11
Advances in Materials and Techniques for Microbial Control
Ronald Ordinola- Zapata, Maria T. Arias-Moliz, Prasanna Neelakantan, Alejandro Perez-Ron, and Joseph T. Crepps
Summary
The prognosis of endodontically treated teeth depends on, among other things, the proper disinfection of the root canal system. The removal of bacteria, toxins, and other inflammatory by-products allows the healing of the periapical tissues. This chapter discusses contemporary methods used to remove or inactivate the bacterial load of the root canal space.
11.1 Introduction
Inflammation and subsequent necrosis of the pulp is caused by microorganisms that gain access to the root canal system as a consequence of several factors ranging from traumatic injuries, caries, cracks in teeth, lateral canals, and iatrogenic exposures of the pulp tissue. The root canal space is a naturally sterile environment, and, consequently, microbial contamination of the pulp tissue will lead to the development of inflammatory changes. Several vascular and immunologic defence mechanisms are active in pulp tissue in order to create barriers to delay the progression of bacterial dentinal infections and to dilute the inflammatory exudate and bacterial toxins [1, 2]. This includes the innate immune system and the presence of lymphatic vessels and microcirculatory mechanisms to maintain tissue pressure and modulate inflammation. If the microbial insult is not removed, the pulp becomes irreversibly inflamed, eventually leading to the development of pulp necrosis and the progression of inflammation to the periapical area.
Like many other infections in the oral cavity, primary endodontic disease has a polymicrobial component. This involves the presence of facultative and anaerobic bacteria from various species including gram-negative anaerobic and facultative gram-positive bacteria [3]. It is generally accepted that infection of the root canal systems meets the morphological and pathogenic characteristics of a biofilm mediated disease [4–6]. These microorganisms, when observed under the optical or transmission scanning electron microscope, are organised in several layers of cells [7], that are able to induce pathological changes within the periradicular tissues in the form of granulomas, apical abscesses, or cysts [8]. Riccucci et al. [4] reported that apical periodontitis fulfills the conditions proposed by Parsek and Singh [9], which defined the following criteria: the bacteria must be associated with the substrate; the bacteria must be immersed in a matrix produced by the microorganisms themselves or the host elements; and the infection is localised in nature and resistant to antibiotics. Remarkably, the elimination of these bacterial structures can lead to the remission of the periapical disease [10, 11].
11.2 Biofilms
In vitro and laboratory experiments have used planktonic bacteria from aerobic or anaerobic species to demonstrate the antimicrobial activity of numerous irrigating solutions such as cetrimide [12, 13], chlorhexidine (CHX) [14], sodium hypochlorite (NaOCl) [14], and iodine potassium iodide (IKI) [15]. However, considering the mode of growth of biofilms in root canals, it has been suggested that testing antimicrobials against planktonic bacteria may not reflect the effectiveness of the solutions when used for actual endodontic purposes in human teeth [16]. For more realistic experimental models, polymicrobial biofilms formed from subgingival plaque are commonly used to test endodontic irrigants in vitro as they generate a more realistic demonstration of the antimicrobial ability of irrigating solutions [17, 18] (Figure 11.1).

Figure 11.1 (A) Biofilm infected dentine. The effect of 5 minutes of 1% NaOCl can be observed in (B) completely dissolved biofilm by the effect of NaOCl; (C) viable bacteria can still be observed inside dentinal tubules. Red-stained bacteria are cells with damaged membranes and green-coloured bacteria are cells with intact membranes after LIVE/DEAD staining.
Previous studies and reviews in the microbiological field have reported that a large percentage of cells within biofilms are not resistant to antimicrobial chemical agents compared to the planktonic mode. When biofilm cells are detached, antibiotic agents can eliminate them [19], although the presence of persistent microorganisms has also been reported [20]. Biofilm resistance has also been attributed to other factors such as:
- reaction of the antimicrobial components with organic components of biofilm where the antimicrobial effect is consumed by the reaction [21]; and
- greater resistance of cells in the deep layers of the biofilm due to the limited diffusion of the antimicrobial agent within their organic structure [21].
The neutralizing activity of organic material such as bacterial cells or extracellular polymeric substance (EPS) on antimicrobial agents is well-documented [13, 22, 23]. Despite the fact that 90% of EPS is water, the presence of polymers, cells, proteins, or DNA acts as a dispersive barrier to antimicrobial agents, which leads to decreased antimicrobial activity [21]. Therefore, cells within the biofilm are expected to be exposed to different concentrations of the antimicrobial agent. This fact depends on their spatial location [20, 24]. This theory is widely accepted in biofilm research [24], and may explain the irregular pattern of antimicrobial activity found with several antimicrobial agents used in root canals such as CHX or iodine solutions, which affect numerous areas of the biofilm but have no effects in other areas. Another reason may be that the contact time was inadequate to allow better distribution of antimicrobials within the biofilm.
It is also accepted that biofilms found in deep layers are in an altered state of metabolism and are more challenging to eradicate with antibiotics [20, 25]. Mathematical models in the biofilm area reveal two stages in its chemical dissolution [26]. The first step promotes rapid dissolution followed by a long period of time to remove the thin residual layers that remain near the substrate. It has also been reported that disorganisation velocity decreases as biofilm thickness decreases [26]. Similarly, a high viability of bacteria in dentine treated with 5.25% NaOCl has been reported [27]; however, this high viability is coupled with a reduced biomass near the dentinal interface.
11.3 Sodium Hypochlorite
More than 5 decades ago, Grossman [28] stated that the principles underlying the treatment of teeth with necrotic pulps should be the same as those that apply to surgery: ‘an aseptic technique, mechanical debridement of the wound, drainage and gentle handling of the tissues with both instruments and drugs are the cardinal principles of surgery.’ The use of NaOCl as the main root canal irrigant was popularised during the 1940s. Household bleaching solutions containing a 5% concentration were recommended because of their ability to dissolve pulpal tissue compared to other irrigants previously used such as sulphuric acid, hydrochloric acid, sodium hydroxide, hydrogen peroxide, and potassium hydroxide [29].
NaOCl is an organic solvent (pH>11) that can degrade fatty acids and neutralize amino acids forming water and salt. The antibacterial action of NaOCl is due to its effect on bacterial essential enzymatic sites promoting irreversible inactivation originated by chloramination and by the hydroxyl ions (high pH) [30]. In a study on the effect of NaOCl on biofilm architecture, it was reported that a dense bacterial mass and a low water content were able to block deeper transport of solutes, thereby limiting NaOCl diffusion [31]. In a subsequent study, the same authors found that biofilm disruption by NaOCl arguably represents an intermediate stage before biofilm dissolution appears. By increasing the contact time between the biofilm and NaOCl, the disrupted layers had a tendency to increase facilitation of the subsequent dissolution process. The authors recommended flushing the root canal system with a larger NaOCl volume in order to increase the reactivity [32].
Typically, the antimicrobial action of NaOCl in laboratory studies has been tested using extracted teeth, various biofilm models, and dentine block models. The latter allows for an investigation into the depth of dentinal disinfection. Numerous conclusions regarding dentinal tubule disinfection have also been obtained from past experiments including the ability to kill planktonic bacteria even at low concentrations, dissolve biofilm architecture, remove or inactivate bacterial virulence factors, and dissolve the organic content of the necrotic pulp from the root canal system [30, 33, 34]. Retamozo et al. [35] reported that it takes 40–60 minutes of exposure to 5.25% NaOCl to obtain a negative culture in dentine previously infected by the bacterium Enterococcus faecalis. This information confirms the difficulty that can be encountered in decontaminating the deep layers of biofilm present inside the dentinal tubules.
NaOCl also has additional advantages: it has a low surface tension, does not interfere with the repair of periapical tissues, does not stain the tooth structure, is nonantigenic, is safe when used properly within the root canal space, and is inexpensive [30]. On the other hand, NaOCl is not able to remove the inorganic component of the smear layer.
The antimicrobial reduction ability of endodontic procedures has been evaluated in a substantial number of publications using the classic culture anaerobic technique or molecular methods. Laboratory research also helped to elucidate the advantages and limitations of current antimicrobials used during root canal treatment. However, decontamination of the infected root canal system is a phenomenon that relies on several factors:
- the quality of the mechanical and chemical canal debridement [36];
- the presence of vital pulp or necrotic tissue in the apical third of canals;
- the anatomical complexity of the apical third of the root including the transverse section of the root canal at this level, the presence of multiple accessory canals (including apical deltas), the presence of apical root resorptions; and
- the virulence of the microbial community among others [1].
The presence of extraradicular infection can also affect profoundly the clinical outcome [36].
To kill bacteria and biofilms, antimicrobial irrigants and medicaments need to be safely delivered to the cleaned and shaped apical third for a sufficient period of time that allows for the killing or inactivation of the root canal microorganisms [37]. Failure to clean or remove the infection from this critical area may occur as a result of the lack of NaOCl diffusion within the necrotic tissue, inadequate volume, insufficient contact time of the irrigant solution, or the presence of intracanal inactivators such as exudates, blood, dentine debris, or organic tissue.
Clinical data [38] demonstrated that canal instrumentation and irrigation using distilled water within single-rooted teeth with a single canal and necrotic pulps reduced the number of intracanal bacteria in at least 50% of the cases. In the other half, the infection persisted despite continuous treatment in four successive appointments. Interestingly, the concentration of bacteria found preoperatively did not change after the four visits used in those cases. In a subsequent clinical study [39] using the same experimental model, the authors compared the antimicrobial efficacy of 0.5% NaOCl, 5% NaOCl, and a protocol that included irrigation with 5% NaOCl, 10 min of 17% Ethylenediaminetetraacetic acid (EDTA), and 5 min of 5% NaOCl in single-rooted teeth. Twenty patients were treated for each protocol. The samples taken at the second visit revealed that bacteria were recovered in twelve, ten, and eleven patients treated with the different protocols, 0.5%, 5%, and 5% plus EDTA, respectively. The canals were reinstrumented and irrigated and at the third visit eight, six, and three cases had residual intracanal bacteria. The authors concluded that there was no difference between the two NaOCl concentrations and that the use of 5% NaOCl and EDTA appeared beneficial for the disinfection of the root canal space.
Another interesting series of clinical experiments addressing the antimicrobial efficacy of different instrumentation and irrigation protocols were published between 1998 and 2005 [40–43]. In the first study [40], the effect of nickel-titanium rotary instrumentation on bacterial reduction within teeth with necrotic pulps was compared with the conventional step-back technique using hand files. This protocol aimed to observe differences between both instrumentation techniques, and the authors decided to use sterile saline as the main intracanal irrigant. The results revealed that both instrumentation techniques were equivalent regarding bacterial reduction. Of 48 individuals that were recruited for this study, 13 were associated with no quantifiable bacteria after the instrumentation procedure whereas 35 cases still contained bacteria after instrumentation. The study stressed the necessity of using chemical adjuncts to increase bacterial reduction in cases with necrotic pulps. The second study [41] included the use of an antimicrobial irrigant (1% NaOCl) and an intracanal dressing (calcium hydroxide). The microbiological samples were obtained from forty-two individuals after the canals were enlarged to different apical sizes ranging from 20 to 35 and irrigated with 1% NaOCl. In addition, the canals were medicated with calcium hydroxide for at least 7 days. Using this protocol, 61% of the cases were bacteria-free at the end of instrumentation and 1% NaOCl irrigation. Furthermore, when calcium hydroxide medication was used, 92% of the cases did not have any bacteria growth. The results revealed that the apical size of the preparation and the use of the intracanal medication contributed to the reduction of the microbial load. Following the rationale of using large apical sizes, another study was conducted in 40 patients with radiographic evidence of apical periodontitis [42]. Microbiological sampling was performed before treatment, after instrumentation with a number 7 ProFile Series 29 instrument (Dentsply Sirona, York, PA, USA) and after apical instrumentation with LightSpeed instruments (Kerr Dental, Pittsburgh, PA, USA) (apical diameters 0.70 and 0.90 mm); 1% NaOCl was used through the root canal procedures. Molars were instrumented to size 60, canines and premolars to size 80. The results revealed that 100% of canals in cuspids and bicuspids had a negative culture after the first instrumentation. Instrumentation in molars using Lightspeed instruments improved negative cultures to 80%. A more recent study in single-rooted teeth supported these findings [44].
One question that researchers have tried to answer is whether a minimal apical size can be compensated with the use of a high concentration of NaOCl during irrigation. McGurkin-Smith et al. [43] conducted a clinical study in 31 patients who had teeth with necrotic pulps. The instrumentation protocol included the use of Greater Taper Nickel Titanium (GT NiTi) files (Dentsply, Tulsa Dental Specialties, Tulsa, OK, USA), and 5.25% NaOCl irrigation; calcium hydroxide was used as intracanal dressing. The results revealed that bacteria were present in 93% of the samples before treatment and that after canal instrumentation and irrigation with the full strength NaOCl irrigation, 52% of the cases had remaining bacteria. The use of calcium hydroxide as an intracanal medication reduced the number of positive cultures to 14%. The results suggested that instrumentation and irrigation using 5.25% NaOCl failed to render the canals bacteria free in almost 50% of the cases and that intracanal bacteria were significantly reduced using calcium hydroxide intracanal medication.
Even though these reports confirmed the benefits of antimicrobials on the reduction of the intracanal microflora, it is important to note that the long-term outcome can be influenced by individual variables that include:
- the complexity of the root canal anatomy [6];
- the virulence of the intracanal microbiota [45–47]; and
- the immunological status of the host [48] among others.
In addition, several nonendodontic factors can also affect the final outcome such as the overall health status of the patient, the periodontal condition, the amount of coronal destruction that can predispose the tooth to cracking and vertical root fractures, and the quality of the coronal restoration.
11.4 Detoxification of the Root Canal System by Endodontic Procedures
Microbiota in root canals are organised as complex communities termed biofilms. Both the microbial content and the extracellular polymeric matrix content of these biofilms contribute to the inflammatory response, resulting in the development of or sustaining apical periodontitis [4]. Despite the wide variation in the microbiome of infected root canals, the toxic products of bacteria including lipopolysaccharides (LPS/endotoxin) from gram-negative bacteria and lipoteichoic acid (LTA/exotoxin) from gram-positive bacteria contribute to apical periodontitis.
Lipoteichoic acid, the major cell wall constituent of gram-positive bacteria, has been demonstrated to contribute to inflammatory responses and chemokine production, in addition to potentially contributing to a downregulation of host inflammatory responses [49, 50]. Indeed, the exact implications of the role of LTA on persistent apical periodontitis remains unclear. More recently, a significant upregulation of the expression levels of the inflammatory cytokine iNOS following infection of mouse macrophages (RAW264.7 cell line) with live or heat-killed E. faecalis cells has been demonstrated [50]. Interestingly, this infection model also stimulated phagocytic activity by macrophages. Thus, it appears that when osteoclast precursors are infected by E. faecalis, the infected cells may be maintained in the inflammatory system, thereby inhibiting its differentiation into mature osteoclasts. However, the simultaneous upregulation of inflammatory cytokines is likely to contribute to osteoclast differentiation.
On the other hand, LPS, which is a component of the outer membrane of gram-negative bacteria, mediates tissue destruction and enhances pain sensations in root canal infections [51, 52]. LPS is secreted in vesicles by growing gram-negative pathogens or it is released when these bacteria disintegrate following cell death. In fact, there is strong evidence to demonstrate a correlation between LPS and apical periodontitis in teeth with primary endodontic infections [53–57]. Furthermore, greater LPS content has been identified in primary infections, compared to secondary infections [58]. Gomes et al. [58] suggested that it is likely that even nanomolar concentrations of LPS are detected by the innate immune system receptors on macrophages, indicating that they are rather strong stimulators of inflammatory responses.
Bacterial LPS is composed of 3 domains: lipid A, core, and O-antigen repeat [59]. Lipid A, the highly conserved domain in LPS, is recognised by Toll-like receptor 4 to stimulate a robust proinflammatory response, facilitating bacterial clearance from infected host tissues [60]. Greater content of LPS has also been correlated with the size of bone destruction and specific clinical features of primary endodontic infections [58]. Rather interestingly, it has also been shown that root canal LPS and the associated chronic apical periodontitis link strongly to depression and a lowered quality of life by activating the oxidative and nitrosative (O&NS) pathways [61]. Given this critical importance of the toxins, it is imperative that root canal debridement strategies are able to eliminate or at least neutralize these toxins.
There is evidence to show that neither 2.5% NaOCl nor 2% CHX are able to eliminate LPS from primary infected root canals, although sodium hypochlorite reduced LPS content significantly more compared to CHX [62]. These results were also supported in a systematic review [63], which reported the efficacy of sodium hypochlorite and CHX to eliminate LPS from infected root canals. This review was based on four studies [62, 64–66], which were subjected to a meta-analysis. Despite the heterogeneity in the study design, the authors were able to conclude that sodium hypochlorite reduced LPS levels compared to CHX treatment. However, the authors also concluded that both sodium hypochlorite and CHX were unable to eliminate LPS from root canals. Important insights are to be gleaned from this review. First, the highest concentration of NaOCl used in any of these studies was 2.5%. Thus, it is unknown if higher concentrations of NaOCl can eliminate endotoxins. An in vitro study demonstrated that 5.25% NaOCl may be able to induce the loss of the lipid A and eliminate LPS of Fusobacterium nucleatum [67]. Thus, at this point, it appears that none of the irrigants used in endodontics are able to eliminate endotoxins and that sodium hypochlorite likely shows a concentration-dependent effect in neutralizing these toxins. Interestingly, photoactivated chitosan nanoparticles have been shown to significantly inactivate endotoxins and reduce inflammatory markers released by activated macrophages [68]. Such neutralization of LPS infected root canals was reported to support the growth of neotissue without signs of inflammation or resorption [69]. Thus, effective inactivation of LPS may play an important role in regenerative endodontic procedures.
While it is assumed that mechanical instrumentation may play a role in the elimination of endotoxins from root canals, there appears to be no significant difference in the type of instrument or the kinematics on endotoxin removal [70, 71]. A systematic review [71] included three studies that compared rotary and reciprocating systems in eliminating endotoxins from root canals and concluded that all instruments significantly decreased endotoxin content compared to the baseline (pre-treatment), and there was no significant difference between the instruments used.
Several laboratory studies have demonstrated the potential of calcium hydroxide medication to inactivate the LPS molecule [72–74]. Using an animal model [75] Nelson-Filho and colleagues reported that LPS did not induce periapical inflammatory reactions in dog’s teeth when the LPS was mixed with calcium hydroxide and placed inside the root canals. On the other hand, when the canals were filled with the endotoxin alone, it induced a severe inflammatory process including resorption of the apical cementum and extensive areas of resorption. Following the same methodology [76, 77], another study evaluated the ability of three concentrations of NaOCl (1, 2.5, and 5%) and 2% CHX to detoxify the root canal. The results revealed that only canals medicated with calcium hydroxide were associated with absent or mild inflammatory infiltrate at the apical third, normal periodontal ligament thickness and low degree of cementum resorption.
11.5 Inactivation of Root Canal Irrigants
NaOCl has a well-recognised antimicrobial activity in vitro, although in some cases it fails to provide complete bacterial eradication under clinical conditions [38, 39, 78]. Several hypotheses have been explored in order to explain the in vivo efficacy and shortcomings of this solution. The limitations of classic needle irrigation appear to be associated with a physical limitation in which the liquid fails to diffuse into the apical third or the lateral components of the root canal system. The second explanation is associated with the presence of a complex chemical environment, which contains a mixture of organic and inorganic products that can consume the chlorine. Inactivation products include the necrotic pulp itself, periapical exudate, pus, bacteria biofilms, and dentine debris [79]. The interaction of the root canal irrigant with all of these components determines the clinical antimicrobial action of the irrigant solution.
The antimicrobial and tissue-dissolving activities of NaOCl solutions depend on their free available chlorine, which are hypochlorous acid and the hypochlorite ion [30]. These molecules are consumed as the NaOCl exerts its biological activity. The action of root canal instruments on the dentinal walls also generates a smear layer and the subsequent accumulation of dentinal hard tissue debris into non-instrumented areas such as lateral canals, fins, and isthmuses [80]. The presence of dentine debris and a smear layer has been shown to exert an inhibitory effect on the antimicrobial and chemical properties of NaOCl [15, 79, 81–83].
The effect of dentine debris on antimicrobial activity is correlated to NaOCl concentration and contact time. When mixed with dentine, NaOCl solution can lose its chlorine concentration during the first minute of contact significantly [79]. The smear layer, which can have an organic component, also reduces the effectiveness of NaOCl in disinfecting E. faecalis contaminated dentinal tubules [82, 84]. In fact, when the antimicrobial solutions are alternated with a chelating agent, the killing of bacteria inside dentinal tubules is superior compared to the absence of a smear layer removal irrigant [82, 83]. These results confirm the importance of eliminating the smear layer and dentine debris in order to optimise the effect of antimicrobial irrigants.
Dentine debris also decrease the tissue dissolution capacity of NaOCl [85–87]. This effect is also a consequence of the consumption of free available chlorine. It is important to note that when the NaOCl irrigant is in contact with dentine or organic debris, the antimicrobial property is less affected than the dissolution effect, possibly because the irrigant solution is still able to kill some bacteria even when used at low concentrations [87]. As the action of the NaOCl progresses, a loss of chlorine concentration leads to the weakening of the solution. This interaction might be different in the main root canal where the bacteria are mainly organised in biofilms rather than in the dentinal tubules [7] (Figure 11.2). The activity of NaOCl has been shown to be lower in dentine tubules than in surface biofilms, which can be not only a consequence of solution inactivation but also because of the difficulties of penetrating inside these areas. It is also important to mention that the NaOCl and EDTA interaction leads to the loss of tissue dissolution ability. If both solutions are combined, EDTA can consume the free-chlorine; thus, both solutions should be use separately [88, 89].

Figure 11.2 Inhibitory effect of dentine debris on the organic tissue dissolution and available free chlorine at baseline, 1, 3 and 10 minutes. (A) Enterococcus faecalis biofilm, (B) after treatment with 1% NaOCl, and (C) after treatment with 1% NaOCl in the presence of dentine debris. (D) Red-stained bacteria are cells with damaged membranes and green-colored bacteria are cells with intact membranes after LIVE/DEAD staining (BacLigth; Invitrogen, Eugene, OR, USA) under confocal laser scanning microscope (CLSM). Each image represents an area of 317 × 317 µm. Reproduced from Tejada et al. [ 87] / with permission from John Wiley & Sons.
11.6 Etidronic Acid and the Continuous Chelation Concept
As mentioned before, clinical studies have shown that NaOCl irrigation does not prevent the growth of residual microorganisms in root canals [78, 90] and that dentinal debris can reduce the free-chlorine concentration and prevent a proper adaptation of root canal filling materials. From this perspective, eliminating the smear layer is important. As NaOCl does not remove the smear layer, possible alternatives to reduce the microbial load are: a) the use of a chelating solution with antimicrobial activity after the instrumentation;b) the use of a mixture of etidronate and NaOCl, which can maintain antimicrobial capacity and prevent smear layer formation [89]; and c) irrigation with 1–2.5% NaOCl after the use of chelator in order to improve the dissolution capacity of residual biofilms without decreasing the mechanical properties of dentine [91, 92].
Etidronic acid or etidronate (HEDP, tetrasodium 1-hydroxyethane 1,1-diphosphonic acid) is a weak chelating agent that is commonly applied in the cosmetic, food, and detergent industries and in water treatment [93]. It is also a first-generation bisphosphonate used in the treatment of patients suffering from osteoporosis or Paget’s disease [94]. HEDP has an alkaline pH (~11) that favours its mixture with NaOCl [95]. It was introduced in endodontics around 2010 and suggested for use in combination with NaOCl as a single-irrigant solution under the concept of continuous chelation [89]. Continuous chelation simplifies the irrigation protocol and also avoids smear layer formation and hard-tissue debris accumulation in uninstrumented areas of canals with complex anatomy [96, 97]. The association of etidronic acid and sodium hypochlorite has been shown to maintain the chemical properties of NaOCl, including organic tissue dissolution capacity [98, 99] and also presents a strong antimicrobial effect against biofilms attached to the dentine surface or infected dentinal tubules [100, 101]. In addition, these properties are maintained in the presence of smear layer and dentine debris [79, 82, 87]. Thus, the antimicrobial effect is similar to the irrigation protocol that includes NaOCl followed by a final rinse with EDTA, during the chemomechanical canal preparation [82, 102] (Figure 11.3).

Figure 11.3 Etidronic acid for root canal irrigation in its commercial presentation. (A) Representative scanning electron microphotograph of a contaminated block after treatment with a mixture of 2.5% NaOCl/9% Etidronic acid solution (HEBP) in the presence of smear layer (B). No bacteria or smear layer can be observed after 3 min of treatment. Courtesy of Dr María Teresa Arias-Moliz.
HEDP is commercially available for root canal irrigation in the form of capsules containing 0.9 mg of etidronate powder to be mixed with 10 mL of NaOCl solutions at different concentrations (Dual Rinse HEDP; Medcem GmbH, Vienna, Austria). The mixture remains active for 1 hour at room temperature [95, 103]. In addition, cool storing the NaOCl/Dual Rinse HEDP mixtures in a refrigerator keeps the available chlorine almost constant for 7 hours. Heating has a detrimental effect on the available chlorine [103]. Finally, the HEDP does not increase the cytotoxicity of NaOCl and no adverse effects have been observed when tested in vivo [104].
11.7 Cetrimide and Surfactants
Cetrimide (CTR) is a cationic surfactant, which has high bactericidal activity as well as capacity to decrease the mechanical stability of biofilms because it weakens the cohesive forces of the exopolymeric matrix [105]. Several surfactants such as CTR, Tween 80, and cetylpyridinium chloride have been proposed in root canal irrigation. Other irrigants that include surfactants in the mixture are: MTAD (Mixture of Tetracycline isomer, Acid and Detergent) (BioPure MTAD; Dentsply Sirona Endodontics, York, PA, USA) and QMix (Dentsply Tulsa Dental, Tulsa, OK, USA) which is a mixture of an antimicrobial agent (CHX) with a chelating agent (EDTA) and CTR; Tetraclean (Ogna Laboratori Farmaceutici, Muggiò, Italy), which combines doxycycline hyclate, citric acid, and two different detergents (propylene glycol and CTR); and Cetrexidin (Vebas, San Giuliano, Milan, Italy), a mixture of CHX and CTR. MTAD is a mixture of an antibiotic (3% doxycylcline hyclate), citric acid (4.25%), and a detergent (0.5% Tween 80). Other irrigants combine EDTA with surfactant agents, such as SmearClear (SybronEndo, Orange, CA, USA).
Cetrimide has been proposed to be used in combination with other irrigating solutions in order to improve their antimicrobial properties and reduce surface tension. The mixture of CTR and chlorhexidine exhibits a higher antimicrobial activity against planktonic cultures [13, 106, 107] and bacterial biofilms [108–110]. When combined with chelating agents such as EDTA, citric acid, and maleic acid, it improves root canal disinfection [111], creating an extended residual antimicrobial activity [112]. The association of maleic acid with CTR maintains the extraction of calcium ions from dentine [113], thereby assisting to preserve the microstructure of root canal dentine [114], and increases the wettability of an epoxy resin root canal sealer to root canal dentine [115].
Cetrimide can also be found in various commercially available irrigating solutions such as Cetrexidin (Vebas, San Giuliano, Milan, Italy) a mixture of chlorhexidine and CTR that has more residual antibacterial effects (substantivity) and lower toxicity than 5.25% NaOCl [107]; Tetraclean (TC) (Ogna Laboratori Farmaceutici, Muggiò, Italy), a combination of doxycycline hyclate, citric acid, and two different detergents (propylene glycol and CTR) that has been proposed for use as a final rinse after NaOCl solution in root canal treatment because it has antimicrobial activity; however, it does not dissolve organic matter [16]; and QMix (Denstply Sirona, Charlotte, NC, USA) a mixture of chlorhexidine, EDTA, and CTR that has antimicrobial activity [17, 27, 83, 116], substantivity [117], and ability to promote smear layer removal [17].
Even though in theory the surfactant could help the root canal irrigants to distribute throughout the root canal anatomy [118, 119], this property has been questioned [120]. The surface tension only occurs at the interface between two immiscible fluids, which is not the case of root canal irrigants that form a homogenous solution when mixed [121]. Moreover, the interaction between the irrigant and the dentine surface depends on the wettability of the dentine, which is highly hydrophilic and consequently easily wetted by the root canal irrigant [122]. Similar to HEDP, to date there is no clinical studies providing a beneficial outcome on the healing of apical periodontitis.
11.8 Passive Ultrasonic Irrigation
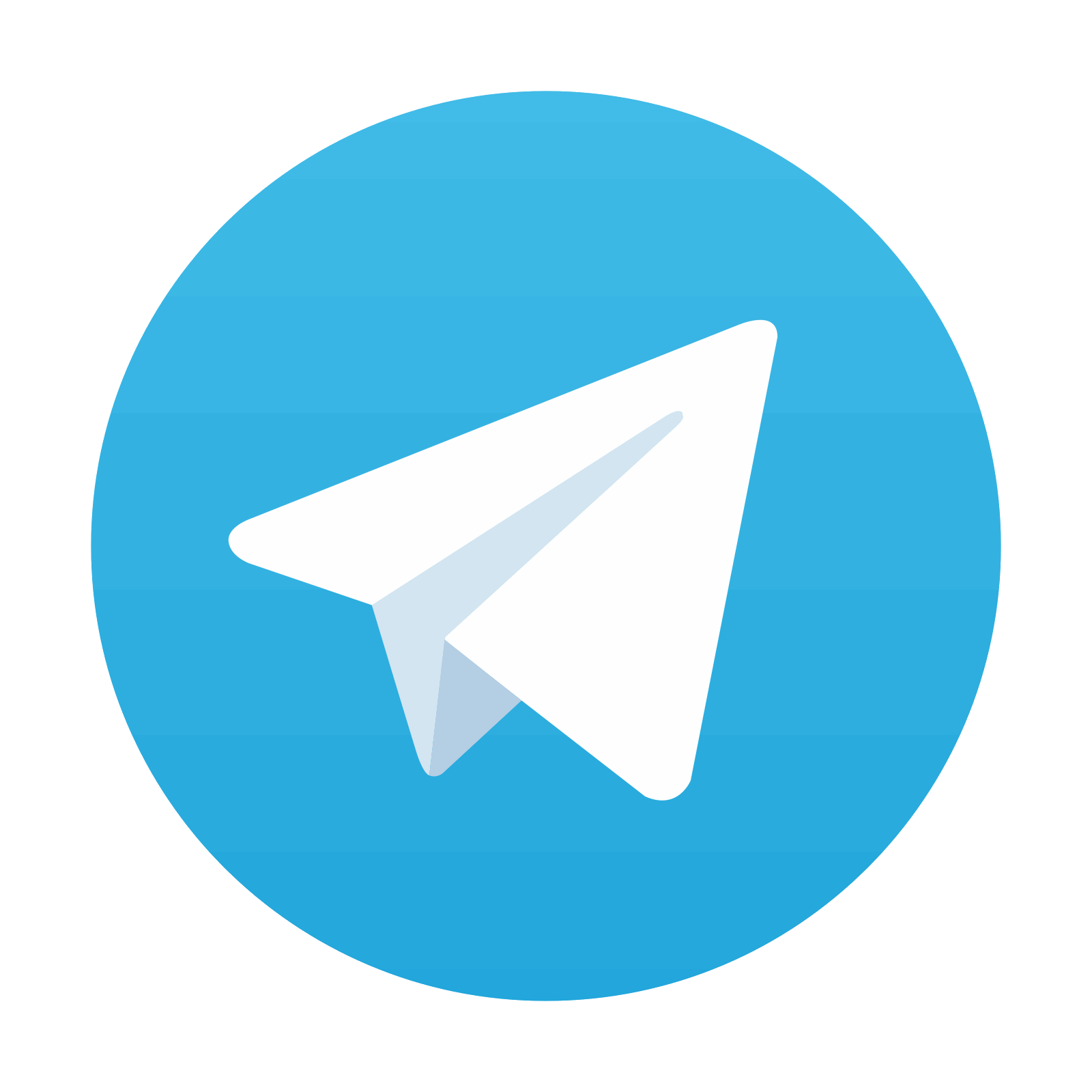
Stay updated, free dental videos. Join our Telegram channel

VIDEdental - Online dental courses
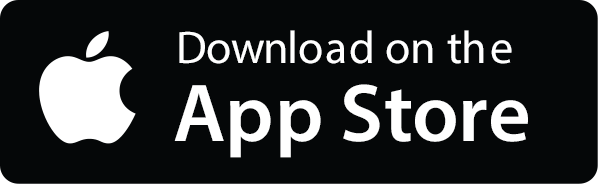
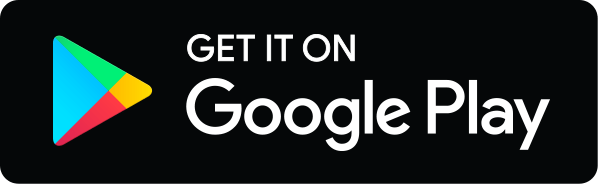