Introduction
Our objective was to evaluate the effect of cusp height of posterior teeth (first premolar, second premolar, first molar) on the accuracy of the computer-aided design and computer-aided manufacturing (CAD-CAM) indirect bonding system.
Material
Five kinds of maxillary arch models, without attrition, were divided into 2 groups: control group (with 0.5 mm of grinding) and experimental group (with the addition of 0.5 mm of wax to the cusp tip). Rapid prototype models were printed for both groups. Transfer jigs of the individual tooth brackets were designed using a digital model. 3-dimensional program to evaluate the differences between the intended digital bracket position and actual bracket position after indirect bonding. The differences were measured in the linear (mesiodistal, buccolingual, vertical) and angular (angulation, rotation, torque) dimensions. The Wilcoxon signed rank test was used for statistical analyses; significance was defined as P <0.05.
Results
Both groups had similar frequencies of errors between the intended and actual bracket positions. The frequencies of vertical errors over 0.5 mm were 3.3% and 6.7% in the control and experimental groups, respectively. The frequencies of angulation, rotation, and torque errors over 1° were 53.3%, 43.3%, and 60%, respectively, for the control group; and 60%, 60%, and 73.3%, respectively, for the experimental group.
Conclusions
A difference in cusp height of maxillary posterior teeth did not produce a statistically significant difference in the linear and angular dimensions of bracket placement with the CAD/CAM indirect bonding system. However, given the tendency for a higher frequency in bracket placement errors in posterior teeth with larger cusp tips, cusp height should be considered when using a CAD/CAM indirect bonding system.
Highlights
- •
Accuracy of bracket placement in CAD/CAM indirect bonding system was studied.
- •
Differences in cusp height of maxillary posterior teeth did not affect accuracy.
- •
Bracket placement errors were more frequent in posterior teeth with larger cusp tips.
There are 2 methods for bracket positioning: direct bonding and indirect bonding. Many orthodontists use the indirect bonding system for accurate bracket positioning. It was introduced by Silverman et al, developed for the fabrication of a transfer core made of compressed plastic fitted over a bracket, and fixed by cement on a model. The indirect bonding system has many advantages: it makes the patients comfortable, facilitates easy reproduction of the bracket, provides easy overcorrection, allows for the control of in-out movements of the teeth, reduces the staff required for the treatment, and reduces treatment duration.
The indirect bonding system was developed in a laboratory process. It was reported that a silicon-based tray provided better precision for bracket positioning compared with a vacuum-based tray.
Recently, computer-aided design and computer-aided manufacturing (CAD/CAM) systems have been used for indirect bonding. This process involves designing a virtual model in a CAD/CAM program to produce a transfer jig. The CAD/CAM transfer jig facilitates bonding of the bracket to the target tooth. For instance, 3-dimensional (3D) modeling of the maxilla and the mandible has been used for the ideal setup and the rapid production of prototype transfer jigs. These would, subsequently, be involved in the transfer of brackets with individualized custom resin bases. Although satisfactory results of treatment have been reported, nonetheless, the literature indicates differences in treatment outcomes on teeth set in wax, after the use of either a CAD/CAM indirect bonding system or a traditional, indirect bonding system; this demonstrated a difference in bracket position between the techniques.
Accurate bracket positioning has an important influence on the result of orthodontic treatment. Factors affecting ideal bracket placement include the following: tooth shape and malformation, material of the transfer tray, bonding agent, technique sensitivity, environment of the clinic, and patient management. An understanding of these factors would enable ideal positioning of the bracket.
The average vertical heights (measured from the marginal ridge to the cusp tip) were 1.7 ± 0.5 mm for the maxillary first premolar, 1.24 ± 0.45 mm for the maxillary second premolar, and 0.83 ± 0.4 mm for the maxillary first molar. These height measurements could be used as references for positioning the bracket. Tooth morphology influences the bracket position for first- and third-order corrections. Additionally, the degree of attrition is classified on the basis of the occlusal attrition of the teeth and can be categorized accordingly: no attrition reported, evidence of a different shape without the loss of the crown length, 1 mm or less loss of crown length, and loss of over 1 mm of the crown length. The cusp heights of the posterior teeth differ by the degrees of attrition; however, the ideal placement of the bracket, based on cusp height, has not been evaluated. Therefore, we aimed to determine the influence of the height of posterior cusps on the accuracy of bracket placement in a CAD/CAM indirect bonding system. Superimposition of 3D images was used in this evaluation.
Material and methods
Five maxillary plaster models were chosen; they had no evidence of attrition, lacked a distinctive cusp tip, and were characterized by mild crowding (<3 mm). The experimental plaster models also had no evidence of the following traits: tooth malformation, congenitally missing teeth, and sink depth and protrusion in the buccal surface. The chosen models were modified accordingly into the control models and the experimental models. The right and left posterior teeth (first premolars, second premolars, first molars) of each model were evaluated. The control and experimental groups each had a sample of 30 teeth, representing common teeth with normal attrition and teeth with maximum cusp height, respectively. Metal brackets with an 0.018-in slot (Mini Diamond Twin; Ormco, Orange, Calif) and buccal tubes (Ormco) were bonded on the teeth.
The cusp tips in the control group were reduced to 0.5 mm to normalize the occlusal surface of natural teeth, and 0.5 mm of wax was added to the cusp tips in the experimental group. There was a 1.0-mm difference in the cusp heights between the 2 groups. Subsequently, the 2 groups of plaster models were scanned using a 3D scanner (7 series; Dental Wings, Montreal, Quebec, Canada); 3D image files of each group were produced. The connecting parts of the virtual cusp tips, formed by the addition of wax, were smoothed with a software program (Dental Wings). Rapid prototype models of the control and experimental groups were fabricated using a 3D printer (EQ-1; CMET, Kanagawa, Japan) ( Fig 1 ).

After fabrication of the rapid prototype models, the 3D images were segmented into individual teeth in a software program (3Txer; Orapix, Seoul, Korea). First, an appropriate arch formed by a treatment plan was chosen in this program. Subsequently, the mild crowding was resolved, and the teeth were aligned automatically in an arch formation. The position of each tooth was then controlled in the following directions: occlusal, buccal, and lingual. Buccal appliances were chosen, and individual bracket positions were controlled according to the positions of each tooth. After this setup, the shape of the transfer jig of each tooth was selected, allowing for the transfer of the bracket to the teeth in the oral cavity. Customized transfer jigs were printed by a 3D printer (Projet HD 3000 Plus; 3D Systems, Rock Hill, SC) after modification of their shape and size according to the shapes of the respective teeth. The insertion of the selected bracket into the jig was followed up by the addition of resin to the mesh of the bracket. Thus, the customized resin base of each tooth of the rapid prototype maxillary model was fabricated by handwork; the manufacture of the final bracket and jig was completed in a laboratory.
Individual brackets were bonded to the teeth of the control and experimental groupz via the fabrication of individual jigs. The 3D scanner (Dental Wings) used in the fabrication of the plaster models provided the 3D images after indirect bonding of the brackets ( Fig 2 ).

Then, we superimposed the intended bracket position and the actual bracket position after indirect bonding and measurement of the differences. The Geomagic Verify program (3D Systems) was used to superimpose the 3D images of best fit onto the occlusal, buccal, and mesiodistal views of each tooth. This was done to evaluate the differences between the intended position of the bracket and the actual position of the bracket to the tooth after indirect bonding ( Fig 3 ). Using the Meshmixer program (Autodesk, San Rafael, Calif), virtual rectangular wires were produced and inserted into the bracket of the initial digital model, and that of the scanned virtual model after indirect bonding. The differences in the wire positions were subsequently compared ( Fig 4 ). Other 3D programs, such as Geomagic Design X and Cimatron (3D Systems), were used to measure the differences between the intended and actual positions of the bracket ( Fig 5 ). The difference between the intended bracket position and the actual bonded bracket was measured. Bracket placement error was measured using the linear distance of the mesiodistal, buccolingual, and vertical views. Placement errors in angulation, rotation, and torque were measured.



Statistical analysis
The mean values and the deviations of the linear distance of the mesiodistal, buccolingual, and vertical errors, in addition to the measurements for angulation, rotation, and torque, were compared using the Wilcoxon signed rank test. Statistical significance was defined as P <0.05.
The frequencies of errors exceeding the clinical limit in the control and experimental groups were compared to measure the prevalence of bracket placement errors. The measurement error for the clinical limit was set at 0.5 mm in the mesiodistal, buccolingual, and vertical dimensions. The clinical limit for angulation, rotation, and torque errors wz set at 1°. The SPSS software program (version 23.0; IBM, Armonk, NY) was used for the statistical analyses.
Five models were randomly selected for a repeat experiment: 3 models in the control group and 2 models in the experimental group. The Wilcoxon signed rank test was performed to compare the frequency of errors in bracket positions in the control and experimental groups. Linear distance errors in the mesiodistal, buccolingual, and vertical dimensions, and angular errors of angulation, rotation, and torque showed no significant differences between the original experiment and the repeated experiment ( P >0.05). Therefore, the occurrence of bonding errors in the indirect bonding system could be excluded in the comparison of the bracket placement errors between the control and experimental groups.
Results
In the comparison of mean errors in bracket positions according to cusp height of posterior teeth, mean mesiodistal errors were 0.09 ± 0.09 mm in the control group and 0.05 ± 0.01 mm in the experimental group for first premolars, second premolars, and first molars. Buccolingual errors were measured at 0.08 ± 0.05 mm and 0.11 ± 0.07 mm, and vertical errors were 0.14 ± 0.14 mm and 0.19 ± 0.2 mm in the control and experimental groups, respectively. Mean angulation errors were 1.53° ± 1.57° and 1.52° ± 1.37, and mean rotation errors were 1.1° ± 1.19° and 1.46° ± 1.26° in the control and experimental groups, respectively. Mean torque showed errors of 2.65° ± 2.91° and 3.36° ± 3.15° in each group. However, mean linear and angular errors in the control and experimental groups of maxillary posterior teeth were not statistically significant ( P >0.05). Mean buccolingual and vertical errors in the experimental group tended to be higher than those in the control group. Vertical errors were the highest in both groups. Mean rotation and torque errors were higher in the experimental group than those in the control group. In addition, torque errors were the highest in both groups, angulation errors were second, and rotation errors were next, in descending order ( Table I ).
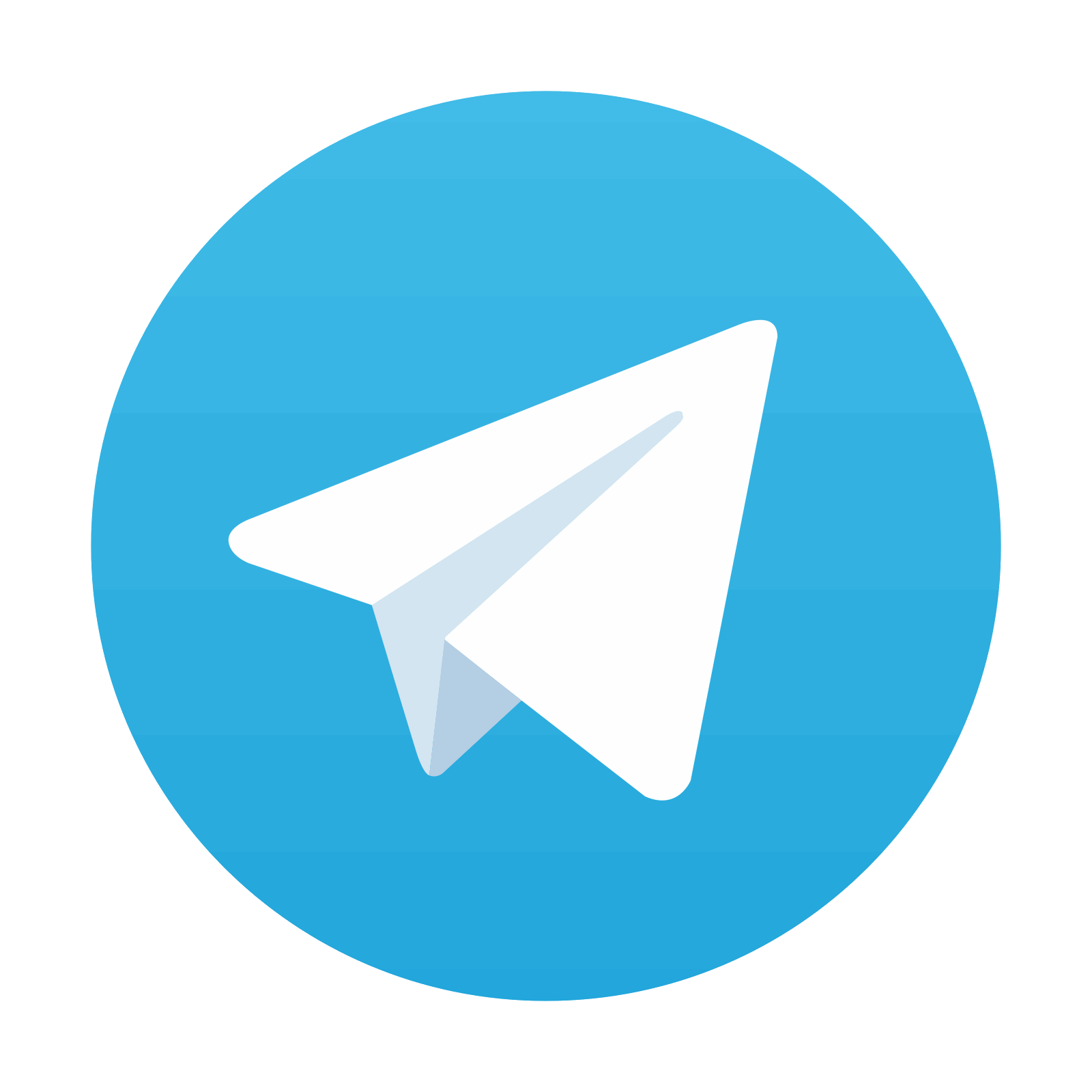
Stay updated, free dental videos. Join our Telegram channel

VIDEdental - Online dental courses
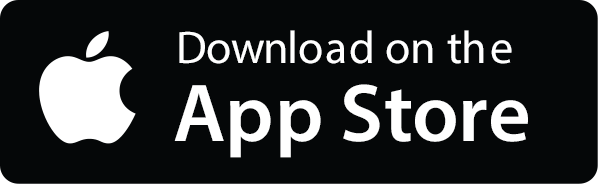
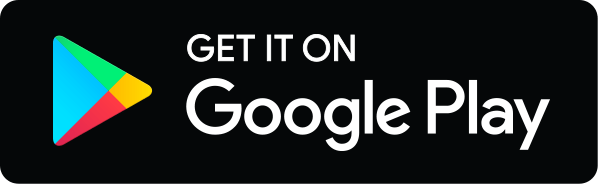
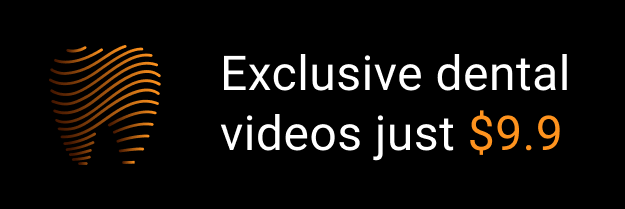