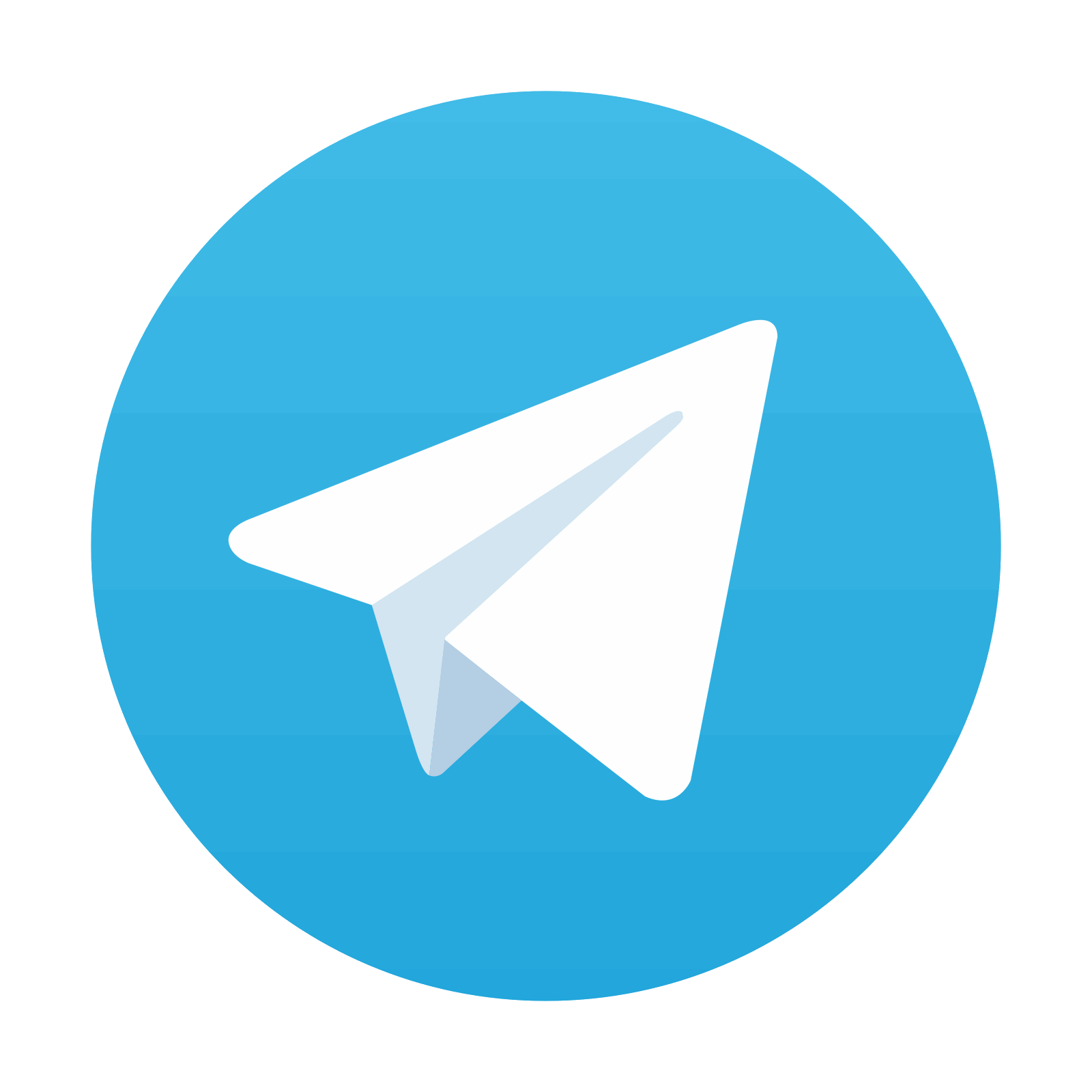
Stay updated, free dental videos. Join our Telegram channel

VIDEdental - Online dental courses
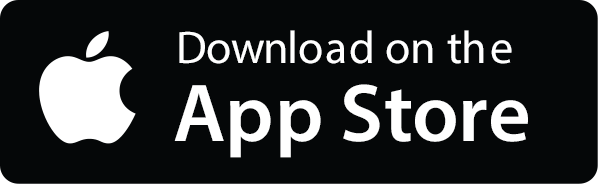
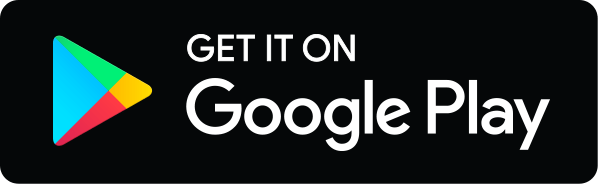