Abstract
Non-silicate ceramics, especially zirconia, have become a topic of great interest in the field of prosthetic and implant dentistry. A clinical problem with use of zirconia-based components is the difficulty in achieving suitable adhesion with intended synthetic substrates or natural tissues. Traditional adhesive techniques used with silica-based ceramics do not work effectively with zirconia. Currently, several technologies are being utilized clinically to address this problem, and other approaches are under investigation. Most focus on surface modification of the inert surfaces of high strength ceramics. The ability to chemically functionalize the surface of zirconia appears to be critical in achieving adhesive bonding. This review will focus on currently available approaches as well as new advanced technologies to address this problem.
1
Introduction
Developments over the last 10–15 years in ceramic materials science for dental applications have led to a class of high strength materials (i.e., zirconia-based ceramics) which potentially provide better fracture resistance and long-term viability when compared to porcelain and other inorganic, non-metallic alternatives. There is a wealth of information in the scientific literature regarding the use of zirconia (ZrO 2 ) in dental applications . Although superior in terms of mechanical performance (strength, toughness, fatigue resistance), there are some inherent problems associated with ZrO 2 . One problem is with adhesion to the variety of substrates (synthetics or tissues) that can be encountered in dental or other biomedical applications. Conventional cementation/attachment techniques used with ZrO 2 components do not provide sufficient bond strength for many of these applications . It is important for high retention, prevention of microleakage, and increased fracture/fatigue resistance, that bonding techniques be improved for zirconia-based systems. Strong resin bonding relies on micromechanical interlocking and adhesive chemical bonding to the ceramic surface, requiring surface roughening for mechanical bonding and surface activation for chemical adhesion. In some instances, high strength ceramic restorations do not require adhesive bonding to tooth structure and can be placed using conventional cements which rely only on micromechanical retention. However, resin bonding is desirable in many clinical situations—e.g., when the prepared tooth structure is unusually short or tapered. In addition, it is likely that strong chemical adhesion would lead to enhanced long-term fracture and fatigue resistance in the oral environment. Non-destructive methods for treating inert ceramics to produce an activated/functionalized surface are desirable in such cases. These methods could also be used in endodontic and implant applications, where ZrO 2 has become a prominent material for fabricating posts and implant components , and where resin-based endodontic filling materials are often employed, and adhesive bonding is desired.
Bonding to traditional silica-based ceramics, generally employing both mechanical and adhesive retentions, has been well researched, and bond strengths are predictable. A strong resin bond relies on chemical adhesion between the cement and ceramic (by way of silane chemistry), and on micromechanical interlocking created by surface roughening. Current roughening techniques are: (1) grinding, (2) abrasion with diamond (or other) rotary instruments, (3) air abrasion with alumina (or other) particles, (4) acid etching (typically HF), and (5) a combination of any of these techniques. Unfortunately, the composition and physical properties of ZrO 2 differ from conventional silica-based materials like porcelain. Zirconia is not readily etched by HF, and requires very aggressive mechanical abrasion methods to increase surface roughness, possibly creating strength reducing surface flaws . Therefore, in order to achieve acceptable cementation in a wide range of clinical applications, alternate attachment methods, ideally utilizing chemical adhesion in addition to mechanical retention, are required for zirconia ceramics. Various approaches to this problem will be discussed in this review.
2
Zirconia as a biomaterial
Zirconium oxide (ZrO 2 ), or zirconia, is a metal oxide that was identified as a reaction product of heating the gem, zircon, by the German chemist Martin Heinrich Klaproth in 1789 . Zirconia is polymorphic in nature, meaning that it displays a different equilibrium (stable) crystal structure at different temperatures with no change in chemistry. It exists in three crystalline forms: monoclinic at low temperatures, tetragonal above 1170 °C and cubic above 2370 °C . A characteristic of this material is that it undergoes a change in crystal structure from tetragonal to monoclinic during cooling, resulting in a volume increase (3–4%) that can induce large stresses. These stresses can produce cracks that result in spallation, crumbing, and failure. Work by Ruff et al. showed that the cubic phase could be stabilized with the addition of small amount of calcia (CaO), making it possible to use ZrO 2 as an engineering material.
Over the years, other metal oxides like cesium oxide (CeO 2 ), magnesia (MgO), and yttria (Y 2 O 3 ) have been used to create a range of partially stabilized zirconia (PSZ) compositions, which are primarily cubic phase at room temperature, with monoclinic and tetragonal precipates as a minor phase . For example, the addition of 8 mol% MgO to ZrO 2 results in the creation of a stable matrix of cubic phase grains, with a metastable phase of tetragonal crystals that precipitate during cooling. In the Y 2 O 3 –ZrO 2 system, the addition of 2–3 mol% Y 2 O 3 to ZrO 2 produces a metastable matrix of tetragonal crystals referred to as tetragonal zirconia polycrystals (TZPs) . PSZ is of particular interest because of its transformation toughening properties . As a crack initiates and propagates in PSZ during application of an external load, the tetragonal phase can transform to a monoclinic phase around and near the tip of the crack. The resulting volume expansion caused by the transformation creates compressive stresses at the crack tip. This actively hinders propagation, because excess energy is now necessary for the crack to continue to propagate, thus increasing PSZ’s resistance to fracture.
PSZs have gained popularity in many industries due to exceptional wear resistance, fracture toughness (7–8 MPa m 1/2 ), and flexural strength (0.9–1.5 GPa) . Because of this, PSZs have been used in blades to cut Kevlar, incorporated into magnetic tape, and as a structural component in a variety of filters . PSZ’s toughness, corrosion resistance, and thermal conductivity make it a good refractory material used in abrasives or as a thermal barrier coating on manufactured parts operating in aggressive environments, like extrusion dyes, valves, and port liners for combustion engines . PSZ is used as a refractory liner or valve part in foundries due to its thermal shock resistance . Its high refractive index and transparency in the visible and near-infrared spectrum make it useful in optical filters, and laser mirrors . It is used in high temperature oxygen separation, oxygen sensors, and fuel cell membranes due to its high ionic conductivity and as an alternative gate dielectric in microelectronics because of its high dielectric constant .
In recent years, ZrO 2 has gained attention as a biomaterial due to superior mechanical properties, compared to alumina (Al 2 O 3 ), and chemical and biological inertness that makes it very biocompatible . Exploration into ZrO 2 as a biomaterial began in 1960s , with most of the work over the years focused on the use of ZrO 2 in orthopedics, specifically in the area of femoral heads for total hip replacements . Recently, ZrO 2 has been introduced in dentistry due to its superior esthetic potential when compared to metal–ceramic constructs. Zirconia has been explored as a dental material for a variety of clinical applications: orthodontic brackets , endodontic posts/dowels and abutments , single crowns and fixed partial dentures .
Bonding to ZrO 2 has become a topic of great interest in recent years . As previously stated, traditional adhesive chemistry is ineffective on ZrO 2 surfaces, since they are essentially non-polar and inert. Additionally, acid etchants like HF do not sufficiently roughen the surface for simple micromechanical attachment. Surface abrasion with Al 2 O 3 particles and application of a tribochemical silica coating allows for chemical bonds to a silane coupling agent and to resin cement. This is a somewhat complicated procedure that does not produce bond strengths as high as those reported for silane bonded porcelain . In addition, there is some speculation that air particle abrasion should not be used, particularly with ZrO 2 ceramics, because it might cause microfractures that would reduce functional strength and lead to premature, catastrophic failure. In vitro analysis has shown that surface flaw generation can reduce the fracture strength of ZrO 2 significantly . The use of phosphoric acid primers or phosphate-modified resin cements has been shown to produce silane-like adhesion, through a similar type of hydroxylation-driven chemistry. However, bond strength values reported in the literature through use of these agents are generally lower than the values reported for tribochemical silica coating, coupled with silane and resin cement. The currently available approaches for adhesive bonding of ZrO 2 bioceramics are not adequate for all clinical applications, and long-term durability is currently unknown . Other hydroxylation approaches are also being developed, but they often utilize very powerful acidic or basic chemistries, and their utility, especially for biomedical application is not yet known .
2
Zirconia as a biomaterial
Zirconium oxide (ZrO 2 ), or zirconia, is a metal oxide that was identified as a reaction product of heating the gem, zircon, by the German chemist Martin Heinrich Klaproth in 1789 . Zirconia is polymorphic in nature, meaning that it displays a different equilibrium (stable) crystal structure at different temperatures with no change in chemistry. It exists in three crystalline forms: monoclinic at low temperatures, tetragonal above 1170 °C and cubic above 2370 °C . A characteristic of this material is that it undergoes a change in crystal structure from tetragonal to monoclinic during cooling, resulting in a volume increase (3–4%) that can induce large stresses. These stresses can produce cracks that result in spallation, crumbing, and failure. Work by Ruff et al. showed that the cubic phase could be stabilized with the addition of small amount of calcia (CaO), making it possible to use ZrO 2 as an engineering material.
Over the years, other metal oxides like cesium oxide (CeO 2 ), magnesia (MgO), and yttria (Y 2 O 3 ) have been used to create a range of partially stabilized zirconia (PSZ) compositions, which are primarily cubic phase at room temperature, with monoclinic and tetragonal precipates as a minor phase . For example, the addition of 8 mol% MgO to ZrO 2 results in the creation of a stable matrix of cubic phase grains, with a metastable phase of tetragonal crystals that precipitate during cooling. In the Y 2 O 3 –ZrO 2 system, the addition of 2–3 mol% Y 2 O 3 to ZrO 2 produces a metastable matrix of tetragonal crystals referred to as tetragonal zirconia polycrystals (TZPs) . PSZ is of particular interest because of its transformation toughening properties . As a crack initiates and propagates in PSZ during application of an external load, the tetragonal phase can transform to a monoclinic phase around and near the tip of the crack. The resulting volume expansion caused by the transformation creates compressive stresses at the crack tip. This actively hinders propagation, because excess energy is now necessary for the crack to continue to propagate, thus increasing PSZ’s resistance to fracture.
PSZs have gained popularity in many industries due to exceptional wear resistance, fracture toughness (7–8 MPa m 1/2 ), and flexural strength (0.9–1.5 GPa) . Because of this, PSZs have been used in blades to cut Kevlar, incorporated into magnetic tape, and as a structural component in a variety of filters . PSZ’s toughness, corrosion resistance, and thermal conductivity make it a good refractory material used in abrasives or as a thermal barrier coating on manufactured parts operating in aggressive environments, like extrusion dyes, valves, and port liners for combustion engines . PSZ is used as a refractory liner or valve part in foundries due to its thermal shock resistance . Its high refractive index and transparency in the visible and near-infrared spectrum make it useful in optical filters, and laser mirrors . It is used in high temperature oxygen separation, oxygen sensors, and fuel cell membranes due to its high ionic conductivity and as an alternative gate dielectric in microelectronics because of its high dielectric constant .
In recent years, ZrO 2 has gained attention as a biomaterial due to superior mechanical properties, compared to alumina (Al 2 O 3 ), and chemical and biological inertness that makes it very biocompatible . Exploration into ZrO 2 as a biomaterial began in 1960s , with most of the work over the years focused on the use of ZrO 2 in orthopedics, specifically in the area of femoral heads for total hip replacements . Recently, ZrO 2 has been introduced in dentistry due to its superior esthetic potential when compared to metal–ceramic constructs. Zirconia has been explored as a dental material for a variety of clinical applications: orthodontic brackets , endodontic posts/dowels and abutments , single crowns and fixed partial dentures .
Bonding to ZrO 2 has become a topic of great interest in recent years . As previously stated, traditional adhesive chemistry is ineffective on ZrO 2 surfaces, since they are essentially non-polar and inert. Additionally, acid etchants like HF do not sufficiently roughen the surface for simple micromechanical attachment. Surface abrasion with Al 2 O 3 particles and application of a tribochemical silica coating allows for chemical bonds to a silane coupling agent and to resin cement. This is a somewhat complicated procedure that does not produce bond strengths as high as those reported for silane bonded porcelain . In addition, there is some speculation that air particle abrasion should not be used, particularly with ZrO 2 ceramics, because it might cause microfractures that would reduce functional strength and lead to premature, catastrophic failure. In vitro analysis has shown that surface flaw generation can reduce the fracture strength of ZrO 2 significantly . The use of phosphoric acid primers or phosphate-modified resin cements has been shown to produce silane-like adhesion, through a similar type of hydroxylation-driven chemistry. However, bond strength values reported in the literature through use of these agents are generally lower than the values reported for tribochemical silica coating, coupled with silane and resin cement. The currently available approaches for adhesive bonding of ZrO 2 bioceramics are not adequate for all clinical applications, and long-term durability is currently unknown . Other hydroxylation approaches are also being developed, but they often utilize very powerful acidic or basic chemistries, and their utility, especially for biomedical application is not yet known .
3
Mechanical bonding
Bonding of ZrO 2 to tooth structure or other substrates requires a strong resin bond. The success of resin bonding relies on mechanical bonding through micromechanical interlocking from surface roughening, and if possible, chemical bonding between ceramic and cement. Phosphoric acid (H 3 PO 4 ) or hydrofluoric acid (HF) etching are commonly recommended methods used to surface roughen silica-based ceramics . This creates a rough, clean surface, which improves wettability and increases surface area available for mechanical interlocking. Unfortunately, H 3 PO 4 and HF cannot be used effectively on non silica-based ceramics, like ZrO 2 , making it difficult to roughen the surface for mechanical retention . The lack of silica also removes the chemical bonding between silica–silane necessary for silanization.
Because of the difficulty in creating mechanical and chemical bonding in ZrO 2 , alternative methods have been explored to bond ZrO 2 using resins. Surface grinding is a commonly used alternative for roughening the surface of ZrO 2 to improve mechanical bonding. There are several methods used for surface grinding: grinding using abrasive paper or wheels (SiC or Al 2 O 3 ), particle air-abrasion using Al 2 O 3 or other abrasive particles ranging in size from 50 to 250 μm , and grinding using a diamond bur . The advantage of these surface grinding methods is that they are generally easy to apply in a dental environment. However, research has shown that surface grinding techniques, using tradition resin cements, have no significant effect on increasing the bond strength of zirconia to resin cements .
Another problem with these techniques is that they can create surface microcracks . These flaws act as crack initiation sites that can decrease strength and apparent fracture toughness. However, surface grinding also results in a tetragonal to monoclinic phase change on the surface of zirconia. This can theoretically produce a compressive stress layer that counteracts the flaw-induced reduction in strength . Work by Guazzato et al. and Kosmač et al. showed that sandblasting produced the most effective tetragonal to monoclinic phase change when compared to fine polishing, grinding with an abrasive wheel, or grinding using a diamond bur . It was determined that sandblasting was able to induce transformation at low temperature, with minimal surface damage. Care has to be taken with the amount of surface grinding, as an excess amount can diminish the strength enhancing effect.
Care also has to be taken when heat treating surface-ground ZrO 2 . It has been shown that heat treatment temperatures for bonding veneering porcelain to ZrO 2 substructures, around 900–930 °C, can cause a decrease in flexure strength. The temperature applied during heat treatments, coupled with existing residual stresses, can sometimes be sufficient to cause a transformation of the monoclinic phase back to the tetragonal phase, which relieves the compressive stress in the surface layer and reduces the flexure strength of ZrO 2 .
The application of fused glass micro-pearls to the surface of ZrO 2 has been shown to increase the bond strength of resin cements to ZrO 2 . In these studies, a slurry of micro-pearls was painted on a ZrO 2 surface and fired in a furnace. The fused glass film increased surface roughness of ZrO 2 , allowing increased micro-retention. The silica-rich film also allows for silanization of ZrO 2 before bonding, making it possible to form siloxane bonds to resin cement. Derand et al. showed that use of this fused micro-pearl film significantly increased the bond strength of ZrO 2 (11.3–18.4 MPa) compared to untreated or silanized ZrO 2 (0.5–1.5 MPa).
A novel surface roughening technique that has been explored for ZrO 2 is selective infiltration etching (SIE) . SIE uses a heat-induced maturation process to pre-stress surface grain boundaries in ZrO 2 to allow infiltration of boundaries with molten glass. The glass is then etched out using HF, creating a 3D network of inter-granular porosity that allows nano-mechanical interlocking of resin cement. The advantage of SIE is that it only involves grains that are exposed to molten glass, allowing control of the area to be etched. Aboushelib et al. showed that using SIE on ZrO 2 resulted in increased microtensile bond strength (49.8 ± 2.7 MPa) when compared to particle air-abraded ZrO 2 (33.4 ± 2.1 MPa). The use of SIE improved nano-mechanical retention of zirconia by increasing the surface area available for bonding. This was confirmed by AFM work done by Casucci et al. showing that the surface roughness of ZrO 2 is significantly greater after SIE, when compared to particle air-abrasion or HF etching.
Recently, another experimental method has been examined for surface roughening of ZrO 2 . The use of a hot chemical etching solution has been proposed to etch the wings of Maryland bridges . Although there has not been any reported research on the bond strength of ZrO 2 to resin cements using this treatment, AFM has shown that the application of hot chemical etching solution produces a surface roughness that is significantly greater than SIE . It is possible that this technique could enhance the mechanical retention of ZrO 2 .
4
Chemical bonding—silane coupling agents
Organo-silanes, generally referred to simply as “silanes” in dentistry, are compounds that contain a silicon (Si) atom or atoms, are similar to orthoesters in structure, and display dual reactivity. Their use in clinical dentistry and affect on adhesive bonding has been described in detail in the scientific literature . One end of a silane molecule is organically functional (e.g., vinyl–CH CH 2 , amino–NH 2 ), and can polymerize with an organic matrix (e.g., a methacrylate). The other end is generally comprised of alkoxy groups (e.g., methoxy–OCH 3 , ethoxy–OCH 2 CH 3 ), which can react with a hydroxylated surface, like porcelain. A idealized schematic of silane chemistry is shown in Fig. 1 . Silanes are commonly used in dentistry to coat glass filler particles in polymer matrix composites, to achieve adhesive bonding of porcelain (or other silica-containing ceramics) to resin luting cements for restorative applications, and with certain ceramic or ceramic-containing composite posts for endodontic applications employing resin-based filling materials. Silanes are also believed to promote surface wetting, which enhances potential micromechanical retention with low viscosity resin cements . Traditional silane chemistry is not truly effective with ZrO 2 , as it possesses a relatively non-polar surface, is more chemically stable than silica-containing ceramics, and not easily hydrolyzed.
Experimentation with different silane coupling agents has resulted in enhancement of luting of ZrO 2 . Matinlinna et al. researched use of three trialkoxysilanes, 3-methacryloyloxypropyl-trimethoxysilane (MPS), 3-acryloyloxypropyl-trimethoxysilane (ACPS), and 3-isocyanatopropyl-triethoxysilane (ICS), in enhancing the bonding of two resin cements, an experimental Bis-GMA and commercial Bis-GMA (RelyX ARC, 3M-ESPE, Seefeld, Germany), to ZrO 2 . They determined that application of a tribochemical coating, followed by silanization with MPS and ACPS, were successful in bonding the two cements to ZrO 2 . It is thought that the RelyX ARC has a coefficient of thermal expansion that matches more closely with ZrO 2 , which results in enhanced bonding. Artificial aging using thermocycling showed that only RelyX ARC was able to maintain its shear bond strength after using MPS and ACPS. The decrease in bonding using ICS is due to the absence of acrylate and methacrylate groups in ICS that could polymerize and bond with the dimethacrylate groups in RelyX ARC. Matinlinna et al. has also worked on a novel silane system, 3-mercaptopropyltrimethoxysilane (MEPS) blended with 1,2-bis-(triethoxysilyl)ethane (BTSE), that has shown promising results. This novel silane system demonstrated similar shear bond strength to MPS under dry conditions on particle air-abraded and silica-coated ZrO 2 . However, after thermocycling, bond strength obtained with the novel silane system decreased significantly and was significantly less that MPS. Further work in optimizing this novel silane system may provide stronger bonding after thermocycling.
Aboushelib et al. showed that application of silane alone resulted in low bond strength. The use of five silanes (MPS, ACPS, and ICS along with styrylethyltrimethoxysilane and 3-( N -allylamino)propyltrimethoxysilane) to aid in luting of as-received ZrO 2 resulted in bond strengths that were significantly less than when using the silanes on SIE ZrO 2 . It was shown that MPS produced greater bond strength when used on SIE ZrO 2 compared to the other silanes. However, bond strength of SIE ZrO 2 using the silanes decreased significantly after long-term storage . This decrease in bond strength demonstrates that use of silanes does not aid in producing a hydrolytically stable bond with ZrO 2 . Although bond strength decreased after time, SIE does create a retentive surface for mechanical bonding. It is also possible that SIE could chemically modify the surface to improve bonding between the silane and ZrO 2 .
Alternatively, the use of a zirconate coupling agent has been explored for pure zirconium and ZrO 2 . The ZrO 2 coupling agent acts like a silane coupling agent by bonding ZrO 2 with hydroxyl groups. This coupling agent enhanced bonding to resin cements but exhibited a significant decrease in bond strength after thermocycling.
5
Primers—silica coating
Due to the lack of silica in ZrO 2 , silica-coating techniques have been explored to utilize the chemical bonding provided by silanization. The use of a tribochemical silica coating is a common practice for coating metal alloys and alumina- and zirconia-based dental ceramics with silica with the CoJet and Rocatec systems (3M ESPE, Seefeld, Germany) being the most heavily favored commercial products utilized for applying the coating. The tribochemical technique air-abrades the ceramic surface with alumina particles that have been coated with silica, embedding/coating the surface with silica . This results in not only preparing a surface for silanization, but also creates micromechanical retention ( Fig. 2 ). Research has shown that the application of a tribochemical coating, before silanization, significantly enhances bond strength between a treated substrate and resin cement. However, there can be significant loss in bond strength over the long-term when using traditional resin cements used for silica-based ceramics. This might be a result of a low concentration of silica on the surface due to difficulty in particle abrasion caused by the high hardness of ZrO 2 .
Cleaning of a tribochemical coated ZrO 2 before resin bonding can be deleterious to bond strength. Nishigawa et al. determined that ultrasonic cleaning of tribochemical coated ZrO 2 before silanization and resin bonding significantly decreases bond strength. Cleaning for 2 or 5 min resulted in bond strength statistically similar to air-abrading alone before bonding. It was shown that ultrasonic cleaning results in decreased silica content on the surface. This decrease, along surface morphology changes, is thought to cause the loss of bond strength. Air pressure has also been shown to affect bond strength when applying a tribochemical coating. Heikkinen et al. determined that increasing the air pressure at which tribochemical coating is applied significantly increases the bond strength of ZrO 2 to resin cement. It was also determined that increasing pressure increased the amount of silica on the ZrO 2 surface. It is thought that increasing air pressure, which increases kinetic energy of particles, causes an increase in surface roughness and the number of particles that contact the surface. This increases mechanical retention the amount of silica available for chemical bonding.
Another method that had been used to apply a silica layer to ZrO 2 -based ceramics is silicoating . Silicoating involves pyrolytically applying a silica coating on a substrate surface, followed by application of silane, before bonding using a resin cement . Using a lab device, butane gas is burned with atmospheric oxygen and guided over a container filled with tetraethoxy silane. The gas is lit and the silane decomposes in the flame, coating the material with a layer of SiO x –C fragments that bond adhesively to the surface of the material . Silicoating, marketed under the Silicoater-Technology (Silicoater, Kulzer Co., Friedrichshof, Germany), has been successful in improving the bond strength of resin cements to metals and decreasing the degradation of bond strength after thermocycling . However, it was expensive and too complex to be commercially viable for standard dental applications.
Recent innovations in silicoating, i.e., the PyrosilPen-Technology (PyrosilPen, SurA Instruments, Jena, Germany), have made it easier to use for chair-side applications. Application of the PyrosilPen-Technology had been researched on ZrO 2 -based ceramics . Although there was no significant difference in bond strength, the ZrO 2 -based ceramics had lower bond strength than that of silicoated silica- and alumina-based ceramics. This could be due to a lack of micromechanical bonding since the surface of the ceramic was only abrasively ground using 800 grit grinding paper . It is possible that bond strength could be improved if the surface was particle air-abraded before silicoating. Further researcher is required before the PyrosilPen-Technology can be used as an acceptable method to enhance bonding of ZrO 2 to resin cements.
Another form of silicoating uses a plasma spray technique to deposit a siloxane coating on ZrO 2 . Derand et al. used a high-frequency generator to deposit hexamethyldisiloxane (HMDS) on the surface of ZrO 2 . When polymerized, HMDS acts like a silane, resulting in a siloxane bond between ZrO 2 and resin cement. The application of the plasma produced a bond strength that was significantly greater than untreated or silanized ZrO 2 but was significantly less than ZrO 2 coated with a film of porcelain micropearls. Using air-abrasion or surface grinding to increase mechanical retention could increase bond strength of plasma coated ZrO 2 .
Recently, another method has been proposed to apply a silica layer to the surface of ZrO 2 ceramics. Piascik et al. have researched application of a unique vapor-phase deposition technique, in which a chloro-silane gas (SiCl 4 ) is combined with water vapor to form a Si x O y -functionalized surface on a ZrO 2 substrate ( Fig. 3 ). The process uses molecular vapor deposition (MVD), an enhancement on conventional vapor deposition, to deposit ultra-thin, uniform coatings on substrates using an in-situ surface plasma treatment . MVD can produce hydrophobic, hydrophilic, biocompatible, protective, ordering, or otherwise reactive coatings on a variety of materials. The study conducted by Piascik et al. showed that ZrO 2 coated with a Si x O y film, followed by silanization and resin cement bonding, enhanced bond strength. Zirconia with a film thickness of 2.6 nm had significantly greater bond strength than tribochemical-coated ZrO 2 and was comparable to porcelain. The ZrO 2 that was bonded using a 23 nm Si x O y film had a significantly lower bond strength than the 2.6 nm film coated ZrO 2 but greater than untreated ZrO 2 ( Fig. 4 ). Based on these results, Piascik et al. stated that it is possible that bond strength of the Si x O y film is dependent on film thickness. The decrease in bond strength could be caused by a lack of chemisorption in the additional layers deposited on the ZrO 2 surface after the initial deposited layer. This would result in reducing the chemical adhesion between subsequent layers, lowering the bond strength of the resin cement to the film. This would explain the increase in adhesive failure observed for the 23 nm film specimens compared the 2.6 nm film specimens. Further investigation into the optimal film thickness could result in expanding the use of ZrO 2 to more dental applications.
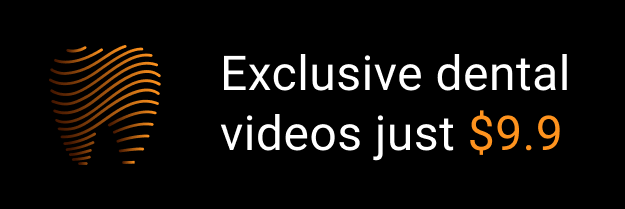