Abstract
Statement of problem
The effect of various scan bodies and scanning techniques on the accuracy and scan time for completely edentulous patients is not well understood.
Purpose
The purpose of this in vitro study was to evaluate the effects of 4 scanning techniques and 5 intraoral scan bodies (ISBs) on the trueness, precision, and scan time in a completely edentulous arch with 4 implants.
Material and methods
Five different ISB systems: AF, NT, DE, C3D, and ZI, and 4 different scanning techniques: unmodified master model (NO), glass fiduciary markers placed on the edentulous ridge (GB), pressure-indicating paste brushed over the ridge and palate (PP), and floss tied between the scan bodies (FL), were evaluated. Scan bodies were attached to an edentulous maxillary model with 4 dental implant analogs and scanned by using a structured blue light industrial scanner, and 5 consecutive digital scans of the model were made by using an intraoral scanner and 1 of the 4 techniques (n=5). The scans were superimposed on the master reference model, and the distance deviation and angular deviation of the scan bodies was calculated. The scan time was also recorded. A 2-factor ANOVA was used to examine the effect of scan body and technique on the trueness and scan time, with subsequent Tukey honestly significant difference or Bonferroni-corrected Student t tests. Precision was evaluated by tests for homogeneity of the variances between groups. Reliability for the entire study was evaluated using the intraclass correlation coefficient (α=.05 for all tests).
Results
The overall reliability of the study according to intraclass correlations was 0.999. In terms of trueness, no statistically significant interaction was found between the effects of scan body and technique on the distance deviation ( P =.246); however, the scan body ( P =.031) and technique ( P <.001) each had a significant effect independently. A statistically significant interaction was found between the effects of the scan body and technique on angular deviation ( P <.001). Testing for the homogeneity of variances demonstrated significant differences in the precision among the groups in terms of distance deviation ( P ≤.013) and angular deviation ( P ≤.003). No statistically significant interaction was found between the effects of the scan body and technique ( P =.076) on the scan time; however, the scan body alone was found to have a significant effect ( P <.001).
Conclusions
The accuracy (trueness and precision) of complete-arch digital implant scans using ISBs was affected by both the scan body and scan technique when using an intraoral scanning system. The ZI scan body had significantly less distance deviation, whereas splinting scan bodies with floss led to significantly more distance deviation. The scan techniques with different surface modifications were not found to improve the scan accuracy. The use of different ISBs led to significant differences in the scan time.
When performing complete-arch digital scans with 4 implants, clinicians should choose the intraoral scan body (ISB) and scan technique carefully, as the ISB and scan technique can significantly affect the accuracy of the scan when using the test scanner. Certain ISBs may be scanned faster than others.
The use of implant-supported prostheses in the replacement of natural missing teeth is a reliable and effective treatment option. Obtaining accurate dental impressions is a crucial step in any implant treatment. Inaccurate transfer of the implant position can lead to an ill-fitting prosthesis, which may put unnecessary strain on the various prosthetic components in the system and ultimately result in complications. Impression procedures for osseointegrated implants traditionally involve the use of impression or transfer copings. These are connected to the implant body at the time of the impression and captured in conjunction with the surrounding teeth and mucosa using impression trays and elastomeric impression materials such as polyvinyl siloxane or polyether. However, with the advent of computer-aided design and computer-aided manufacturing (CAD-CAM) technology, it is now possible to eliminate this step and use a completely digital workflow for the fabrication of implant-supported restorations.
The digital workflow can be either direct or indirect. The indirect workflow involves making a conventional implant impression, which is then digitized in the laboratory by using a benchtop extraoral optical scanner and laboratory scan bodies. The direct workflow includes the use of intraoral scan bodies (ISBs) and an intraoral scanning (IOS) device to generate a digital scan directly from the patient’s mouth. Both the extraoral optical scanner and IOS technology use a variety of principles to capture raw data in the form of point clouds, including confocal microscopy, triangulation, interferometry, wavefront sampling, structured light, laser, and video. A difference in technology may lead to differences in accuracy.
By definition, accuracy is a combination of precision and trueness. Precision describes how close repeated measurements of the same object are to each other, whereas trueness describes how far the measurement deviates from the actual dimensions of the measured object. Both are important considerations in the digital implant dentistry workflow and should be optimized by not only the scanning devices but also the scan bodies themselves.
The first digitally scannable implant components were introduced in 2004 and used an innovative coded healing abutment (Encode; Zimmer Biomet Dental), which provided 3D information on the implant location in relation to the adjacent teeth, opposing dentition, and surrounding soft tissues. In 2008, new types of digital technology were introduced and shown to be valid alternatives to traditional impression-making procedures. The first scannable impression copings were released shortly after and termed “scan bodies” by the manufacturer (Straumann Holding AG). The commercial ISB design has been highly variable, and their influence on accuracy is not completely understood.
In single-unit and short-span situations, digital implant scans using ISBs are shown to have similar accuracy as conventional impressions. In completely edentulous patients, however, decreased accuracy has been shown. Although the overall quality of the digitized data depends heavily on the specific IOS system, the surface topography and characteristics of the surface(s) to be scanned can affect the accuracy of digital implant impressions using ISBs. In attempting to scan an edentulous arch with ISBs, one specific challenge is the limited number of quality reference points between the scan bodies. When common reference points are limited, the images may not be stitched together properly or parts of the scan may be misinterpreted as redundant data. Thus, techniques have been proposed to overcome this limitation by increasing the number of reference data points in an edentulous arch by modifying the surface and topography of the edentulous ridge. However, the authors are unaware of a published study that has evaluated the effects of using these techniques in a completely edentulous arch.
The purpose of this in vitro study was to compare 4 different scanning techniques (conventional intraoral digital scan, a novel scan body splinting technique, and 2 mucosa surface modification techniques) and 5 different ISBs to evaluate their effects on the accuracy (trueness and precision) and scan time in a completely edentulous arch. The first null hypothesis was that different scanning techniques and scan bodies would not affect the trueness of the scans. The second null hypothesis was that different scanning techniques and scan bodies would not affect the precision of the scans. The third null hypothesis was that different scanning techniques and scan bodies would not affect the scan time.
Material and methods
Five different ISB systems were evaluated: AF (IO-Flo; Dentsply Sirona), NT (Nt-Trading GmbH & Co KG), DE (DESS-USA), C3D (Core3Dcentres), and ZI (Zimmer Biomet Dental). Four different scanning techniques were evaluated: unmodified master model (NO), glass fiduciary markers placed on the edentulous ridge (GB), pressure-indicating paste (PIP) brushed over the ridge and palate (PP), and floss tied between the scan bodies (FL) ( Figs. 1 , 2 ).


Five identical polyurethane edentulous maxillary models with a soft tissue replica were fabricated with 4 parallel dental implant analogs (TSV 4.1; Zimmer Biomet Dental) in the first molar and canine positions placed 3 mm below the model’s surface. The distance between the 2 middle implants was approximately 20 mm and approximately 14 mm from the middle implant to the posterior implant. Four identical ISBs from 5 different manufacturers were attached to the implants, giving a total of 5 master models. The scan bodies were inserted and tightened into place following the manufacturer recommended guidelines.
Each reference model was scanned, and its surface was reverse engineered by using an ISO 17025 calibrated, structured blue light industrial scanner (COMET L3D; Carl Zeiss Optotechnik GmbH). The digitized model was saved as a standard tessellation language file to serve as the master reference model (MRM). Owing to the nature of the scanner technology, an antireflective powder spray with an approximate thickness of 2 μm was applied to the models before scanning. The scanner’s uncertainty was reported to be less than 11 μm ( www.3d-engineering.net ).
Five consecutive digital scans (n=5) of the master model were made by using an intraoral scanner (TRIOS; 3Shape) and each of the 4 techniques in a temperature- and humidity-controlled environment. The groups were scanned in a random order generated by a spreadsheet (Excel; Microsoft Corp). A standardized scan path was used according to the manufacturer’s recommendation, which consisted of scanning the occlusal surface, then the buccal surface, and then the palatal surface. All scans were timed from start to finish, and a scan was considered complete once the scan body surfaces were captured entirely and no major holes in the reference model were present.
All test scans were then superimposed over the MRM using the best-fit algorithm of an industrial metrology software program (Polyworks; InnovMetric Software Inc), which excluded the scan bodies. As a result, only unchanged features of the standard tessellation language files, such as the palate, ridges, and model base, were used in the superimposition to minimize any alignment errors or averaging of data in the measured portion of the scan bodies.
A coordinate system was created and used throughout the entire inspection to measure the 3D distance deviation and angular deviation of the scan bodies. For the positional deviations, a cross section was created 2.25 mm from the top of each scan body, and their center point was located and compared with the corresponding point on the MRM, giving positional changes in the X, Y, and Z directions. The scan bodies used for each measurement were labeled 1 through 4, and the same labels were used for every inspection ( Fig. 3 ). The distance deviation was calculated by entering the raw data into the distance formula to generate the 3D distance deviation for each scan body and then averaged among the 4 scan bodies on the model. To determine the angular deviation, cylinders were fitted to each scan body by using the same computer software program, and a central axis was generated for each. The nominal axis from the MRM was considered to be at an angle of zero, and the resultant 3D angle between the MRM and test model was recorded and then averaged to generate the angular deviation among the 4 scan bodies.

A 2-factor ANOVA was conducted that examined the effect of the scan body and technique on distance deviation, angular deviation, and scan time. For each ANOVA, the main effects were scan body and technique, and the analyses included their interactions. A Tukey test was used to more completely resolve the statistically significant effects on distance deviation and time, whereas a Bonferroni-corrected t test was used for angular deviation (α=.05 for all tests).
Precision was defined as the degree of variance within groups of scans, whereas trueness was defined as the amount of average distance and average angular deviation between the corresponding scan bodies in the test scan and the MRM. In terms of precision, 4 tests (Levene, O’Brien, Brown and Forsythe, and Bartlett) were used to evaluate the homogeneity of the variances among the scan body and technique subgroups. The overall reliability of the study method was evaluated by using intraclass correlation according to Shrout and Fleiss.
Results
The total number of measurements in the raw data set was 1152, and 48 measurements were missing because of postprocessing errors in the scanner software. In these situations, an average was taken of the 3 remaining scan bodies to limit the biasing influence. Intraclass correlation for reliability for the raw data set was found to be 0.999.
The raw data for distance deviation, angular deviation, and scan time are shown in Figures 4-6 , and the results of the ANOVAs are shown in Table 1 . According to the Tukey adjustment, ZI showed significantly less distance deviation than AF ( P =.041), and FL showed significantly more distance deviation than GB ( P =.008), PP ( P =.013), and NO ( P =.002) ( Figs. 7 , 8 ). A significant difference in precision with regard to distance deviation was noted by the tests for homogeneity of the variances ( P ≤.013); however, subsequent pairwise comparisons could not further resolve these differences.



Property | Effect | Dfnum | Dfden | F-ratio | P |
---|---|---|---|---|---|
Distance deviation (μm) | Scan body | 4 | 80 | 2.815 | .031 |
Technique | 3 | 80 | 5.790 | .001 | |
Scan body×technique | 12 | 80 | 1.282 | .246 | |
Angular deviation (degrees) | Scan body | 4 | 80 | 17.827 | <.001 |
Technique | 3 | 80 | 0.855 | .468 | |
Scan body×technique | 12 | 80 | 4.308 | <.001 | |
Scan time (min) | Scan body | 4 | 80 | 7.933 | <.001 |
Technique | 3 | 80 | 2.210 | .093 | |
Scan body×technique | 12 | 80 | 1.728 | .076 |
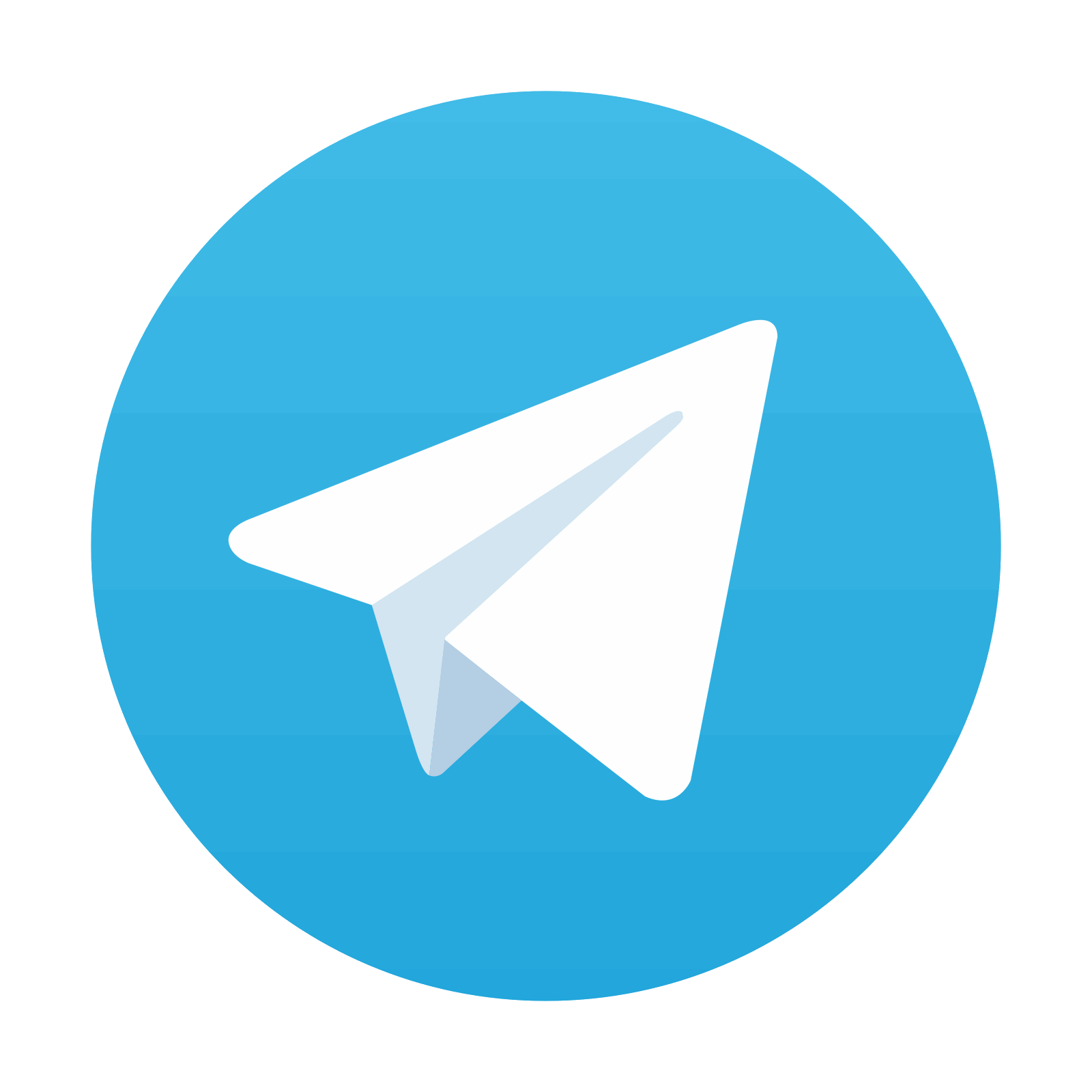
Stay updated, free dental videos. Join our Telegram channel

VIDEdental - Online dental courses
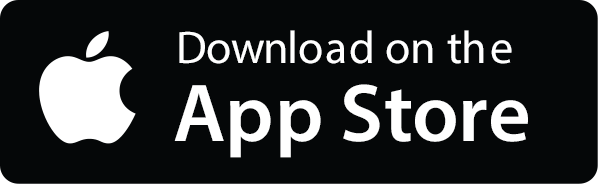
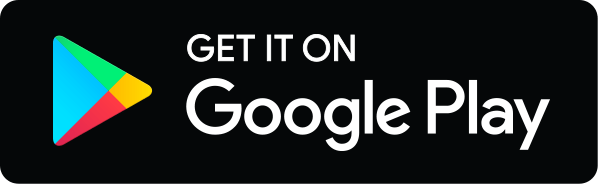
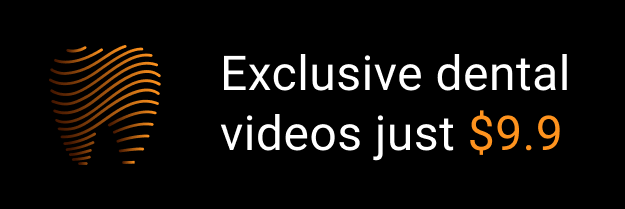