Introduction
Orthodontic tooth movement results from increased inflammation and osteoclast activation. Since patients of all ages now routinely seek orthodontics treatment, we investigated whether age-dependent biologic responses to orthodontic force correlate with the rate of tooth movement.
Methods
We studied 18 healthy subjects, adolescents (11-14 years) and adults (21-45 years), with Class II Division 1 malocclusion requiring 4 first premolar extractions. Canines were retracted with a constant force of 50 cN. Gingival crevicular fluid was collected before orthodontic treatment and at days 1, 7, 14, and 28 after the canine retraction. Cytokine (IL-1β, CCL2, TNF-α) and osteoclast markers (RANKL and MMP-9) were measured using antibody-based protein assays. Pain and discomfort were monitored with a numeric rating scale. The canine retraction rate was measured from study models taken at days 28 and 56.
Results
Although the cytokine and osteoclast markers increased significantly in both age groups at days 1, 7, and 14, the increases were greater in adults than in adolescents. Interestingly, the rate of tooth movement in adults was significantly slower than in adolescents over the 56-day study period. Adults also reported significantly more discomfort and pain.
Conclusions
Age is a significant variable contributing to the biologic response to orthodontic tooth movement. Adults exhibited a significantly higher level of cytokine and osteoclasts activity but, counterintuitively, had a significantly slower rate of tooth movement.
Highlights
- •
Age is an important factor in the biologic response to orthodontic forces.
- •
Cytokine and osteoclast markers increased significantly in adolescents and adults in the first weeks of orthodontic force application.
- •
Increases in cytokine and osteoclast markers are greater in adults than in adolescents in response to the same orthodontic force level.
- •
The rate of tooth movement in adults is slower than in adolescents.
- •
Adults report more pain and discomfort than adolescents in response to same force level.
Highly integrated cellular signaling plays a critical role in controlling the rate of orthodontic tooth movement. Orthodontic force triggers these responses by inducing chemokine and cytokine (ie. inflammatory markers) release in the periodontium. These inflammatory markers recruit osteoclast precursors and induce their differentiation and activation via the RANK-RANKL pathway. The level of osteoclast activation controls the rate of bone resorption and, subsequently, the rate of tooth movement. Inhibiting inflammation decreases the rate of tooth movement, while promoting inflammation significantly increases the rate of tooth movement.
If the biologic responses to orthodontic forces are so well defined, why then do orthodontists encounter a wide range of clinical responses to similar treatments? Why does it take longer to close extraction spaces in some patients compared with others? Why do we obtain a strong orthopedic correction using functional appliances in some patients but not in others? Why do we observe root resorption in some patients but not in others? These questions support the notion that orthodontic tooth movement results from a complex array of interacting biologic variables, not just the cellular signaling pathways described above. These variables can be extrinsic, such as magnitude of force and type of tooth movement, or they can be intrinsic, such as systemic factors, periodontal health, and root morphology. One variable is the effect of the patient’s age on orthodontic-induced inflammation and the rate of tooth movement. During maturation, alveolar bone gradually becomes denser, whereas the periodontal ligament becomes more fibrotic. These changes may affect how these tissues respond to microtrauma induced by orthodontic forces. Age is a clinically relevant biologic variable because patients of all ages are now routinely seeking orthodontic treatment. Therefore, understanding age-dependent responses to orthodontic forces can improve our selection of force systems to provide more efficient and safer treatment for all orthodontics patients.
We hypothesized that younger patients will have a more robust biologic response to orthodontic forces that results in a faster rate of tooth movement when compared with older patients. If age is a significant factor in the biologic response to orthodontic forces, our current standard of care warrants significant changes as patients of different ages are exposed to similar force systems, regardless of their biologic differences.
Material and methods
A nonrandomized, single-center, single-blinded clinical study was approved by the Institutional Review Board of New York University (IRB #S12-00298). Between January 2013 and December 2015, healthy subjects of both sexes, regardless of their ethnicity, were recruited from patients seeking comprehensive orthodontic treatment at the New York University Department of Orthodontics ( Table I ). The subjects were divided into 2 age groups: adolescents (11-14 years) and adults (21-36 years). Additionally, they had fully erupted maxillary canines in a Class II Division 1 malocclusion that required extraction of both maxillary first premolars and at least 3 mm of distal movement.
Inclusion criteria | Exclusion criteria |
---|---|
Age range, 11-14 or 21-45 years | Long-term use (6 month before study enrollment) of antibiotics, phenytoin, cyclosporin, anti-inflammatory drugs, systemic corticosteroids, and calcium channel blockers |
Class II Division 1 malocclusion with overjet <10 mm, Pg-Nper <18 mm, ANB <7°, SN-GoGn <38° |
Extreme skeletal Class II malocclusion or crossbite |
Have permanent dentition at least from first molar to contralateral first molar, and need canine retraction of 3 mm or more | Systemic disease |
Nonsmokers | Radiographic evidence of bone loss |
No gingivitis, no active periodontal disease, no untreated caries | Past periodontal disease on maxillary canines; past periodontal treatments during the 6-month period before study enrollment |
Plaque index ≤1 | Poor oral hygiene |
Gingival Index ≤1 | Probing depths >4 mm on any tooth |
Four orthodontic residents were trained and calibrated by the principal investigators (M.A., C.T.). They were responsible for examining the subjects, determining their eligibility, and rendering the orthodontic treatment under the supervision of a faculty member (E.K.) who was not the principal investigator. Before starting orthodontic treatment, patients who met the selection criteria completed an informed consent form, as adults or as guardian to a minor. The orthodontic residents (S.A., R.K., T.E., M.A.) rendering the treatment were aware of the subjects’ age, but the investigators (A.A., C.S.) performing data analysis were blinded to the subjects’ identity and age.
Routine orthodontic records were obtained for all subjects before orthodontic treatment, including portrait and intraoral photographs, panoramic radiographs, lateral cephalograms, periodontal measurements, and alginate impressions. At the start of treatment, fixed appliances were bonded on both arches (0.022-in MBT prescription) including maxillary canine brackets with an auxiliary vertical slot (GAC International, Bohemia, NY). The teeth were leveled and aligned as needed with sequential archwires from 0.016-in nickel-titanium to 0.017 × 0.025-in stainless steel. All subjects received the same treatment protocol and were monitored for oral hygiene and periodontal status at each clinic visit throughout the orthodontic treatment.
The patients were referred to the same surgeon for extraction of the maxillary first premolars to minimize operator variability. Canine retraction was not initiated until leveling and aligning were achieved, and after the last archwire was passive for at least 2 months, and at least 6 months after premolar extractions. Canine retraction began by connecting a calibrated 50-cN nickel-titanium closing-coil spring (GAC International) generating a constant force from the power arm extending from the accessory tube of the molar bands to a power arm extending from the ipsilateral canine bracket ( Fig 1 , A ). The length of the power arm was determined by the estimated location of the center of resistance using periapical radiographs, to allow force application to be as close to the center of resistance as possible, therefore facilitating bodily movement of the canines. To minimize the movement of adjacent teeth, all incisors and posterior teeth (from second premolar to second molar) were ligated as segments with a ligature wire. One canine was randomly selected from each subject for analysis to minimize the effect of uneven occlusal force due to habitual occlusion predominantly on 1 side.

The patients were asked to refrain from taking pain medications during treatment and were seen 24 hours after canine retraction for the first follow-up visit. At each subsequent visit, the force generated by the coil was checked and adjusted, and the appliances were monitored for any deformation or change in position caused by chewing.
The timetable of events and data collected at different time points are summarized in Table II . This clinical study was concluded after 8 weeks of canine retraction, and the subjects continued to receive orthodontic treatment. Routine orthodontic final records were taken at the end of treatment.
Leveling and aligning to stage of 0.016 × 0.022-in SS | 0-6 months | ||
Placement and activation of canine retraction apparatus | ≥6 months after extractions | ||
Monitoring OTM (GCF sampling) | Pre-Tx sample (0 months) | Start of canine retraction (≥6 months) | 1, 7, 14, and 28 days after canine retraction |
Monitoring OH (GI, PD, PI) | Pre-Tx sample (0 months) | Start of canine retraction (≥6 months) | 1, 7, 14, 28, and 56 days after canine retraction |
Intraoral photos, alginate impressions, and study models | Pre-Tx sample (0 months) | Start of canine retraction (≥6 months) | 28 and 56 days after canine retraction |
The sample size was calculated based on the results of our previous clinical study, assuming an estimated 50% difference in the expression of inflammatory markers between the 2 age groups. Type I error was set at 5%, and the power of the statistical test was set at 90% (power, 0.9; β, 0.1). Based on this calculation, a sample size of 8 per age group was suggested (total, 16 subjects). We opted to enroll 9 subjects per group (total, 18 subjects) to account for any dropouts.
To evaluate the level of inflammatory markers, gingival crevicular fluid (GCF) was collected from the distobuccal gingival crevice of the maxillary canines at the following times: before orthodontic treatment; immediately before canine retraction (day 0); and 1, 7, 14, and 28 days after canine retraction began. GCF collection was performed as described previously using filter-paper strips (Periopaper; Oraflow, Smithtown, NY).
Sample volume was assessed with Periotron 8000 (Oraflow) according to the manufacturer’s instructions. Total protein amount was quantified using the BCA protein assay kit (Pierce, Rockford, Ill). An estimated volume of 0.6 to 1.2 μL of GCF was collected and diluted with phosphate-buffered saline solution (Invitrogen, Burlington, Ontario, Canada) to obtain the sample of 50 to 100 μL required for analysis. Cytokine levels were measured using a custom glass slide-based protein array for the following cytokines: IL-1β, CCL2 (MCP1), TNF-α, RANKL, and MMP-9 (RayBiotech, Norcross, Ga) according to the manufacturer’s instructions.
To evaluate the rate of canine retraction, alginate impressions were taken at the following times: before the orthodontic appliances were bonded, immediately before canine retraction, and 28 and 56 days after canine retraction. Impressions were immediately poured with plaster (calcium sulfate). The models were labeled with the date taken and the subject’s assigned study number. On the palatal surfaces of the lateral incisors and canines, vertical lines were drawn from the middle of the incisal edge to the middle of the cervical line, dividing each crown into equal halves ( Fig 1 , B ). Three landmarks along these lines were marked at the incisal edge, in the middle of the crown, and at the cementoenamel junction or the gingival line ( Fig 1 , C ). Distances between these landmarks on each canine and its adjacent lateral incisor were measured and averaged using a digital caliper (Orthopli, Philadelphia, Pa) with an accuracy of 0.01 mm. The amount of canine retraction was calculated by subtracting the averaged distances between 2 selected time points.
Both intraobserver and interobserver errors were evaluated. Intraobserver error was evaluated by individual investigators who measured 10 models twice at least 2 weeks apart. Interobserver error was evaluated using the same set of 10 models measured by investigators (A.A., C.S.). The Dahlberg equation was applied to estimate random errors, and the paired t test was applied to identify systematic errors according to the method of Houston. Random errors were 0.059 for intraobserver evaluation and 0.091 mm for interobserver evaluations; these were not statistically significant. Systematic errors were also small and not statistically significant ( P = 0.85 for intraobserver and P = 0.81 for interobserver errors).
The subjects were asked to assess their level of discomfort immediately before canine retraction (day 0) and 1, 7, 28, and 56 days after canine retraction with a numeric rating scale, which is a highly reliabile tool comparable with a visual analog scale. The patients were instructed to choose a number (from 0 to 10) that best described their pain: 0 indicated no pain, and 10 indicated worst possible pain.
Statistical analysis
After confirming normal distribution of samples by the Shapiro-Wilk test, we assessed group comparisons with analysis of variance. Pairwise multiple comparison analysis was performed with the Tukey post hoc test. In some experiments, paired and unpaired t tests were used to compare the 2 groups. Two-tailed P values were calculated, and P <0.05 was set as the level of statistical significance.
Results
Eighteen subjects (9 adolescents, 9 adults) were recruited and completed the study. The adolescent group comprised 5 girls and 4 boys with a mean age of 13.3 years. The adult group comprised 6 women and 3 men with a mean age of 31 years ( Table III ). The subjects had similar types and severities of malocclusion ( Table IV ). All patients maintained good oral hygiene throughout the study and took no additional medications, including analgesics.
Patients | Adolescents | Adults |
---|---|---|
Number (sex) | 9 (5 female, 4 male) | 9 (6 female, 3 male) |
Age range (y) | 11-14 | 23-36 |
Mean age, SD (y) | 13.3 ± 0.9 | 31 ± 5.5 |
Ethnic origin | ||
White | 1 | 3 |
African American | 3 | 2 |
Asian | 2 | 3 |
Hispanic | 3 | 1 |
Cephalometric measurement | Adult | Adolescent | P value |
---|---|---|---|
ANB (°) | 5.2 ± 0.8 | 4.6 ± 0.51 | 0.096 |
GoGn-SN (°) | 28.8 ± 3.2 | 30.2 ± 2.7 | 0.33 |
U1-SN (°) | 108 ± 3.6 | 107.9 ± 4.1 | 0.59 |
IMPA (°) | 97.5 ± 4.4 | 97.7 ± 3.2 | 0.31 |
Overjet (mm) | 5.25 + 0.6 | 4.7 ± 0.7 | 0.092 |
GCF samples were collected at different time points ( Table II ), and the concentrations of selected inflammatory markers in GCF were measured by protein arrays ( Fig 2 ). Before orthodontic treatment (baseline) or before canine retraction (day 0), there was no significant difference in the GCF levels of IL-1β, CCL2, and TNF-α protein between the 2 age groups ( P >0.05). Likewise, there was no significant difference in GCF levels of IL-1β, TNF-α, and CCL2 proteins at baseline and day 0 ( P >0.05) in either group.

In the adult group, the concentrations of IL-1β, TNF-α, and CCL2 increased by 3.5-, 4.2-, and 4.3-fold, respectively, 1 day after canine retraction began compared with day 0; all increases were statistically significant ( P <0.05). Seven days after canine retraction began, the concentrations of IL-1β, TNF-α, and CCL2 decreased slightly compared with day 1; however, these values were still significantly higher ( P <0.05) than at day 0, by 2.7-, 2.3-, and 2.9-fold, respectively. At days 14 and 28, the concentrations of all 3 inflammatory markers decreased back to day 0 levels ( P >0.05).
In the adolescent group, 1 day after canine retraction began, the concentrations of IL-1β, TNF-α, and CCL2 increased 1.9-, 2.3-, and 2.1-fold, respectively, compared with day 0 ( P <0.05). However, no significant differences were observed at any later times ( P >0.05).
The concentrations of all 3 markers were significantly higher in adults than in adolescents at both days 1 and 7 (0.05), but no significant differences between the 2 age groups were observed at any other times ( Fig 2 ).
To evaluate the difference in osteoclast activation between the 2 age groups in response to the same magnitude of orthodontic force, GCF concentrations of the osteoclast markers RANKL and matrix metalloproteinase 9 (MMP-9) were assayed. At day 0, RANKL and MMP-9 concentrations were not significantly different from the baseline in either age group ( P >0.05), and there was no significant difference between the age groups ( Fig 3 ). However, 1, 7, and 14 days after canine retraction, RANKL concentrations in the adult group increased significantly by 2.9-, 5.8-, and 5.1-fold, respectively, compared with day 0 ( P <0.05). Similarly, RANKL concentrations in the adolescent group increased significantly by 2.1-, 3.8-, and 3.7-fold after canine retraction at 1, 7, and 14 days, respectively ( P <0.05). Although the concentrations of RANKL between the adolescent and adult groups were not significantly different at day 1 ( P >0.05), they were significantly higher in adults at days 7 and 14 ( P <0.05). At day 28, RANKL concentration returned to day 0 level ( P >0.05; Fig 3 , A ).

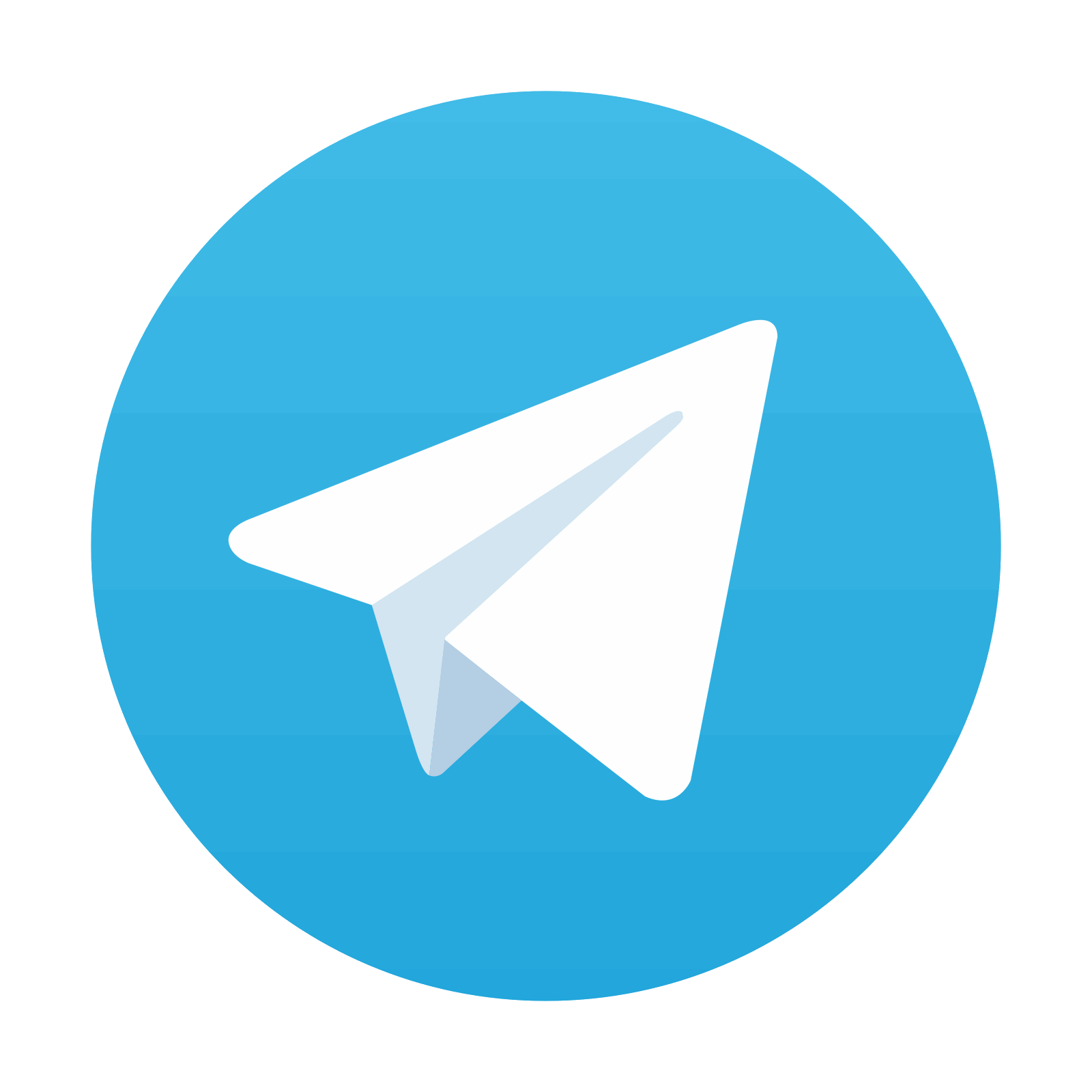
Stay updated, free dental videos. Join our Telegram channel

VIDEdental - Online dental courses
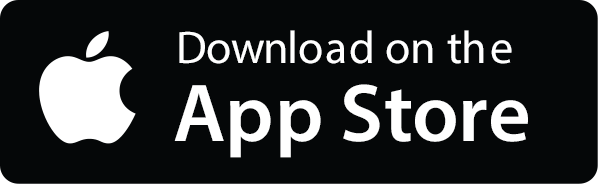
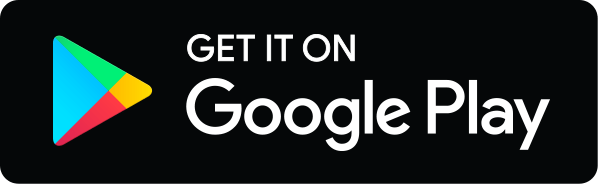
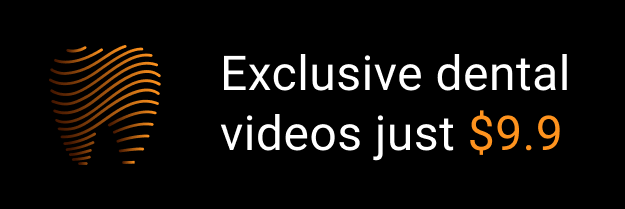