Given the enormity of the field of adhesion and the number of commercial products available, the discipline of modern adhesive dentistry can be daunting with respect to materials and techniques. This article organizes contemporary bonding practice and materials around an understanding of the fundamentals of adhesion to tooth structure. In providing this context, adhesive development, bonding systems, and their appropriate use are better understood. The end result is the better practice of adhesive dentistry.
The achievement of high-strength, durable bonds between tooth structure and restorative materials has been a long-term goal of the dental profession. The formation of such bonds would lead to a true surgical model of operative treatment of teeth and the universal application of minimally invasive dentistry. In principle, operative intervention would only require the removal of the diseased or undermined parts of teeth. Subsequent reconstruction for the long-term would be based on the use of a restorative material retained only by an adhesive system, whether in load or non–load-bearing environments. This approach provides the advantages of (1) work simplification, (2) reduced treatment time, (3) reduced biological cost, (4) an ability to retain restorative materials without any form of retention, including pins and posts, and (5) an improvement in the quality of the interface between materials and tooth walls.
Although it took more than 20 years to become widely accepted, Buonocore established the basis for modern restorative dentistry in 1955, when he described a technique for bonding acrylic materials to enamel using phosphoric acid . More than two decades later, the strength of adhesive joints formed by bonding resins to etched enamel resulted in the widespread use of acid-etching techniques rather than pins for the retention of class IV composite restorations . Thus began the era of adhesive dentistry.
After nearly three decades of experience, adhesive techniques are routinely incorporated into clinical practice. Acid treatment of enamel or dentin, whether an a priori or concomitant bonding step, has been integrated into methods to retain materials and significantly reduce microleakage at material-tooth interfaces . In addition to tooth reconstruction, a multiplicity of adhesive clinical procedures—ranging from fissure sealants to laminate veneering to resin bonded bridges—has been spawned. Little did Buonocore appreciate the impact of his discovery on the quality of care more than 50 years later.
Given the enormity of the field of adhesion and the number of commercial products available, the discipline of modern adhesive dentistry can be daunting with respect to materials and techniques. This article organizes contemporary bonding practice and materials around an understanding of the fundamentals of adhesion to tooth structure. In providing this context, adhesive development, bonding systems, and their appropriate use are better understood. The end result is the better practice of adhesive dentistry.
Adhesion basics
In descriptive terminology, adhesion is the bonding or the attachment of dissimilar materials. When applied to surfaces, adhesives join materials together to resist separation and transmit loads across the bonds. The materials being joined are commonly referred to as substrates or adherends.
Adhesion is not an inherent property of an adhesive. Rather, it is the response of an assembly to deformation loads. In the dental literature, this is often referred to as “bond strength.” When stress is applied to the assembly, energy is absorbed. The adhesive transfers and distributes the stress to the components of the joint. The strain response to the stress is a manifestation of the properties of all the materials that comprise the assembly.
Failure of assemblies (eg, composite-tooth joints) occurs as a result of flaws, which propagate under stress as cracks and can originate in any component of the assembly. The ability of an assembly to resist crack growth is a function of its toughness. Although “bond strength” is commonly used in the dental literature, a load being applied is often attenuated by the adherends before load transfer to the bond. For example, the modulus, or stiffness of those adherends, rather than the strength of the adhesive can vary the result of a “bond strength” test. For conventional tests of dental bonding systems, such as the commonly used shear test, cracks often initiate in one of the adherends. In these cases, although results are often referred to as “bond strength,” the intrinsic strength of the bond layer is not quantified. Rather, the strength of the adhesive joint is measured. A better term for describing these types of tests is “adhesive joint strength.”
The microtensile bond test was developed to overcome this problem by designing samples to concentrate stress to the bond layer of an assembly. Here, test failure always occurs at the adhesive level. Information about the strength inherent to bonding materials rather than the adherends is generated. The microtensile test is more cumbersome and more difficult to undertake than some of the conventional tests; however, the insights gained are thought to outweigh these issues . Because of increased difficulty in sample preparation and testing, conventional tests, such as shear bond strength, are still used and reported in the literature .
Microtensile tests use multiple samples from a single tooth-composite assembly. For example, if eight specimens are obtained from a single assembly, this data set is treated as a sample of size eight by many researchers. This approach may not be appropriate with respect to managing experimental variation and analysis, however. Consequently, conclusions from bond strength studies in which adequate sample size has not been used may not be supported by the data. Appropriate sample size management and increasing the number of experimental units (tooth-composite assemblies) provide better reliability of conclusions drawn from the vast literature on adhesives .
Adhesion promotion
The survival rate of no-preparation bonded cervical composite restorations in at least some short-term clinical trials has been shown to range from 45% to 100% . That restoration retention occurs at all by only applying solutions to tooth surfaces—and that it varies significantly—speaks to phenomena that occur at the interface between materials and tooth structure. These phenomena are largely based on surface reactions of those solutions with tooth structure. The elucidation of these surface reactions provides a good starting point for appreciating contemporary commercial bonding systems.
Whether bonding composites to tooth structure or paint to steel, two primary variables are to be considered in the formation of bonds between dissimilar materials. The first of these variables is the substrate being bonded to, and the second involves the nature of materials that interfacially interact with those surfaces. Their interactions and the properties of the materials used are the major determinants for the long-term success of the adhesive joints formed. In all cases, adhesion promotion follows a prescribed generic methodology, which involves (1) substrate preparation, (2) the application of functional molecules (primers) to the surface, and (3) the placement of application-specific overlayers that react with the primer.
Each step in the methodology has a specific function. Substrate optimization involves the dissolution of barrier layers that inhibit primer interaction with the intended bulk material (ie, the material to which bonds are to be formed). In some cases, these layers are measured in angstroms, as in the case of an oxide layer or multilayer. (As a point of reference, a sheet of paper is approximately 1 million Å thick.) In heterogeneous materials (eg, enamel and dentin), surface preparation also can involve the selective removal of substrate components to enable more efficient surface reactions, alter surface conformation, or change the surface energy .
Optimization of tooth surfaces involves the use of acids for the removal of smear debris (the barrier layer on tooth surfaces) and the dissolution of apatite crystals from enamel and dentin (the selective removal of substrate components) to develop microporous surfaces. Acid treatment also modifies the surface energy, which can be easily observed by placing a drop of water on either enamel or dentin before and after acid treatment. In the “before” case, the drop of water is observed to “bead up” and have a relatively large contact angle. After acid treatment, the water spreads on the surface and has a contact angle that is effectively as low as zero.
In general, primers are bi- or multifunctional molecules with chemical group terminations that react with the adherends. In dental applications, primer molecules have an “adhesive” group at one end to react with enamel or dentin and a polymerizable group at the other end to cross-link with resin overlayers, typically known as “bonding” or “adhesive” resins. Variations of phosphorous-containing functionalities have been the most common feature of adhesive groups, although other functionalities, such as carboxylic acids, have been shown to be useful. The adhesive and polymerizable ends of the primer are separated by a “spacer,” which determines many of the properties of the primer, including solubility and wetting .
The function of a primer when bonding to enamel is to wet the surface (one of the requirements for promoting strong adhesion) and penetrate microporosities. In dentin, in addition to wetting, primers diffuse through the surface of the demineralized region and entangle collagen fibers on polymerization. Primers also can react with functional groups inherent to collagen or with apatite inherent to enamel or at the front between unaltered and demineralized dentin.
Resin overlayers copolymerize with primers and with subsequently placed composite resins. They have extensive cross-linking capabilities and add considerable strength to the adhesive joint. They are typically composed of comonomers, each of which contributes specific beneficial properties to the formation of the adhesive joint. Resin overlayers also can bond mechanically to other materials, such as amalgam, when using amalgam-bonding techniques.
Adhesion promotion
The survival rate of no-preparation bonded cervical composite restorations in at least some short-term clinical trials has been shown to range from 45% to 100% . That restoration retention occurs at all by only applying solutions to tooth surfaces—and that it varies significantly—speaks to phenomena that occur at the interface between materials and tooth structure. These phenomena are largely based on surface reactions of those solutions with tooth structure. The elucidation of these surface reactions provides a good starting point for appreciating contemporary commercial bonding systems.
Whether bonding composites to tooth structure or paint to steel, two primary variables are to be considered in the formation of bonds between dissimilar materials. The first of these variables is the substrate being bonded to, and the second involves the nature of materials that interfacially interact with those surfaces. Their interactions and the properties of the materials used are the major determinants for the long-term success of the adhesive joints formed. In all cases, adhesion promotion follows a prescribed generic methodology, which involves (1) substrate preparation, (2) the application of functional molecules (primers) to the surface, and (3) the placement of application-specific overlayers that react with the primer.
Each step in the methodology has a specific function. Substrate optimization involves the dissolution of barrier layers that inhibit primer interaction with the intended bulk material (ie, the material to which bonds are to be formed). In some cases, these layers are measured in angstroms, as in the case of an oxide layer or multilayer. (As a point of reference, a sheet of paper is approximately 1 million Å thick.) In heterogeneous materials (eg, enamel and dentin), surface preparation also can involve the selective removal of substrate components to enable more efficient surface reactions, alter surface conformation, or change the surface energy .
Optimization of tooth surfaces involves the use of acids for the removal of smear debris (the barrier layer on tooth surfaces) and the dissolution of apatite crystals from enamel and dentin (the selective removal of substrate components) to develop microporous surfaces. Acid treatment also modifies the surface energy, which can be easily observed by placing a drop of water on either enamel or dentin before and after acid treatment. In the “before” case, the drop of water is observed to “bead up” and have a relatively large contact angle. After acid treatment, the water spreads on the surface and has a contact angle that is effectively as low as zero.
In general, primers are bi- or multifunctional molecules with chemical group terminations that react with the adherends. In dental applications, primer molecules have an “adhesive” group at one end to react with enamel or dentin and a polymerizable group at the other end to cross-link with resin overlayers, typically known as “bonding” or “adhesive” resins. Variations of phosphorous-containing functionalities have been the most common feature of adhesive groups, although other functionalities, such as carboxylic acids, have been shown to be useful. The adhesive and polymerizable ends of the primer are separated by a “spacer,” which determines many of the properties of the primer, including solubility and wetting .
The function of a primer when bonding to enamel is to wet the surface (one of the requirements for promoting strong adhesion) and penetrate microporosities. In dentin, in addition to wetting, primers diffuse through the surface of the demineralized region and entangle collagen fibers on polymerization. Primers also can react with functional groups inherent to collagen or with apatite inherent to enamel or at the front between unaltered and demineralized dentin.
Resin overlayers copolymerize with primers and with subsequently placed composite resins. They have extensive cross-linking capabilities and add considerable strength to the adhesive joint. They are typically composed of comonomers, each of which contributes specific beneficial properties to the formation of the adhesive joint. Resin overlayers also can bond mechanically to other materials, such as amalgam, when using amalgam-bonding techniques.
Nomenclature
Historically, bonding systems have been composed of components that were applied sequentially and followed the generic adhesion promotion steps previously outlined. To simplify bonding procedures and reduce the reporting of postoperative sensitivity (the latter being thought to result from incomplete permeation of primers in demineralized dentin), steps were combined. Adhesive systems based on various permutations of the application steps were developed, including adhesives that combined the generic steps one and two, or two and three, or even steps one, two, and three into a single solution.
In contemporary nomenclature, bonding systems are classified by whether an acidic solution is applied and rinsed or left in situ. These are respectively referred to as “etch-and-rinse” or “no-rinse” systems. The former is composed of either three- or two-step systems, in which the initial step always involves a neat acid application. The etch-and-rinse two-step system is sometimes referred to as a one-bottle system, despite the need for two steps. The one-bottle system is composed of a solution that contains primer and adhesive resin components.
No-rinse systems, generically referred to as self-etch bonding materials, can either involve two- or one-step applications. The former application demineralizes and primes tooth surfaces simultaneously. The adhesive layer is applied as a second step. One-step bonding materials demineralize, prime, and bond in a single application. With the exception of glass ionomer cements, whose carboxyl functionality complexes with calcium in apatite , all adhesive systems used by dental professionals follow the generic bonding methodology described. The systems differ from each other with respect to application steps and specific composition used for achieving each of the adhesion promotion objectives. These differences account for their differences in clinical performance.
Substrate structure
Although well characterized elsewhere, a brief discussion of tooth substrates is useful with respect to a more detailed discussion of surface reactions of bonding systems.
Enamel
Compositionally, enamel contains 96% inorganic matter by weight, the balance being water and organic material . The latter consists of proteins in the form of amelogenins and enamelins. The inorganic matter consists of hydroxyapatite, a crystalline form of calcium phosphate, although some carbonate is present. Collectively, they are referred to as biological apatite . Structurally, enamel is composed of a higher order structure that consists of “rods,” which are organized in a repetitive pattern referred to as a “head-and-tail” arrangement. Crystal organization within the rod is complex, with crystal orientation varying based on location .
Dentin
Dentin is considerably more complex and consists of solid and porous phases. The porous phase consists of numerous fluid-filled tubules emanating from the pulp. They traverse the dentin to the dentino-enamel junction, making dentin a highly permeable tissue. Each dentinal tubule is surrounded by a collar of hypermineralized, peritubular dentin . The organic matrix of peritubular dentin is delicate and is lost along the mineral phase after decalcification. The structure surrounding the peritubular dentin is intertubular dentin, which is approximately 9% less mineralized than peritubular dentin.
Intertubular dentin constitutes the bulk of the solid phase of dentin. The intertubular dentin is a biphasic biologic composite that contains mineral and organic components. As in enamel, the mineral phase consists of crystals of biologic apatite, although dentinal hydroxyapatite is plate-shaped and much smaller than the hydroxyapatite crystal in enamel . The organic phase of dentin primarily consists of type I collagen, which acts as a scaffold for mineralization, and minor amounts of noncollagenous proteins, which are implicated in mineralization . Collagen is generally acid insoluble , whereas noncollagenous proteins are soluble in the acids used for tooth demineralization (Ivan Stangel, DMD, unpublished data, 1996). The dentin matrix remains relatively intact after decalcification, whereas noncollagenous proteins are removed from their surfaces.
Surface reactions: acid effects
Adhesion promotion to mineralized tissue is based on the reaction of biologic apatite with acids. For water-soluble acids, the nature of the reaction and the degree of demineralization are complex and are based on pH and counterion. In dentin, the reaction kinetics are diffusion controlled . Although proton concentration can correlate directly to the degree of demineralization, the solubility of calcium salts (reaction products) at the demineralization front affects the degree of demineralization . For example, for a given pH, maleic acid produces a deeper demineralized region in dentin than does citric acid, partly because of the formation of insoluble salts at the demineralization front . For water-soluble salts, an increase in pH increases the degree of demineralization according to the general reaction in the following equation :
This reaction has implications for the integrity of adhesion to enamel for certain acidic primers with respect to proton concentration and the ability to sufficiently demineralize tooth surfaces. Milder self-etch adhesives have a lower proton concentration, which affects the quality of etch on enamel and dentin.
In essence, acid reaction with either enamel or dentin dissolves apatite crystals and creates a microporous surface morphology. In the case of enamel, the rate of dissolution depends on crystal orientation-inter-rod rather than rod peripheries are preferentially removed to produce the microporous surface. The chemical composition of this surface, when using water soluble acids, remains comparable to native enamel.
In the case of dentin, acid treatment removes the smear layer, exposes collagen, increases dentin permeability, and chemically modifies the surface . A microporous surface is also formed. However, here it is predominantly composed of a complex network of collagen fibrils largely absent of mineral crystals and containing significant amounts of water that has displaced apatite crystals. In addition to demineralizing effects, the application of phosphoric acid to dentin surfaces removes acid-soluble phosphoproteins that are implicated in mineralization of collagen surfaces .
Thus, the basis for most adhesion in modern dentistry involves the reaction of biological apatites with protons and the subsequent secondary reactions that occur with priming agents. The manner of applications of the agents and the nature of the materials designed to interact with the acid-treated surfaces are what distinguish the modern bonding systems.
Mechanisms of adhesion
The mechanisms of adhesion to the inorganic and organic components of teeth have relied primarily on the evaluation of morphologic relationships of materials with tooth substrates. As of this writing, a literature search on Pub Med using the key words [morphology dental bonding] returned 3300 “hits.” Although they make major contributions to understanding phenomena relating to adhesion, theories based only on microscopic examination of resin-tooth interfaces fail to consider potential chemical adhesion, inasmuch as the elucidation of molecular interactions cannot be determined by microscopy. Surface-sensitive techniques using various spectroscopies have been used to elucidate reactions between tooth structure and materials used for adhesive bonding. These techniques are described subsequently.
Enamel
Bonding to enamel can occur through either the well-known micromechanical retention of polymers on etched surfaces or chemical bonding to apatite. For the former approach, the gold standard for demineralizing enamel is highly concentrated (generally, pH<1.0) phosphoric acid having a concentration on the order of 35 weight percent. When coated with primers and subsequently placed resin overlayers, the ensuing micromechanical bond to phosphoric-acid etched enamel is substantial. The in vitro strength of adhesive joints formed by composite resins bonded to etched enamel has been reported to be on the order of 27 MPa .
Self-etching primers use an acidic primer to demineralize enamel surfaces. Primers can be made acidic by grafting on carboxylic acid or a phosphate ester. The pH of self-etching primers ranges from aggressive to mild (approximately 0.4–2.5). As would be expected, the etch patterns of the milder systems on uncut enamel are not as profound as those with a more aggressive etch . The latter system produces etch patterns consistent with total-etch systems.
Whether an aggressive or mild etch pattern, the microtensile bond strength of no-rinse, self-etching systems on uncut enamel tends to be less than that of a conventional etch-and-rinse system . On ground enamel, the bond strength for some self-etching systems improves but does not approach the strength and reliability of etch-and-rinse systems . Consistently, the highest bond strengths to enamel are obtained with an a priori etch and remain the standard against which all other systems are compared.
The use of a prior acid application step (to be rinsed off) increases bond strength to enamel for at least some no-rinse, self-etching adhesives and is recommended when restorations primarily rely on bonds to enamel formed to enamel . The application of phosphoric acid to dentin diminishes the bonding efficacy of self-etching adhesives to dentin, however . Effective bonding to noncarious sclerotic dentin in the cervical region of teeth also may require enhanced demineralization capability . The use of mild self-etching adhesives may preclude predictable bonding for no-preparation restorations.
In addition to micromechanical bonding, bonding to enamel apatite can occur through complexation with the calcium ion or reaction with the phosphate or the hydroxyl groups . Reactions along these lines occur with the treatment of enamel by polyalkenoic acids . The complexation of carboxyl groups derived from polyalkenoic acid with calcium is ionic in nature and may not be substantial for clinical use. Improvement in the glass ionomer bond to enamel can be made by pretreating surfaces with polyalkenoic acid . The effect of the acid, as with other acids, is to remove smear layer and partially demineralize the enamel surface. In addition to ionic bond formation to apatite, a micromechanical component to the bond is obtained .
Dentin
The hybridization theory originally advanced by Nakabayashi is the most commonly accepted basis for adhesion to dentin. Acid demineralization of superficial dentin exposes a collagen fibril network having inter- and intrafibrillar microporosities. Low-viscosity monomers placed on this surface diffuse into the demineralized region to form a resin-dentin interdiffusion zone. On polymerization, entanglement of the fibrils by the resin occurs to create a hybrid layer of resin-reinforced dentin. Formation of this hybrid layer is thought to be the primary bonding mechanism for many adhesive systems . That this is a commonly accepted mechanism may relate to the previously mentioned preponderance of studies that rely on microscopy and the morphologic evaluation of adhesive joints.
Mechanisms are more complex, however, and may involve primer solubility issues and chemical reactions with collagen, with residual apatite in dentin demineralized with mild acids, or with unaltered dentin at its interface with demineralized collagen. A secondary (possibly even a primary) bonding mechanism also may occur at the interface between demineralized and unaltered dentin. Examination of this interface demonstrates a relatively irregular surface that may inherently produce micromechanical bonds comparable to those formed to etched enamel.
The latter phenomenon can be observed in studies in which deproteination of collagen-rich demineralized dentin produces bonds that are substantial . This occurrence indicates that at least for some adhesive systems, the presence of a conventional hybrid layer is not necessarily a requirement for producing high bond strength. It further suggests that other mechanisms of bond formation to dentin are in effect . The basis for this observation needs further study.
The development of dental priming molecules has concentrated on the synthesis of phosphate derivatives of bifunctional mono- or dimethacrylate monomers. The adhesion mechanism of these structures may be based on ionic bonding of the phosphate group with one of the components of dentin. That the phosphate group may play a significant role in adhesion has been shown in a study that evaluated surface state effects on phosphate-mediated bonds to dentin . In that study, dipentaerythritol pentaacrylate monophosphate (DPP) and its analog, dipentaerythritol monohydroxy-pentaacrylate (DPO), having a hydroxyl rather than a phosphate termination, were respectively bonded to (1) smeared dentin, (2) polished dentin (dentin in which the smear layer had been mechanically removed), (3) demineralized dentin, and (4) demineralized/deproteinated dentin. The adhesive joint strength for DPP was significantly greater than that of DPO at each level. The shear strength (in MPa) of the adhesive joint for DPP ranged from 22.7 to 33.1, whereas the MPa range for DPO was 0.5 to 11.1 MPa. That DPO had some low level of bond formation to polished dentin suggests that the terminal OH group has some capability of bonding to hydroxyapatite. The strength to demineralized and deproteinated dentin suggests a contribution of micromechanical bond formation.
In addition to hybridization, chemical bonding to mineral and protein components of dentin has been described . Organic compounds that can bond to ions in mineral apatite found in dentin include (1) phosphate-based adhesives, (2) amino acid- or amino alcohol-based adhesives, and (3) adhesives based on dicarboxylates . Compounds that contain hydroxyl, carboxyl, amino, and amido groups can potentially react with bonding sites inherent to collagen .
Type I collagen comprises approximately 90% of the organic phase of dentin. Collagen is an ordered structure whose basic unit, the collagen molecule, is composed of a triple helix, with each of its strands being composed of approximately 20 amino acids, in which every third amino acid is glycine. Potential reactive sites in collagen primarily consist of carboxylate and hydroxyl groups. The latter can be found in sufficient concentration in hydroxyproline, threonine, and serine. The former groups are found in aspartate and glutamate. Reactions to either or both of these groups could form the basis of chemical bonding to the organic phase of dentin.
Using subtractive spectroscopic techniques and surface-sensitive photoacoustic Fourier transform infrared spectroscopy and Fourier transform-Raman spectroscopies, surface reactions between hydroxyethyl methacrylate, a common component of contemporary adhesive systems, and collagen have been elaborated . These reactions have been shown to involve transesterification, secondary amide formation, or possibly hydrogen bonding. It should be noted that several mechanisms of adhesion can operate simultaneously. Hybridization and chemical bond formation can work synergistically in forming bonds to dentin.
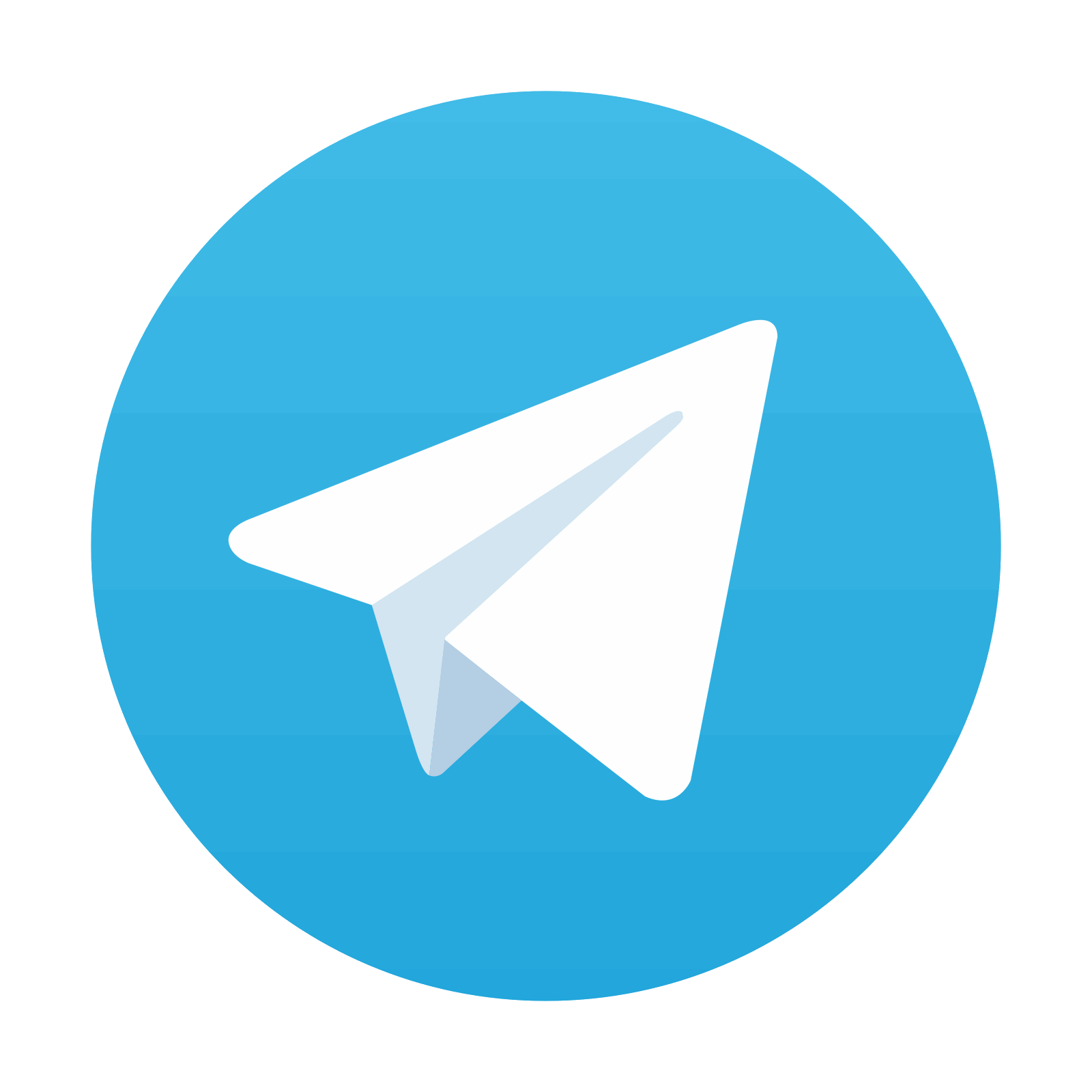
Stay updated, free dental videos. Join our Telegram channel

VIDEdental - Online dental courses
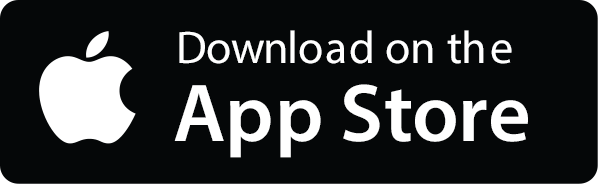
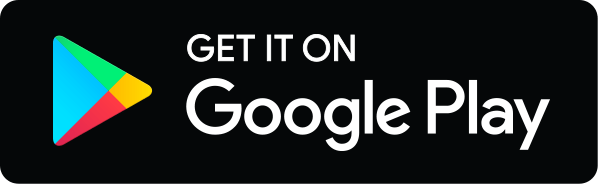