Introduction
A rapidly advancing digital technology in orthodontics is 3-dimensional (3D) modeling and printing, prompting a transition from a more traditional clinical workflow toward an almost exclusively digital format. There is limited literature on the accuracy of the 3D printed dental models. The aim of this study was to assess the accuracy of 2 types of 3D printing techniques.
Methods
Digital and alginate impressions of the oral environment were collected from 30 patients. Subsequently, digital impressions were used to print 3D models using digital light processing (DLP) and polyjet printing techniques, and alginate impressions were poured up in stone. Measurements for the 3 model types (digital, DLP, and polyjet) were compared with the stone models. Tooth measurements (first molar to first molar) included mesiodistal (crown width) and incisal/occlusal-gingival (crown height). Arch measurements included arch depth and intercanine and intermolar widths. Intraobserver reliability of the repeated measurement error was assessed using intraclass correlation coefficients.
Results
The intraclass correlation coefficients were high for all recorded measurements, indicating that all measurements on all model types were highly reproducible. There were high degrees of agreement between all sets of models and all measurements, with the exception of the crown height measurements between the stone and DLP models, where the mean difference was statistically significant.
Conclusions
Both the DLP and polyjet printers produced clinically acceptable models and should be considered viable options for clinical application.
Highlights
- •
Alginate and intraoral digital impressions of 30 patients were taken.
- •
Linear tooth and arch measurements were evaluated for accuracy.
- •
Digital light precessing (DLP) and polyjet printing produced 3D models that were clinically acceptable.
- •
DLP and polyjet printers are viable options for clinical applications.
- •
DLP and polyjet printers may serve as a replacement for stone models.
The evolution of digital technologies in orthodontics has influenced the traditional clinical workflow toward an almost exclusively digital format. One of the most rapidly advancing digital technologies is 3-dimensional (3D) modeling that uses scanned data or digital impressions to create digital models. These digital impressions can be acquired either indirectly from a patient’s models or impressions using a desktop or intraoral scanner, or directly from the patient’s oral cavity using an intraoral scanner.
The authors of most previous scanner studies have evaluated the accuracy of digital models produced from indirectly acquired digital impressions. However, the current digital workflow in orthodontics predominantly relies on digital impressions directly acquired from the oral environment with an intraoral scanner. This inconsistency between research and practice demonstrates a need to test the accuracy of the latter, as has been done for the former.
The advantages of digital models include no physical storage requirement; instant accessibility; ability to do digital diagnostic or treatment simulations; positive patient perceptions; ability to immediately send to an outside laboratory; no risk of breakage, wear, degradation, or loss; and an overall improved continuity of care; in addition, digital models are less labor intensive. Although the advantages are numerous, digital models come with at least 1 important disadvantage. Without a physical model, treatment planning for complex cases in a teaching environment can be challenging, and a physical model is still required for appliance fabrication.
Rapid prototyping provides a solution to this limitation by producing a physical replica of a digital model. In orthodontics, the most common rapid prototyping technique is 3D printing. Two of the most common 3D techniques in the marketplace today are digital light processing (DLP) printing and polyjet printing. Both techniques use additive manufacturing to fabricate a 3D model, layer by layer, based on a digital model. DLP printing uses a conventional light source such as an arc lamp, liquid crystal display panel, or projection source to cure the surface layer of a vat of photo-polymerizing resin in a specific orientation based on a digital model. Polyjet printing uses jet heads that spray or jet the resin in the desired areas. As the jet heads make subsequent passes, each sprayed layer is cured using an ultraviolet light source. In both printing techniques, the initial layer of resin is cured onto a build platform or a build plate, with each subsequent layer cured directly to the previous layer of cured resin in the z-axis to create a 3D object. The build plate lowers at a predetermined increment, which determines the thickness of each layer of resin that is cured. The process of producing a 3D printed model from a digital impression is summarized in Figure 1 .

There is limited literature on the accuracy of 3D printed dental models. Most previous studies have concluded that 3D printed models are reasonably accurate and may be suitable for clinical use. However, these studies have had limited sample sizes, did not measure arch dimensions, and used digital impressions that were not recorded directly from the patient. The authors of a recent study investigated the accuracy of 3D printed models produced from digital models acquired from the oral environment and measured multiple arch dimensions. However, they used a limited sample size and did not compare the printed models with stone models, which have been established as the gold standard in previous studies.
The accuracy of a 3D printer must be confirmed through research for it to be mainstreamed into the orthodontic specialty. Therefore, this study was designed to assess the accuracy of 3D digital models and printed models in the context of a commonly used digital workflow in orthodontic practice.
Material and methods
Ethical approval for this study was obtained from the Institutional Review Board for the Protection of Human Subjects at the University of Oklahoma (Institutional Review Board number 7132; reference number 655134) in Oklahoma City.
A power analysis was done based on a similar precursor study. A total of 22 patients were required for this study to oftain an 80% probability of generating 95% confidence intervals with margins of error less than 0.1 mm for tooth measurements and 0.25 mm for arch dimension measurements.
Thirty patients were randomly selected from a pool of retention patients who received treatment at the University of Oklahoma Graduate Orthodontic Clinic. A patient was considered in retention if the appliances had been removed 6 to 18 months before records collection for this study. The inclusion criteria were (1) orthodontic treatment at the Graduate Orthodontic Clinic, (2) complete comprehensive treatment, and (3) all permanent teeth, first molar to first molar, in both dental arches.
Both the digital and alginate impressions were taken at the 1-year retention appointment for all patients. Digital impressions were recorded using a chairside intraoral scanner (Omnicam; Dentsply Sirona, York, Pa). Jeltrate fast-set alginate (Sirona Dentsply) was used to make impressions with stock impression trays. The alginate was mixed using Cadco Alginator II electric mixer (Patterson Dental, St Paul, Minn). Impressions were poured with white ISO type 3 orthodontic model stone (Whip Mix, Louisville, Ky) and mixed using a Vac-U-Mixer (Whip Mix). All aspects of record collection followed the manufacturers’ recommendations. Alginate impressions were poured with dental stone within 24 hours.
All digital models generated with the intraoral scanner were converted to .STL file format using additional 3D imaging software (Dolphin Imaging and Management Solutions, Chatsworth, Calif). The .STL files were cleaned and prepared for 3D printing using Netfabb additive manufacturing and design software (Autodesk, San Rafael, Calif). The prepared .STL file for each patient was printed using two 3D printing techniques: DLP (Juell 3D Flash OC; Park Dental Research, New York, NY) and polyjet (Objet Eden 260VS; Statasys, Eden Prairie, Minn). Models were printed successively from their respective printers. The workflow for collecting patient records and producing study models is summarized in Figure 2 . All 3D printed models were printed as solid models with a horseshoe-shaped base and in the horizontal position. Figure 3 illustrates both 3D printed model types.


A total of 240 maxillary and mandibular arches were measured with 60 arches from each of the 4 model types (stone, digital, DLP, and polyjet). All measurements of the physical models were obtained using a calibrated digital caliper (Orthopli, Philadelphia, Pa) and measured to the nearest 0.01 mm directly from the models, with the exception of arch depth, which was measured using the digital caliper from a 1:1 scale photocopy of the occlusal view. Measurements of the digital models were all obtained using Dolphin Imaging software. For all teeth, first molar to first molar, in both arches, the tooth measurements included (1) the mesiodistal widths from point contact to point contact (crown width) and (2) the incisal/occlusal-gingival heights from gingival zenith to cusp tip or incisal edge (crown height). For both arches, arch dimension measurements included (1) intercanine width from cusp tip to cusp tip, (2) intermolar width from mesiolingual cusp tip to mesiolingual cusp tip, and (3) arch depth from the midline of the central incisors to a perpendicular line crossing the mesial contact of the first molars. Crown height measurements for the digital models were not possible because of the inability of the software to replicate the same measurement technique used on the physical models. All other digital measurements were collected using the same measurement technique as on the physical models. Three calibrated researchers measured and recorded the data, with 1 researcher measuring either crown width, crown height, or arch dimensions for all models.
Statistical analysis
Bland-Altman plots were used to evaluate the agreement of the crown width, crown height, intercanine, intermolar, and arch depth measurements between the stone, digital, DLP, and polyjet models. Table I lists the comparisons and what each evaluated. Ten percent of each model type was measured a second time with a 2-week interval between measurements. Intraclass correlation coefficients (ICC) were used to assess the intraobserver reliability of the repeated measurement errors.
Comparison | Evaluation |
---|---|
Stone and digital | Clinical accuracy of digital impression |
Digital and DLP | Accuracy of the DLP printing process |
Digital and polyjet | Accuracy of the polyjet printing process |
Stone and DLP | Clinical accuracy of DLP printer |
Stone and polyjet | Clinical accuracy of polyjet printer |
Results
A total of 5760 tooth measurements were recorded from 240 maxillary and mandibular arches. An additional 720 arch dimension measurements were recorded from the same sets of arches, for a total of 6480 measurements. The ICC values were high for all recorded measurements based on repeated measurement error, indicating that all measurements on all model types were highly reproducible ( Table II ).
Model | Crown width | Crown height | Intercanine width | Intermolar width | Arch depth |
---|---|---|---|---|---|
Stone | 0.03 | 0.04 | 0.07 | 0.08 | 0.06 |
Digital | 0.04 | NA ∗ | 0.07 | 0.08 | 0.04 |
DLP | 0.03 | 0.05 | 0.07 | 0.07 | 0.06 |
Polyjet | 0.02 | 0.07 | 0.08 | 0.08 | 0.06 |
∗ NA indicates that repeated measurement mean errors were not calculated due to the inability to replicate the same measuring technique digitally as was done physically with a digital caliper.
The Bland-Altman plots demonstrated high agreement between (1) stone and digital models for crown width, intercanine, intermolar, and arch depth measurements; (2) stone and polyjet models for all measurements; and (3) digital and polyjet models for crown width, intercanine, intermolar, and arch depth measurements ( Table III ). The limits of agreement are given in Table III . In addition, the Bland-Altman plots showed high agreement between stone and DLP models and between digital and DLP models for crown width, intercanine, intermolar, and arch depth measurements. However, the mean difference was statistically significant for crown height measurements between stone and DLP models. The mean crown height measurements of the DLP models were significantly lower than the mean crown height measurements for stone models by 0.29 mm, suggesting that the DLP printed models underestimated the true crown height dimensions.
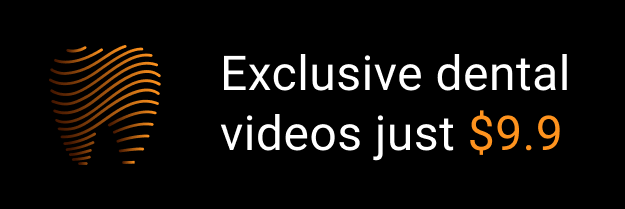