Key points
- •
The use of a cadaver model allows assessment of the effect of individual steps of computer-assisted surgery on orbital implant positioning.
- •
The accuracy and consistency of implant positioning without computer-assisted surgery are low, which enphasizes the need for intraoperative control.
- •
3D virtual surgical planning leads to a better implant position through enhanced insight in anatomy and fracture details, and detailed knowledge on the ideal implant position.
- •
Deviations from the ideal implant position can be identified with intraoperative imaging and the implant’s position can be improved accordingly.
- •
Real-time navigation proves to be the safest, fastest and most accurate method of intraoperative navigation for orbital reconstruction.
Introduction
In most, if not all, clinical research papers and presentations on novel techniques in orbital reconstruction, combinations of different techniques have been used to highlight technological progress and increased quality of surgical outcomes in terms of predictability and reliability. In the Amsterdam University Medical Centers, The Netherlands, a comprehensive research started in 2014 with the development of a human cadaver model for assessment of orbital reconstruction. This model was used in sequence to evaluate every single technological step in the process of orbital reconstruction. This article is a resume of this series of investigations and publications in which the additive value of individual steps used in computer-assisted surgery, including various intraoperative navigation techniques, in orbital reconstruction has been assessed.
The interventions evaluated were as follows:
- 1.
Conventional transconjunctival reconstruction (baseline)
- 2.
Reconstruction with transsinusoidal endoscopy
- 3.
Reconstruction with virtual surgical planning
- 4.
Reconstruction with intraoperative imaging
- 5.
Reconstruction with intraoperative image-guided navigation
- 6.
Reconstruction with intraoperative marker-based navigation
- 7.
Reconstruction with intraoperative real-time navigation
Materials and methods
Materials and methods of assessment were almost identical in all studies. Fixed human cadaver heads were obtained from the Department of Medical Biology, Section of Clinical Anatomy and Embryology of the Amsterdam University Medical Centers. Computed tomography (CT) scans of the cadaver heads were performed at baseline (with intact orbits, T0) (SOMATOM Sensation 64; Siemens Healthineers, Erlangen, Germany). Scan parameters included collimation of 20 × 0.6 mm, 120 kV, 350 mAs, pitch 0.85, field of view 30 cm, matrix 512 × 512, reconstruction slice thickness of 0.75 mm with overlapping increments of 0.4 mm, bone kernel H70s, and bone window W1600 L400. The orbital floor and medial wall were fully exposed through a standard transconjunctival incision and retroseptal preparation. Following the Jaquiéry classification, complex orbital defects (class III–IV) were created with piezoelectric surgery (Mectron SpA, Carasco, Italy).
After creation of the orbital defects (T1), and postoperatively after implant placement (T2), consecutive CT scans were acquired using the aforementioned scanning protocol. After T2 imaging was acquired, the orbital reconstruction plates were removed, and the screw holes were covered with dental filling material (DuraLay; Reliance Dental Mfg Co, Worth, IL, USA) to make them invisible. In all studies, preformed orbital titanium mesh implants (KLS Martin, Tuttlingen, Germany) were used; the stereolithographic models (STL files) of these implants were imported in the iPlan Cranial software environment (version 3.0.5; Brainlab AG, Munich, Germany). The optimal position of the implant was virtually planned and served as the target position during reconstruction. The faces of the human cadaver heads were covered to prevent recognition in consecutive studies, and the various studies were carried out with time intervals of several months to years.
Validation studies were performed to investigate the accuracy and precision of different methods for orbital reconstruction. The acquired implant position was compared with the target implant position of the planning. For this purpose, a reference frame for the preformed orbital implant was created ( Fig. 1 ). This reference frame made quantification of the difference between acquired and planned implant position possible: the individual rotational (roll, pitch, and yaw, in degrees) and translational (in millimeters) deviations served as the outcome parameters for all studies. In studies 1 to 5, 19 orbits were reconstructed by 2 surgeons; 10 orbits were reconstructed by 1 surgeon in study 6, and 20 orbits (of another cadaver group) were reconstructed by 2 surgeons in study 7. The results of studies 2 to 5 were compared with the baseline results (study 1). Studies 6 and 7 were compared with the results of study 5, because image-guided navigation could be considered the reference method for evaluating these enhanced navigation methods.

Conventional transconjunctival reconstruction (study 1)
In the first surgical session, all orbits were reconstructed without further technological additions, with a transconjunctival approach. Preformed orbital implants were positioned according to the best judgment of the surgeon and fixed with osteosynthesis screws. It is important to know that the surgeon had no access to information on the preoperative virtual planning, but only to a multiplanar visualization and 3-dimensional (3D) reconstruction of the CT scan acquired after creation of the orbital defects (T1). The information available to the surgeon and hardware used are visualized in Fig. 2 . This study acted as a baseline study for comparison of implementation of the technologies evaluated.

Reconstruction with transsinusoidal endoscopy (study 2)
The orbital reconstructions in this surgical session were performed with the additional use of a transsinusoidal endoscope. The standard transconjunctival approach was again used to position the implant. To facilitate inspection with an endoscope, a gingivobuccal incision and a 5-mm antrostomy were created in the canine fossa concavity using a Piezotome (Mectron SpA). The sinus mucosa was removed, so the defect and position of the implant could be visualized from the maxillary sinus with a 30°endoscope (KARL STORZ SE & Co KG, Tuttlingen, Germany). In Fig. 3 , the available information during surgery and the hardware used are visualized. The results of this session were compared with the baseline study (study 1).

Reconstruction with virtual surgical planning (study 3)
In the third session, the surgeons had access to a 3D virtual computer environment and to virtual planning tools in iPlan Cranial, such as segmentation and mirroring. Information on the ideal position of the orbital implant, which had been planned with the help of a skilled and experienced technical physician, was available during reconstruction ( Fig. 4 ). The surgeons were challenged to put the implant in the best position according to the virtual plan. The results of reconstruction with preoperative 3D virtual planning were compared with the baseline results (study 1).

Reconstruction with intraoperative imaging (study 4)
An intraoperative CT scan was made after reconstruction of the orbits in this study. A scanning protocol identical to the T0, T1, and T2 studies was used for intraoperative imaging. The implant position was verified by the surgeon on the information seen in Fig. 5 and, if required, the orbital implant position was corrected. The procedure and the scans were repeated until the surgeon was satisfied with the resulting implant position. The results were compared with baseline (study 1).

Reconstruction with intraoperative image-guided navigation (study 5)
All orbits were reconstructed with assistance of intraoperative image-guided navigation (Kolibri; Brainlab AG). The preoperative virtual surgical plan was linked to the intraoperative position of the cadaver head (registration). The cadaver head was tracked using a skull reference marker array (Brainlab AG). After a stable position of the orbital implant was achieved intraoperatively, the navigation pointer was moved along the implant. The result was verified on the multiplanar views: the surgeon could compare the trajectory of the pointer with the contour of the planned position of the implant, which was shown as an overlay ( Fig. 6 ). Results were compared with the baseline study (study 1).

Reconstruction with intraoperative marker-based navigation (study 6)
In this study, intraoperative navigation was again used, but feedback was obtained by indicating orientation markers in the implant design. Three markers were embedded in the preformed implant design, in a triangular fashion ( Fig. 7 ). , The markers were indicated as landmarks in the virtual surgical planning, which allowed comparison between the current position of the marker (indicated with the navigation pointer) and the planned position. This feedback was both visual, to obtain information about the direction of displacement, and quantitative, on the amount of displacement (as seen in Fig. 8 ). The implant-guided navigation workflow results were compared with the results of the image-guided navigation workflow (study 5).


Reconstruction with intraoperative real-time navigation (study 7)
For this study, a real-time navigation workflow was developed and used. An instrument (Titanium Orbital Positioner) was designed that attached to the preformed orbital implant and was compatible with tracking by the navigation system. The cohesion between implant, instrument and navigation tracking allowed calculation of the implant position from the instrument’s position, even during positioning of the implant. Real-time feedback on the current implant position in comparison to the planned position was provided, both visually and quantitatively, and the surgeon could be steered to the correct implant position under navigation guidance. The instrument attachment to the preformed implant and the feedback obtained during positioning are shown in Fig. 9 . The reconstruction was compared with reconstruction with implant-oriented navigation (study 6).

Results
The results are all presented in later discussion in the same manner; statistical workflows, however, differed in some situations because of study design or scientific insights over the years. The outcome of the Orbital Implant Positioning Frame (shown in Fig. 1 ) parameters (roll, pitch, yaw, and translation) are provided in tables, and graphic representation of the mean obtained implant position with each technique (in relation to the planned position) is provided in the figures.
Conventional transconjunctival reconstruction (study 1)
The rotational and translational deviations of the acquired implant position in relation to the planned implant position are provided in Table 1 . The mean and standard deviations of the positioning parameters show that there is minimal control in positioning of the orbital implant: the mean rotation around the z-axis (yaw) is larger than 15° and the mean translation is 5 mm. A maximum value of 47.6° is seen for the yaw. Precision, the consistency of positioning, is also low, as seen by the large standard deviations. Visual appraisal of the average acquired position of the implant ( Fig. 10 ) illustrates the size of the quantitative deviations found. These results underline the need for intraoperative control during reconstruction.
Conventional | ||
---|---|---|
Mean | SD | |
Roll (deg) | −9.8 | 9.1 |
Pitch (deg) | −1.3 | 3.1 |
Yaw (deg) | 17.8 | 10.9 |
Translation (mm) | 5.0 | 2.2 |
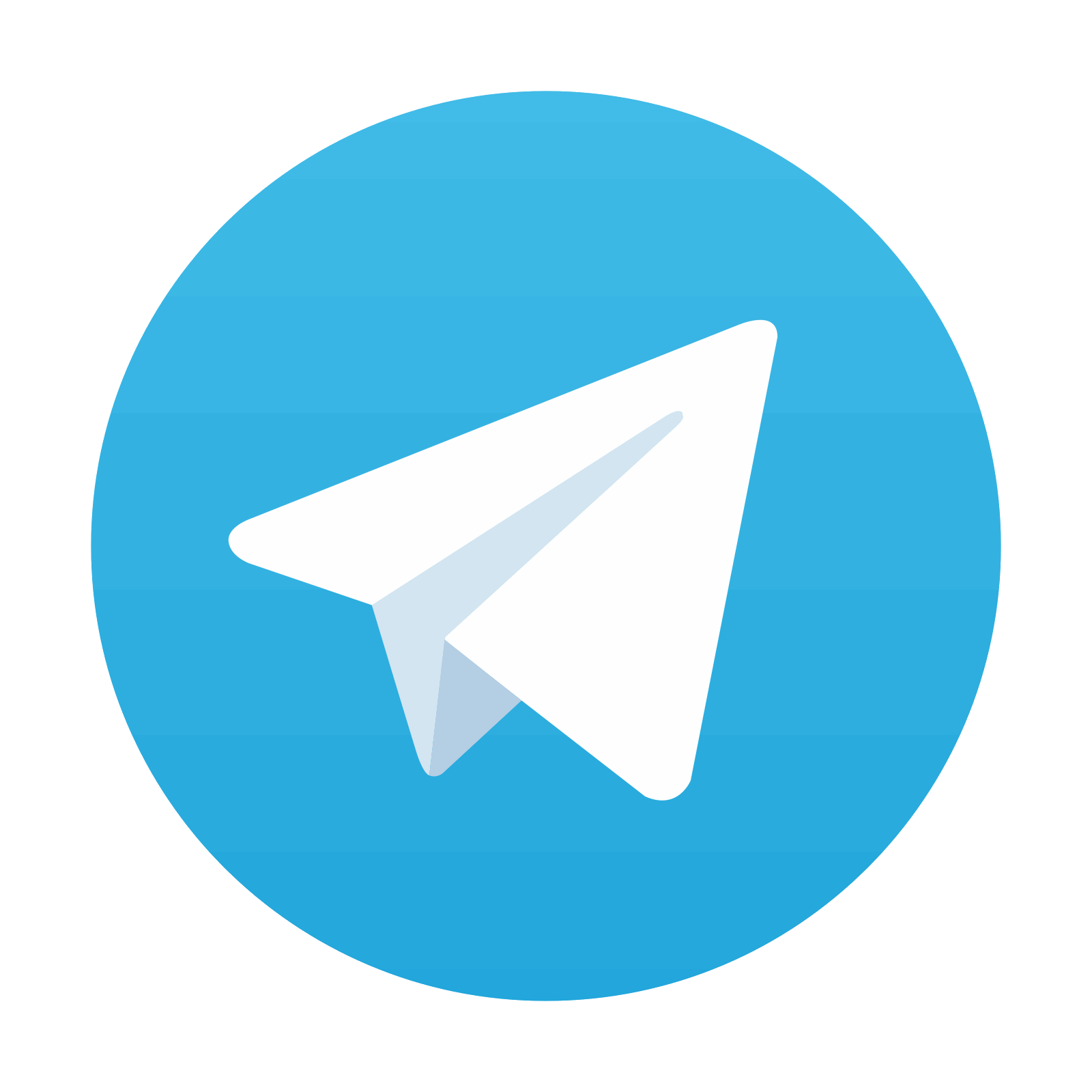
Stay updated, free dental videos. Join our Telegram channel

VIDEdental - Online dental courses
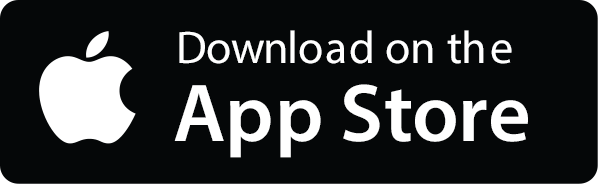
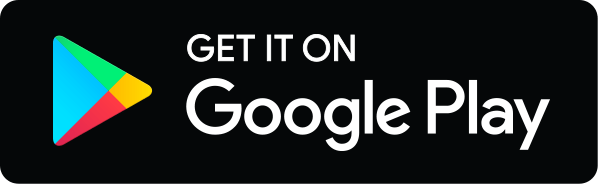
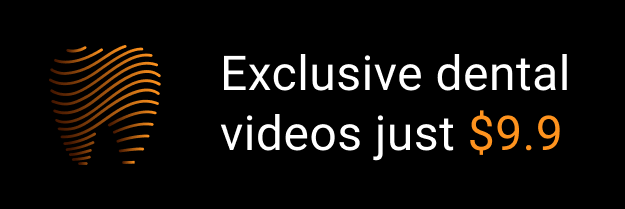