Abstract
Objectives
The impact of implant surfaces in dental biofilm development is presently unknown. The aim of this investigation was to assess in vitro the development of a complex biofilm model on titanium and zirconium implant surfaces, and to compare it with the same biofilm formed on hydroxyapatite surface.
Methods
Six standard reference strains were used to develop an in vitro biofilm over sterile titanium, zirconium and hydroxyapatite discs, coated with saliva within the wells of pre-sterilized polystyrene tissue culture plates. The selected species used represent initial ( Streptococcus oralis and Actinomyces naeslundii ), early ( Veillonella parvula ), secondary ( Fusobacterium nucleatum ) and late colonizers ( Porphyromonas gingivalis and Aggregatibacter actinomycetemcomitans ). The developed biofilms (growth time 1 to 120 h) were studied with confocal laser scanning microscopy using a vital fluorescence technique and with low-temperature scanning electron microscopy. The number (colony forming units/biofilm) and kinetics of the bacteria within the biofilm were studied with quantitative PCR (qPCR). As outcome variables, the biofilm thickness, the percentage of cell vitality and the number of bacteria were compared using the analysis of variance.
Results
The bacteria adhered and matured within the biofilm over the three surfaces with similar dynamics. Different surfaces, however, demonstrated differences both in the thickness, deposition of the extracellular polysaccharide matrix as well as in the organization of the bacterial cells.
Significance
While the formation and dynamics of an in vitro biofilm model was similar irrespective of the surface of inoculation (hydroxyapatite, titanium or zirconium), there were significant differences in regards to the biofilm thickness and three-dimensional structure.
1
Introduction
Biofilms are complex microbial communities developed on solid non-shed surfaces exposed to a wet environment . In the oral cavity bacteria may attach to different types of surfaces including teeth, prosthetic devices and dental implants. Biofilms on dental implants have not been characterized, although similar to the etiological association between dental biofilms and the most prevalent oral infections , the formation and maturation of biofilms on dental implant surfaces may have pathogenic implications in the development of peri-implant diseases, such as peri-implant mucositis or peri-implantitis .
Studies on the microbiota around dental implants have shown that when bacteria colonize the peri-implant crevice soon after implant placement, the dominant species are streptococci and members of the yellow and purple complexes, such as Actinomyces spp., soon developing a poly-microbial community . Similarly, the microorganisms associated to the implant surface in presence of healthy peri-implant tissues are predominantly Gram-positive cocci and rods . As the biofilm matures, members of the red, orange, and green complexes colonize and in clinical situations associated with failing implants or peri-implant diseases, there is a net predominance of orange and red complex species .
Even though changes in the composition of the peri-implant microbiota have been associated with the pathogenesis of peri-implant diseases , there is controversy on how the implant surface characteristics may affect bacterial colonization and biofilm formation, thus potentially influencing the initiation and progression of peri-implant diseases .
With the purpose of studying the implant surface bacterial interactions, several in vitro biofilm models have been tested and validated . These systems have usually included one or two bacterial species or short-term evaluations (24 h or less), thus lacking the ability to study the dynamics of the biofilm maturation and its potential pathogenicity. Our research has developed and tested an in vitro model for complex biofilm formation on tooth (hydroxyapatite) surfaces, both under static and dynamic conditions, using six bacterial species comprising ( Streptococcus oralis and Actinomyces naeslundii ), early ( Veillonella parvula ), secondary ( Fusobacterium nucleatum ) and late colonizers ( Porphyromonas gingivalis and Aggregatibacter actinomycetemcomitans ) . We, therefore, aim in this investigation to test this in vitro biofilm model on implant surfaces, testing both titanium and zirconium surfaces, and to compare the obtained biofilms with the standard on hydroxyapatite surfaces.
2
Material and methods
2.1
Bacterial strains and culture conditions
Standard reference strains of S. oralis CECT 907T, V. parvula NCTC 11810, A. naeslundii ATCC 19039, F. nucleatum DMSZ 20482, A. actinomycetemcomitans DSMZ 8324 and P. gingivalis ATCC 33277 were used. Bacteria were grown on blood agar plates (Blood Agar Oxoid No 2; Oxoid, Basingstoke, UK), supplemented with 5% (v/v) sterile horse blood (Oxoid), 5.0 mg mL −1 hemin (Sigma, St. Louis, MO, USA) and 1.0 mg mL −1 menadione (Merck, Darmstadt, Germany) in anaerobic conditions (10% H 2 , 10% CO 2 , and balance N 2 ) at 37 °C for 24–72 h.
2.2
Material specimens
Three different surface materials were used: sterile calcium hydroxyapatite discs with a 7 mm of diameter and a thickness 1.8 mm (standard deviation, SD = 0.2) (Clarkson Chromatography Products, Williamsport, PA, USA); sterile titanium discs, grade 2, SLA (Sand-blasted, Large grit, Acid-etched) surface of 5 mm of diameter (Straumann; Institut Straumann AG, Basel, Switzerland); sterile zirconium oxide (ZrO 2 ) discs, surface of 5 mm of diameter with a rough micro surface obtained after chemical treatment with a hot solution of hydrofluoric acid according to a proprietary process of Institut Straumann AG (Institut Straumann AG, Basel, Switzerland). The resultant rough surface topography has a S a value of 0.55 mm (SD = 0.01). This rough surface topography when evaluated with SEM has similar topography as Ti-SLA surface implants, although zirconia surfaces seemed to have a flatter profile with less porosity [ S a value of Ti-SLA surface of 1.17 mm (SD = 0.04)] .
2.3
Saliva preparation
Un-stimulated saliva was obtained from healthy volunteers in 10 mL aliquots at least 1.5 h after eating, drinking or tooth brushing. Each saliva sample was treated with 2.5 mmol L −1 dl -dithiothreitol (Sigma) for 10 min with continuous stirring in order to reduce salivary protein aggregation. It was then centrifuged (10 min, 4 °C, 9000 × g ) and the obtained supernatant were diluted (1:1) with phosphate buffered saline (PBS). The sample was then filtered and sterilized through a 0.22 μm pore size Millex GV low-protein-binding filter X50 (Millipore, Millipore Corporation Bedford, USA) and stored at -20 °C. The efficacy of this protocol was assessed by plating processed saliva samples onto supplemented blood agar plates for 72 h at 37 °C and confirmed by the lack of any bacterial growth on either aerobically or anaerobically incubated plates.
2.4
Biofilm development
Biofilms were grown on the three surfaces as previously described by Sánchez et al. . In brief, pure cultures of each bacteria were grown anaerobically in a protein-rich medium containing brain-heart infusion (BHI) (Becton, Dickinson and Company, Franklin Lakes, NJ, USA) supplemented with 2.5 g L −1 mucin (Oxoid), 1.0 g L −1 yeast extract (Oxoid), 0.1 g L −1 cysteine (Sigma), 2.0 g L −1 sodium bicarbonate (Merck), 5.0 mg mL −1 hemin (Sigma), 1.0 mg mL −1 menadione (Merck) and 0.25% (v/v) glutamic acid (Sigma). The bacterial growth was harvested at mid-exponential phase (measured by spectrophotometry), and a mixed bacteria suspension in modified BHI medium containing 10 3 colony forming units (CFU) mL −1 for S. oralis , 10 5 CFU mL −1 for V. parvula and A. naeslundii , and 10 6 CFU mL −1 for F. nucleatum , A. actinomycetemcomitans and P. gingivalis was prepared. Sterile discs were coated with treated saliva for 4 h at 37 °C in sterile plastic tubes, and then placed in the wells of a 24-well tissue culture plate (Greiner Bio-one, Frickenhausen, Germany). Each well was inoculated with 1.5 mL mixed bacteria suspension prepared and incubated in anaerobic conditions (10% H 2 , 10% CO 2 , and balance N 2 ) at 37 °C for 1, 12, 24, 48, 72, 96 and 120 h. Plates containing only culture medium were also incubated to check for sterility.
2.5
Analysis of biofilms by confocal laser scanning microscopy (CLSM)
Three independent trials (on three different occasions) with trios of biofilms were carried out. Before CLSM analysis, the discs were sequentially rinsed in 2 mL of sterile PBS (immersion time per rinse, 10 s) three times, in order to remove non-adherent bacteria. Non-invasive confocal imaging of fully hydrated biofilms was carried out using a fixed-stage TCS SP2 confocal microscope (Leica Microsystems, Milton Keynes, United Kingdom) incorporating a 488 nm Ar/Ar–Kr laser scan head mounted on a vibration-free platform. The objective lenses used were 63× water-immersion lenses (Leica Microsystems). Specimens were stained with LIVE/DEAD ® BacLightTM Bacterial Viability Kit solution (Molecular Probes B. V., Leiden, The Netherlands) at room temperature. The 1:1 fluorocrome ratio with a staining time of 9 ± 1 min was used to obtain the optimum fluorescence signal at the corresponding wave lengths (Syto9: 515–530 nm; propidium iodide (PI): >600 nm). At least three separate and representative locations on the discs covered with biofilm were selected for these measurements (based on the presence of stacks or “towers” identified in the confocal view field). Within each area, the thickest point was measured by determining the upper and lower limits of the biofilm. The CLSM software was set to take a z-series of scans (xyz) of 1 μm thickness (8 bits, 1024 × 1024 pixel). Image stacks were analyzed with the Leica Confocal Lite ® software (Leica Microsystems). In order to quantify the biomass and cell viability within the biofilm, total fluorescent staining of the confocal micrographs was analyzed using a specific image analysis software program (MetaMorph ® 7.6; Molecular Devices Corporation, Sunnyvale, CA, USA). Fluorescence intensity thresholds were manually set for each of the fluorescent colors.
2.6
Analysis of biofilms by low-temperature scanning electron microscopy (LTSEM)
Three independent trials (on three different occasions) with trios of biofilms were carried out. For this analysis, the discs were sequentially rinsed in 2 mL of sterile PBS (immersion time per rinse, 10 s) three times, in order to remove non-adherent bacteria. The specimens were then fixed onto the specimen holder of the cryo-transfer system using liquid nitrogen and then transferred to a preparation unit via an air-lock transfer. The frozen specimens were transferred directly via a second air lock to the microscope cold stage, where they were etched for 2 min by raising the temperature to −90 °C. After ice sublimation, the etched surfaces were sputter-coated with gold in the preparation unit and then transferred onto the cold stage of the scanning electron microscope chamber. Surfaces were observed at −135 °C with a DMS 960 scanning electron microscope (Digital Scanning Microscope, Zeiss).
2.7
DNA isolation and quantitative polymerase chain reaction (qPCR)
Before the DNA isolation, the discs were sequentially rinsed in 2 mL of sterile PBS (immersion time per rinse, 10 s) three times, in order to remove non-adherent bacteria. Biofilm DNA was isolated from all samples using a commercial kit (MolYsis Complete5; Molzym GmbH & CoKG, Bremen, Germany), following manufacturer’s instructions (the protocol for bacterial DNA extraction was followed from step 6, avoiding preliminary steps). The hydrolysis probes 5′ nuclease assay PCR method was used for detecting and quantifying the bacterial DNA. Primers and probes were obtained by Life Technologies Invitrogen (Carlsbad, CA, USA), Applied Biosystems (Carlsbad, CA, USA) and Roche (Roche Diagnostic GmbH; Mannheim, Germany) and were targeted against 16S rRNA gene ( Table 1 ). The qPCR amplification was performed in a total reaction mixture volume of 20 μL. The reaction mixtures contained 10 μL of 2× master mixture (LC 480 Probes Master; Roche), optimal concentrations of primers and probe (900, 900 and 300 nM for S. oralis ; 300, 300 and 300 nM for A. naeslundii ; 750, 750 and 400 nM for V. parvula ; 300, 300 and 200 nM for A. actinomycetemcomitans ; 300, 300 and 300 nM, for P. gingivalis and 600, 600 and 300 nM for F. nucleatum ), and 5 μL of DNA from samples. The negative control was 5 μL of sterile water [no template control (NTC)] (Water PCR grade, Roche). The samples were subjected to an initial amplification cycle of 95 °C for 10 min, followed by 40 cycles at 95 °C for 15 s and 60 °C for 1 min Analyses was performed with a LightCycler ® 480 II thermocycler (Roche). The plates used in the study were FramStar 480 of natural frame and white wells ( 4 titude; The North Barn; Damphurst Lane, UK), sealed by QPCR Adhesive Clear Seals ( 4 titude).
Bacteria | Sequence (5′–3′) | Length (bp) | Reference |
---|---|---|---|
So | |||
Forward | CAACGATACATAGCCGACCTGAG | 97 | Present study |
Reverse | TCCATTGCCGAAGATTCC | ||
Probe | 6FAM-CTCCTACGGGAGGCAGCAGTAGGGA-BBQ | ||
Vp | |||
Forward | TGCTAATACCGCATACGATCTAACC | 66 | |
Reverse | GCTTATAAATAGAGGCCACCTTTCA | ||
Probe | 6FAM-CTATCCTCGATGCCGA-TAMRA | ||
An | |||
Forward | GGCTGCGATACCGTGAGG | 103 | Present study |
Reverse | TCTGCGATTACTAGCGACTCC | ||
Probe | 6FAM-CCCTAAAAGCCGGTCTCAGTTCGGAT-BBQ | ||
Pg | |||
Forward | GCGCTCAACGTTCAGCC | 67 | |
Reverse | CACGAATTCCGCCTGC | ||
Probe | 6FAM-CACTGAACTCAAGCCCGGCAGTTTCAA-TAMRA | ||
Aa | |||
Forward | GAACCTTAC CTACTCTTGACATCCGAA | 80 | |
Reverse | TGCAGCACCTGTCTCAAAGC | ||
Probe | 6FAM-AGAACTCAGAGATGGGTTTGTGCCTTAGGG-TAMRA | ||
Fn | |||
Forward | GGATTTATTGGGCGTAAAGC | 162 | |
Reverse | GGCATTCCTACAAATATCTACGAA | ||
Probe | 6FAM-CTCTACACTTGTAGTTCCG-TAMRA |
Each DNA sample was analyzed in duplicate. Quantification cycle ( Cq ) values, previously known as cycle threshold ( Ct ) values, describing the PCR cycle number at which fluorescence rises above the baseline, were determined using the provided software package (LC 480 Software 1.5; Roche). Quantification of viable cells by qPCR was based on standard curves, following a protocol previously described . The correlation between Cq values and CFU/mL was automatically generated through the software (LC 480 Software 1.5; Roche).
All assays were developed with a linear quantitative detection range established by the slope range of 3.3–3.6 cycles/log decade, r 2 > 0.997 and an efficiency range of 1.9–2.0.
Measures to avoid carryover DNA were established, such as establishing barrier methods, using flow cabin and physical separation of pre- and post-PCR procedures. In spite of this, when NTC was detectable, the limit of detection was established on the last value of the standard curve that holds five cycles of difference with NTC.
2.8
Statistical analyses
The selected outcome variables to compare biofilms formed on different surfaces were micrometers of height, percentage of vitality and CFU/biofilm. An experiment-level analysis was performed for each study parameter ( n = 9 for CLSM results and n = 3 for qPCR results). Shapiro–Wilk goodness-of-fit tests and distribution of data were used to assess normality. Data were expressed as means, SD and 95% confidence intervals (95% CI). To compare the effects of the material surface at different exposure times on micrometers of height, percentage of vitality and CFU/biofilm, one-way analysis of variance and post hoc testing with Bonferroni’s correction for multiple comparisons were used.
Results were considered statistically significant at p < 0.05. A software package (IBM SPSS Statistics 19.0; IBM Corporation, Armonk, NY, USA) was used for all data analysis.
2
Material and methods
2.1
Bacterial strains and culture conditions
Standard reference strains of S. oralis CECT 907T, V. parvula NCTC 11810, A. naeslundii ATCC 19039, F. nucleatum DMSZ 20482, A. actinomycetemcomitans DSMZ 8324 and P. gingivalis ATCC 33277 were used. Bacteria were grown on blood agar plates (Blood Agar Oxoid No 2; Oxoid, Basingstoke, UK), supplemented with 5% (v/v) sterile horse blood (Oxoid), 5.0 mg mL −1 hemin (Sigma, St. Louis, MO, USA) and 1.0 mg mL −1 menadione (Merck, Darmstadt, Germany) in anaerobic conditions (10% H 2 , 10% CO 2 , and balance N 2 ) at 37 °C for 24–72 h.
2.2
Material specimens
Three different surface materials were used: sterile calcium hydroxyapatite discs with a 7 mm of diameter and a thickness 1.8 mm (standard deviation, SD = 0.2) (Clarkson Chromatography Products, Williamsport, PA, USA); sterile titanium discs, grade 2, SLA (Sand-blasted, Large grit, Acid-etched) surface of 5 mm of diameter (Straumann; Institut Straumann AG, Basel, Switzerland); sterile zirconium oxide (ZrO 2 ) discs, surface of 5 mm of diameter with a rough micro surface obtained after chemical treatment with a hot solution of hydrofluoric acid according to a proprietary process of Institut Straumann AG (Institut Straumann AG, Basel, Switzerland). The resultant rough surface topography has a S a value of 0.55 mm (SD = 0.01). This rough surface topography when evaluated with SEM has similar topography as Ti-SLA surface implants, although zirconia surfaces seemed to have a flatter profile with less porosity [ S a value of Ti-SLA surface of 1.17 mm (SD = 0.04)] .
2.3
Saliva preparation
Un-stimulated saliva was obtained from healthy volunteers in 10 mL aliquots at least 1.5 h after eating, drinking or tooth brushing. Each saliva sample was treated with 2.5 mmol L −1 dl -dithiothreitol (Sigma) for 10 min with continuous stirring in order to reduce salivary protein aggregation. It was then centrifuged (10 min, 4 °C, 9000 × g ) and the obtained supernatant were diluted (1:1) with phosphate buffered saline (PBS). The sample was then filtered and sterilized through a 0.22 μm pore size Millex GV low-protein-binding filter X50 (Millipore, Millipore Corporation Bedford, USA) and stored at -20 °C. The efficacy of this protocol was assessed by plating processed saliva samples onto supplemented blood agar plates for 72 h at 37 °C and confirmed by the lack of any bacterial growth on either aerobically or anaerobically incubated plates.
2.4
Biofilm development
Biofilms were grown on the three surfaces as previously described by Sánchez et al. . In brief, pure cultures of each bacteria were grown anaerobically in a protein-rich medium containing brain-heart infusion (BHI) (Becton, Dickinson and Company, Franklin Lakes, NJ, USA) supplemented with 2.5 g L −1 mucin (Oxoid), 1.0 g L −1 yeast extract (Oxoid), 0.1 g L −1 cysteine (Sigma), 2.0 g L −1 sodium bicarbonate (Merck), 5.0 mg mL −1 hemin (Sigma), 1.0 mg mL −1 menadione (Merck) and 0.25% (v/v) glutamic acid (Sigma). The bacterial growth was harvested at mid-exponential phase (measured by spectrophotometry), and a mixed bacteria suspension in modified BHI medium containing 10 3 colony forming units (CFU) mL −1 for S. oralis , 10 5 CFU mL −1 for V. parvula and A. naeslundii , and 10 6 CFU mL −1 for F. nucleatum , A. actinomycetemcomitans and P. gingivalis was prepared. Sterile discs were coated with treated saliva for 4 h at 37 °C in sterile plastic tubes, and then placed in the wells of a 24-well tissue culture plate (Greiner Bio-one, Frickenhausen, Germany). Each well was inoculated with 1.5 mL mixed bacteria suspension prepared and incubated in anaerobic conditions (10% H 2 , 10% CO 2 , and balance N 2 ) at 37 °C for 1, 12, 24, 48, 72, 96 and 120 h. Plates containing only culture medium were also incubated to check for sterility.
2.5
Analysis of biofilms by confocal laser scanning microscopy (CLSM)
Three independent trials (on three different occasions) with trios of biofilms were carried out. Before CLSM analysis, the discs were sequentially rinsed in 2 mL of sterile PBS (immersion time per rinse, 10 s) three times, in order to remove non-adherent bacteria. Non-invasive confocal imaging of fully hydrated biofilms was carried out using a fixed-stage TCS SP2 confocal microscope (Leica Microsystems, Milton Keynes, United Kingdom) incorporating a 488 nm Ar/Ar–Kr laser scan head mounted on a vibration-free platform. The objective lenses used were 63× water-immersion lenses (Leica Microsystems). Specimens were stained with LIVE/DEAD ® BacLightTM Bacterial Viability Kit solution (Molecular Probes B. V., Leiden, The Netherlands) at room temperature. The 1:1 fluorocrome ratio with a staining time of 9 ± 1 min was used to obtain the optimum fluorescence signal at the corresponding wave lengths (Syto9: 515–530 nm; propidium iodide (PI): >600 nm). At least three separate and representative locations on the discs covered with biofilm were selected for these measurements (based on the presence of stacks or “towers” identified in the confocal view field). Within each area, the thickest point was measured by determining the upper and lower limits of the biofilm. The CLSM software was set to take a z-series of scans (xyz) of 1 μm thickness (8 bits, 1024 × 1024 pixel). Image stacks were analyzed with the Leica Confocal Lite ® software (Leica Microsystems). In order to quantify the biomass and cell viability within the biofilm, total fluorescent staining of the confocal micrographs was analyzed using a specific image analysis software program (MetaMorph ® 7.6; Molecular Devices Corporation, Sunnyvale, CA, USA). Fluorescence intensity thresholds were manually set for each of the fluorescent colors.
2.6
Analysis of biofilms by low-temperature scanning electron microscopy (LTSEM)
Three independent trials (on three different occasions) with trios of biofilms were carried out. For this analysis, the discs were sequentially rinsed in 2 mL of sterile PBS (immersion time per rinse, 10 s) three times, in order to remove non-adherent bacteria. The specimens were then fixed onto the specimen holder of the cryo-transfer system using liquid nitrogen and then transferred to a preparation unit via an air-lock transfer. The frozen specimens were transferred directly via a second air lock to the microscope cold stage, where they were etched for 2 min by raising the temperature to −90 °C. After ice sublimation, the etched surfaces were sputter-coated with gold in the preparation unit and then transferred onto the cold stage of the scanning electron microscope chamber. Surfaces were observed at −135 °C with a DMS 960 scanning electron microscope (Digital Scanning Microscope, Zeiss).
2.7
DNA isolation and quantitative polymerase chain reaction (qPCR)
Before the DNA isolation, the discs were sequentially rinsed in 2 mL of sterile PBS (immersion time per rinse, 10 s) three times, in order to remove non-adherent bacteria. Biofilm DNA was isolated from all samples using a commercial kit (MolYsis Complete5; Molzym GmbH & CoKG, Bremen, Germany), following manufacturer’s instructions (the protocol for bacterial DNA extraction was followed from step 6, avoiding preliminary steps). The hydrolysis probes 5′ nuclease assay PCR method was used for detecting and quantifying the bacterial DNA. Primers and probes were obtained by Life Technologies Invitrogen (Carlsbad, CA, USA), Applied Biosystems (Carlsbad, CA, USA) and Roche (Roche Diagnostic GmbH; Mannheim, Germany) and were targeted against 16S rRNA gene ( Table 1 ). The qPCR amplification was performed in a total reaction mixture volume of 20 μL. The reaction mixtures contained 10 μL of 2× master mixture (LC 480 Probes Master; Roche), optimal concentrations of primers and probe (900, 900 and 300 nM for S. oralis ; 300, 300 and 300 nM for A. naeslundii ; 750, 750 and 400 nM for V. parvula ; 300, 300 and 200 nM for A. actinomycetemcomitans ; 300, 300 and 300 nM, for P. gingivalis and 600, 600 and 300 nM for F. nucleatum ), and 5 μL of DNA from samples. The negative control was 5 μL of sterile water [no template control (NTC)] (Water PCR grade, Roche). The samples were subjected to an initial amplification cycle of 95 °C for 10 min, followed by 40 cycles at 95 °C for 15 s and 60 °C for 1 min Analyses was performed with a LightCycler ® 480 II thermocycler (Roche). The plates used in the study were FramStar 480 of natural frame and white wells ( 4 titude; The North Barn; Damphurst Lane, UK), sealed by QPCR Adhesive Clear Seals ( 4 titude).
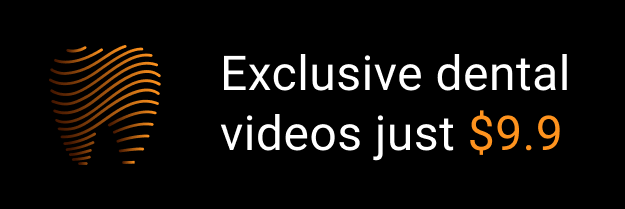