Introduction
Genetic and environmental etiologic factors have been described for maxillary canine impaction, except for the trabecular bone characteristics in the impacted area. The aim of this study was to evaluate the surface area and fractal dimension of the alveolar bone on cone-beam computed tomography (CBCT) images of patients with maxillary impacted canines.
Methods
The sample comprised preorthodontic treatment CBCT images of 49 participants with maxillary impacted canines (31 unilateral and 18 bilateral). CBCT images were acquired in portrait mode (17 × 23 cm high field of view) at 120 kV, 5 mA, 8.9-seconds exposure time, and 0.3-mm voxel size. Coronal slices (0.3 mm) were obtained from the right and left alveolar processes between the first and second maxillary premolars. We collected 64 × 64-pixel regions of interest between the premolars to assess maxillary bone area and fractal dimension using ImageJ software (National Institutes of Health, Bethesda, Md). Comparisons were made using paired t tests and linear regression. Repeated measurements were obtained randomly from about 20% of the sample.
Results
In subjects with unilateral impactions, the maxillary bone area ( P = 0.0227) was higher in the impacted side, with a mean difference of 245.5 pixels (SD, 569.2), but the fractal dimension ( P = 0.9822) was not, −0.0003 pixels (SD, 0.082). Comparisons of unilateral and bilateral subjects using a general linear mixed model test confirmed the increased bone area in the impacted side ( P = 0.1062). The repeated measurements showed similar results.
Conclusions
The maxillary alveolar bone area is increased in the impacted side compared with the nonimpacted side.
Highlights
- •
Fractal analysis has been proposed as an accurate measurement of bone density.
- •
The method was applied to CBCT images of patients with unilateral impacted canines.
- •
Differences were observed between the impacted and nonimpacted sides.
Maxillary permanent canine impaction has been reported to affect 1% to 3% of the general population. The maxillary canine is the second most frequently impacted tooth, after the third molar. The impaction is more common in female patients, with some authors reporting its occurrence twice as high as in male patients. The incidence of palatally displaced canines has been observed to be 2 to 3 times greater than buccally impacted canines. Previous studies have suggested that palatally displaced canines are associated with increased space in the dental arch, whereas buccally shifted canines are associated with inadequate space for eruption. Other potential etiologies for palatally impacted canines have been documented, including genetic defects and the absence or underdevelopment of the lateral incisors.
The development and increasing use of cone-beam computed tomography (CBCT) in the dental field has facilitated the study and classification of impacted canines. CBCT has several advantages compared with traditional 2-dimensional techniques. CBCT can produce 3-dimensional images of structures located in different spatial planes. Other advantages of CBCT include a much lower radiation dose, a much lower cost, and less image distortion compared with other computed tomography modalities.
It has been known for some time that CBCT can be used to locate impacted canines 3 dimensionally and determine the degree of root resorption. A recent study compared CBCT against a traditional panoramic radiograph; the authors found that CBCT was more predictive in determining the position labio-palatally of the impacted canine. Jung et al also found that the extent of root resorption was more accurately classified with CBCT. Botticelli et al speculated that CBCT can lead to a better prognosis after treatment.
CBCT has been used to find radiographic predictors of canine impaction. The strongest predictors were found to be canine angulation in reference to the lateral incisor, the cusp tip angulation in relation to the occlusal plane, and the overall crown position. However, there is a lack of studies assessing the bone quality of the maxilla in the impacted and nonimpacted contralateral canine areas using CBCT. This may be because bone density measurements in Hounsfield units using CBCT are not reliable. Other approaches to measuring bone quality and microstructure, such as trabecular number and fractal dimension analysis, have been proposed and validated. Hsu et al found high correlations between gray-scale values taken by CBCT and bone microarchitecture parameters when measured by microcomputed tomography. Ibrahim et al concluded that CBCT can be used to evaluate the microstructure of trabecular bone at the site of implants, with the most reliable indicator being trabecular number. Similarly, fractal dimension analysis, calculated from CBCT scans, has been shown to be effective in determining bony structures. Previous studies have concluded that fractal values calculated from CBCT scans correlate with bone mineral density and trabecular patterning. Due to the irregular and random nature of trabecular bone, measuring fractal dimension may be a more reliable method of analysis compared with the traditional analysis with Hounsfield units.
To our knowledge, previous studies have not proposed bone density of the alveolar process as an etiologic factor of canine impaction. Nevertheless, understanding the effect of the microstructure of the alveolar bone on the etiology of impacted canines may aid in diagnosis and treatment of the disorder. Therefore, we used CBCT to assess the relationship of the presence or absence of unilateral and bilateral maxillary canine impactions and the microstructure of the maxillary alveolar bone, as measured by the bone surface area and the bone fractal dimension. The null hypothesis was that there is no difference in bone density between the impacted and nonimpacted sides as measured by bone surface area and the bone fractal dimension.
Material and methods
The institutional review board of the University of Minnesota approved this retrospective study (number 1506M73701) using the pretreatment CBCT scans of patients with unilateral or bilateral canine impaction seeking treatment at the University of Minnesota Orthodontic Clinic. Three hundred sixty-nine potential participants were initially identified using the preliminary clinical or radiographic diagnosis at the initial orthodontic examination or during the treatment planning visits. From these 369 patients with unilateral or bilateral canine impaction, 105 had pretreatment CBCT scans. All CBCT scans were completed at the University of Minnesota School of Dentistry. Canine impaction was defined as an unerupted canine when root development was complete, or by the complete eruption of the contralateral canine. Similarly, complete eruption was defined as the tooth in its expected occlusion and position. The inclusion criteria for the study were (1) CBCT scan showing unilateral or bilateral maxillary canine impaction with complementing clinical diagnosis, (2) complete eruption of the contralateral canine in unilateral patients, (3) classification of buccal or palatal impaction based on the location of at least 50% of the crown compared with the alveolar ridge in a coronal cross section, and (4) no prior orthodontic treatment. The exclusion criteria were (1) evidence of systemic disease affecting bone health; (2) CBCT scans that displayed oral pathology; (3) congenitally missing teeth, supernumerary teeth, dentigerous cyst, or an enlarged cystic follicle; (4) history of dental trauma or anterior maxillary surgery; (5) evidence of motion or any other significant artifact in the CBCT scan; and (6) periodontal abnormalities. A complete review of each patient’s dental and medical histories and the CBCT scans was completed to verify these criteria. Forty-nine subjects were selected based our inclusion and exclusion criteria (20 male, 29 female; age at time of the scan, 10-34 years). There were 31 subjects with unilateral canine impaction and 18 with bilateral impaction. Of the 31 subjects with unilateral impactions, 20 were identified as having palatal impactions and 11 had buccal impactions. In those with bilateral impactions, 18 of the impacted canines were impacted palatally and 18 were impacted buccally.
CBCT scans were acquired with an i-CAT Next Generation scanner (Imaging Sciences International, Hatfield, Pa). CBCT images were acquired in portrait mode (17 cm diameter × 23 cm high field of view) at 120 kV, 5 mA, 8.9-seconds exposure time, and 0.3-mm voxel size.
Using Office Picture Manager (Microsoft, Redmond, Wash), images were cropped to a 64 × 64-pixel region of interest. Regions of interest were selected from areas that did not contain dental structures, cortical bone, or vascular canals. The maxillary alveolar process interproximal to the first and second premolars was selected as the region of interest because of the availability of trabecular bone ( Fig 1 ).

A description of the mathematics associated with fractal measurements has been previously described. Fractal objects have been described as irregularly shaped objects that cannot be approximated by typical polygons. Due to its irregular nature, trabecular bone can be described mathematically in terms of a fractal dimension as a number between 1 and 2. In terms of bone fractal analysis, a bony region containing a sample of 100% bone would correspond to a fractal value of 2, whereas a region containing 0% bone would correspond to a fractal value of 1. In terms of bone marrow fractal analysis, a fractal value of 2 would correspond to 100% marrow space, and a fractal value of 1 would correspond to 100% bone. Because every sample of trabecular bone contains dense bony regions as well as marrow spaces, fractal values of 1 and 2 are only theoretical and cannot naturally exist. Therefore, the range of possible fractal values of trabecular bone, measured in terms of the bone or the bone marrow, must lie between 1 and 2.
The specific procedures for site selection and fractal measurement have been described in a previous study. Briefly, using the “implant” screen of the i-CATVision software (version 1.7.2.4, Imaging Sciences International), coronal images were obtained from the alveolar process between the first and second, both right and left, maxillary premolars. The images were viewed on a 22-in wide-screen flat panel monitor with a NVIDIA Quadro FX 4,600 graphics card (2208WFP; Dell, Round Rock, Tex) under subdued ambient lighting. Images were obtained from the scans and saved as JPEG files for analysis. All images were set to the “normal” filter, 0.3-mm slice thickness using the slice thickness tool, default brightness and contrast, and maximum magnification using the magnification tool. Pixel measurements were calculated with Microsoft Office Picture Manager ( Figs 2 , A , and 3 , A ).


Image processing was done with ImageJ software (National Institutes of Health, Bethesda, Md). Following the protocol adopted from White and Rudolph, JPEG images of the regions of interest were binarized into regions of trabecular bone and bone marrow spaces. Image preparation was sequential and consisted of blurring, using the Gaussian blur function (r = 35) ( Figs 2 , B , and 3 , B ). The blurred image was subtracted from the original image to eliminate noise and soft tissue variation ( Figs 2 , C , and 3 , C ). As published in previous studies, the constant value of 128 was added to each pixel ( Figs 2 , D , and 3 , D ). The image threshold was adjusted to convert the image to a black and white binary image ( Figs 2 , E , and 3 , E ). All pixel values less than or equal to the value of the threshold were converted to black, and the additional pixels greater than the threshold were converted to white. The binarized image threshold was defined to have a value between 0 and 128. The resulting image estimated bone marrow regions in black and trabecular bony regions in white ( Fig 2 , F ). To further remove image noise, the image was eroded ( Fig 2 , G ) and dilated once ( Fig 2 , H ). An alternate processing of the images was performed with the images inverted ( Fig 3 , F ); therefore, the bone marrow regions were estimated in white, and trabecular bony regions were in black ( Fig 3 , G ). The alternate processing followed the same protocol of blurring ( Fig 3 , B , and C ), value adding ( Fig 3 , D ), and threshold adjusting ( Fig 3 , E ) but were then inverted before erosion ( Fig 3 , H ) and dilation ( Fig 3 , I ) of the images. For simplicity, the first images used to calculate bone surface area and bone fractal dimension were designated as the bone sample. The images used to calculate the bone marrow surface area and the bone marrow fractal dimension were designated as the bone marrow sample.
To calculate the bone surface area of the 64 × 64 pixel region of interest, a histogram was generated. It separated the binarized image of 4096 pixels based on their color, black or white. The white pixels had a value of 0 on the histogram, and the black pixels had a value of 128. The number of white pixels was recorded; it approximated the bone surface area in the bone sample or the bone marrow surface area in the bone marrow sample. Each set of the 2 sets of images was measured according to this protocol for the number of white pixels.
After the bone surface area and bone marrow surface area were approximated, the images were inverted ( Figs 2 , I , and 3 , J ) and skeletonized ( Figs 2 , J , and 3 , K ). For the bone measurements, the skeletonized images approximated the bone spicule midlines in black and all other regions in white, whereas in the bone marrow images, the bone marrow midline was calculated in black. Fractal dimension calculations were completed using the ImageJ function, “fractal box count,” as previously described.
Statistical analysis
Paired t tests were used to compare mean bone area and mean fractal analysis between impacted and nonimpacted sites for single unilateral impactions and between impacted sites for single bilateral impactions. A general linear mixed-model approach was used for per-site analyses. Patient was treated as a random effect to account for potential within-patient correlations. Software (version 9.3; SAS, Cary, NC) was used for the analyses. P values less than 0.05 were considered statistically significant.
Results
A total of 49 patients were included in our study. The comparison of the impacted and nonimpacted sides in the unilateral subjects showed that the bone surface area was increased on the impacted side ( P = 0.0227), whereas there was no difference in the bone fractal dimension between the sides ( Table I ). Similarly, the bone marrow surface area was decreased in the impacted side ( P = 0.0315), but there was no difference in bone marrow fractal dimension between the impacted and nonimpacted sides. Therefore, the null hypothesis was rejected. In subjects with bilaterally impacted maxillary canines, we found no significant differences among any characteristic analyzed (bone fractal dimension, bone surface area, bone marrow fractal dimension, and bone marrow surface area) between the right and left sides. Interestingly, we observed that the bone fractal dimension and the bone marrow fractal dimension of bilateral impacted canines was greater than those for impacted canines in subjects with unilateral impactions (bone fractal dimensions, 1.266, 1.254 >1.235; bone marrow fractal dimensions, 1.323, 1.319 > 1.305; Tables I and II ). In the per-site analysis, the nonaffected side of the unilateral impacted patients functioning as controls showed a trend for increased bone surface area ( P = 0.1062) and decreased bone marrow surface area ( P = 0.1325) in the impacted site, although it was not statistically significant; these results are summarized in Table III . The observation of greater bone surface areas and fractal dimensions in the impacted side supported our hypothesis that trabecular bone is denser in the sites of impaction. This was confirmed by the observation of greater amounts of bone marrow surface area and bone marrow fractal dimension measurements on the nonimpacted side. The impacted sites palatal and buccal impactions were not associated with bone surface area or fractal dimension values: P = 0.1912 and P = 0.0702, respectively. A similar trend was seen in the bone marrow surface area ( P = 0.0823) and bone marrow fractal dimension ( P = 0.3141) measurements. Additionally, as a part of our analysis, approximately 20% of the bone sample was reanalyzed to verify the reliability of the method; these measurements are summarized in Table IV . Although the Pearson correlation coefficient was low, both the first and second measurements showed similar bone characteristics in the impacted and nonimpacted sides, despite the differences in the anatomy caused by different images and locations of regions of interest.
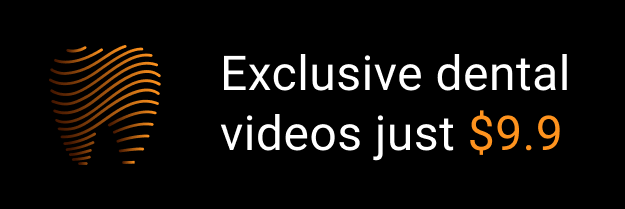